Highlights
- •
Resin-matrix ceramics systems demonstrated high reliability at functional loads.
- •
Resin-matrix ceramic fracture, chipping or catastrophic, is the chief failure mode.
- •
Cracks originated by quasiplastic deformation under compression loading.
Abstract
Objective
To evaluate the fatigue survival, failure mode, and maximum principal stress (MP Stress) and strain (MP Strain) of resin-matrix ceramic systems used for implant-supported crowns.
Methods
Identical molar crowns were milled using four resin-matrix ceramics (n = 21/material): (i) Shofu Hard, (ii) Cerasmart (iii) Enamic, and (iv) Shofu HC. Crowns were cemented on the abutments, and the assembly underwent step-stress accelerated-life testing. Use level probability Weibull curves at 300 N were plotted and the reliability at 300, 500 and 800 N was calculated for a mission of 50,000 cycles. Fractographic analysis was performed using stereomicroscope and scanning electron microscope. MP Stress and MP Strain were determined by finite element analysis.
Results
While fatigue dictated failures for Cerasmart (β > 1), material strength controlled Shofu Hard, Enamic, and Shofu HC failures (β < 1). Shofu HC presented lower reliability at 300 N (79%) and 500 N (59%) than other systems (>90%), statistically different at 500 N. Enamic (57%) exhibited a significant reduction in the probability of survival at 800 N, significantly lower than Shofu Hard and Cerasmart; however, higher than Shofu HC (12%). Shofu Hard and Cerasmart (>93%) demonstrated no significant difference for any calculated mission (300–800 N). Failure mode predominantly involved resin-matrix ceramic fracture originated from occlusal cracks, corroborating with the MP Stress and Strain location, propagating through the proximal and cervical margins.
Significance
All resin-matrix ceramics crowns demonstrated high probability of survival in a physiological molar load, whereas Shofu Hard and Cerasmart outperformed Enamic and Shofu HC at higher loads. Material fracture comprised the main failure mode.
1
Introduction
All-ceramic alternatives for implant-supported crowns indicate an increasing use of porcelain-fused to zirconia (PFZ), as a substitute for metal ceramics, accounting for approximately 90% survival rates after 5 years in function [ ]. Although such survival rates are high in the medium-term, a veneering ceramic chipping frequency of 24.5% for PFZ when compared to 9.5% for metal ceramics after 2.1 years has been observed [ ]. Improvements in the mechanical performance have been reported in a study that found a chipping rate of 4% for PFZ and no failures for metal ceramics [ ]. Veneering porcelain fracture in PFZ has been strongly associated with lower porcelain fracture toughness [ ], deleterious residual stresses during the cooling phase of veneer sintering [ ], among other causes. Also, when PFZ reconstructions are implant-supported, they may be more prone to fracture due to the lack of resiliency given by the periodontal ligament and its dampening effect upon occlusal forces [ , ]. Whereas results for tooth-supported reconstructions seem to be more encouraging, those for implant-supported crowns are still challenging and PFZ use has been suggested as a risk factor for failure, especially in the presence of an opposing ceramic reconstruction [ , ]. To overcome the aforementioned limitations, the development of monolithic blocks for computer-aided design/ computer-aided manufacturing (CAD/CAM) has become a fast-growing research field in the restorative arena [ ].
New polymeric restorative systems highly filled with ceramics are available through discs and/or blocks for milling and incorporate innovations in materials composition and microstructure [ ]. The so-called resin-matrix ceramic systems are usually comprised of methacrylate-based polymer matrices containing predominantly inorganic compounds that might include glasses, porcelains, glass-ceramics, and ceramics, and have been coded as ceramics by the American Dental Association (ADA) due to their high ceramic content [ , ]. Despite the ADA designation, materials science is very clear in defining such composites as polymer systems [ , ]. Advantages of these materials include a homogeneous, dense, and reliable microstructure due to block/disc fabrication under an industrial environment in high temperature and pressure [ , ], as well as milling damage tolerance that may be linked to an excellent machinability and small marginal gap, no need for post-milling firing, easy adjustment and polishing for optimal occlusion, and repairability [ , ]. The mechanical properties of different resin-matrix ceramic systems are dictated by their composition and microstructure, presenting a range of 150−270 MPa in flexural strength and 10−30 GPa in elastic modulus [ , , , ].
The improved biomechanical behavior of resin-matrix ceramics for implant-supported reconstructions is determined by their polymeric content and lower elastic modulus relative to ceramics, likely providing favorable resilience and improving occlusal forces dampening and damage tolerance [ ]. In the implant-supported scenario, reconstructions with resin-matrix ceramics have been associated with significantly reduced stress transmission to the peri-implant area, which may also decrease long-term biological complications [ , ]. Despite favorable biomechanics, a recent systematic review and meta-analysis comparing the clinical performance of implant-supported single crowns has exhibited a high fracture rate for a first-generation resin-matrix ceramic system (33% after 5 years), which was statistically higher compared to all-ceramic systems such as lithium disilicate and zirconia (both ∼9%) [ ]. Apparently, the material composition negatively influenced its fracture stability in a demanding functional scenario, thus modifications in the filler compounds and weight content and microstructure of newly developed resin-matrix systems claiming to expand clinical application, especially for implant reconstructions, have been proposed and demand further investigations.
Restorative systems placed in the oral cavity interact with the oral environment and a complex chemically assisted mechanical scenario operate in the damage initiation and propagation through slow crack growth that leads to a reduction in strength [ , ]. Therefore, fatigue testing has been considered a clinically relevant predictor of materials resistance to environmentally assisted functional damage [ , ]. However, there is scarce information about the fatigue properties of several resin-matrix ceramics milled in anatomical geometries [ , ]. Hence, this study evaluated the probability of survival, failure modes and maximum principal stress (MP Stress) and strain (MP Strain) of implant-supported molar crowns of different resin-matrix ceramic systems when subjected to step-stress accelerated-life fatigue testing. The postulated null hypotheses were: (i) that resin-matrix ceramic systems would not present different fatigue survival, (ii) that resin-matrix ceramic systems would not present different fatigue failure mode, and (iii) that resin-matrix ceramic systems would not present substantially different MP Stress/Strain concentration and distribution.
2
Materials and methods
Eigthy-four Ti-6Al-4V abutments for implants with a locking taper connection (Universal abutments, Bicon LLC, Boston, MA, USA) and implants (3 mm well, 8 mm height, Bicon LLC) were obtained. A wax model maxillary first molar crown was scanned (InEos Blue, Sirona, Charlotte, NC, USA) and crowns were milled (inLab MC XL, Sirona) from four hybrid ceramic systems blocks (n = 21/group) [ , , ]:
- (i)
Shofu Hard: fine-structured zirconium silicate and methacrylate monomers (σ: 270 MPa, E: ∼10 GPa, Shofu Dental Corporation, Tokyo, Japan),
- (ii)
Cerasmart: feldspathic ceramic and methacrylate monomers (σ: 230 MPa, E: ∼10 GPa, GC Corporation. Tokyo, Japan),
- (iii)
Enamic: feldspathic ceramic network and methacrylate monomers (σ: 150 MPa, E: 30 GPa, Vita Zahnfabrik, Bad Sackingen, Germany), and
- (iv)
Shofu HC: zirconium silicate and methacrylate monomers (σ: 190 MPa, E: ∼10 GPa, Shofu Dental Corporation).
Crowns were finished and polished according to manufacturers’ instructions. A self-adhesive resin composite cement (RelyX U200, 3M Oral Care, St. Paul, MN, USA) was used to bond the crowns, which had their cementation surface previously etched with 5% hydrofluoric acid for 60 s (Enamic and Cerasmart), or sandblasted with aluminum oxide 50 μm particles with 0.2 MPa pressure for 10 s (Shofu Hard and Shofu HC) followed by cleaning with ethanol, and then treated with a layer of ceramic primer (RelyX Ceramic Primer, 3M Oral Care, MN). A crown/implant positioning apparatus was fabricated to allow repeated pouring of the self-curing acrylic resin (Orthodontic Resin, Dentsply-Caulk, Philadelphia, PA, USA) and standardized embedding of samples to be fixed on the testing machine.
2.1
Step-stress accelerated life testing (SSALT) and reliability analysis
SSALT was performed in an axial direction at constant frequency of 10 Hz with the spherical indenter (6 mm diameter, d -2 Steel) positioned at the mesiolingual cusp (ElectroPuls™ E3000 Linear-Torsion system mechanical testing equipment, Instron, Norwood, MA, USA). Crowns were submerged in water at room temperature throughout fatigue testing. Although details of SSALT method used in this study has been described elsewhere [ , ], in brief, three profiles were designed as mild, moderate, and aggressive, with the number of specimens assigned to each group being distributed in the ratio of 3:2:1, respectively (n = 9 in the mild, n = 6 in the moderate, and n = 3 in the aggressive load profile). These profiles are named based on the step-wise load increase that the specimen will be fatigued throughout the cycles until a certain level of load, meaning that specimens assigned to a mild profile will be cycled longer to reach the same load level of a specimen assigned to the aggressive profile [ ]. Fatigue loads throughout SSALT ranged from 200 N up to a maximum of 2000 N with a steady increase in load as a function of elapsed cycles. The test was conducted until specimen failure or suspension (absence of fracture until the end of a profile). The findings were recorded as fracture load, number of cycles in which the sample failed, and stress profile.
Data analysis consisted of an underlying life distribution that described the life data collected at different stress levels and a life-stress model that quantified the manner in which the life distribution changed across different stress levels [ , , ]. Thus, the Weibull Distribution was chosen to fit the life data collected in SSALT. Considering the time-varying stress model of SSALT, the inverse power law relationship was selected to extrapolate a use level condition considering the cumulative effect of the applied stresses, commonly referred as the cumulative damage model. From the extrapolated use level condition, a variety of functions could be derived. Hence, the use level probability Weibull curves (probability of failure versus number of cycles) with a set load of 300 N at 90% two-sided confidence interval were calculated and plotted (CI: 90%) (Synthesis 9, Alta Pro, Reliasoft, Tucson, AZ, USA). Also, the reliability was calculated for completion of a mission of 50,000 cycles at 300, 500, and 800 N and the differences between groups were identified based on the non-overlap of the CI. Parameters estimation was accomplished via maximum likelihood estimation (MLE) method, and CI were approximated using the Fisher matrix approach.
If the calculated use level probability Weibull beta parameter of any group was <1, then a Weibull 2-parameter calculation of the Weibull modulus, a unitless parameter that measures the variability of the results, and the characteristic strength, load at which 63.2% of the specimens would fail, was presented using the final load failure or survival (Weibull 9++, Reliasoft) [ , , ]. Weibull 2-parameter contour plot (Weibull modulus vs. characteristic strength) was graphed to determine statistical differences through the non-overlap of CI. Parameters estimation was accomplished via MLE method, and 90% CI were approximated using the Fisher matrix approach.
2.2
Fractographic analysis
Failed samples were first inspected in polarized light stereomicroscope (AxioZoom V16, Zeiss, Oberkochen, Germany) using Z-stack capture mode which uses automated sequential imaging along the z-plane and sticks them within the same depth of focus (ZEN 2.3 PRO, Zeiss) to depict fracture planes and allow fractographic analysis under magnifications up to 260×. Subsequently, fractured samples were evaluated under scanning electron microscope (SEM, Model 3500S, Hitachi Ltd., Osaka, Japan). Criteria used for failure were cohesive fracture within the composite (chipping), fracture exposing the abutment or fracture in several pieces (catastrophic), and abutment fracture.
2.3
In silico voxel based finite element analysis (FEA)
Each stereo lithography (STL) file scanned was voxelized with 0.05 mm/voxel in the voxel based FEA software (VOXELCON2015, Quint, Fuchu, Japan). Total voxel number was 5,880,870. Elastic modulus of each material was summarized in Table 1 . Poisson’s ratio of each material was set to 0.38 according to that of dental composites [ ]. Z axes for FEA models were defined along axial direction. Static loads of 300 N, 500 N, and 800 N were loaded on an area according to loading condition of in vitro fatigue testing as shown in Fig. 1 . CAD/CAM resin-matrix ceramic crowns were perfectly cemented on the abutment and the bottom surface of the abutment was completely fixed along to X, Y, and Z axes. By following aforementioned boundary conditions, the voxel based FEA was conducted using matrix solver with an error of 0.0001 [ ]. Maximum principal stress (MP Stress) and strain (MP Strain) distribution were assessed, and maximum values were compared.
Elastic modulus (MPa) | Poisson ratio | |
---|---|---|
Shofu Hard | 8,800 | 0.38 |
Cerasmart | 7,300 | 0.38 |
Enamic | 15,000 | 0.38 |
Shofu HC | 6,000 | 0.38 |
Abutment | 114,000 | 0.34 |
Cement | 8,000 | 0.33 |

3
Results
The calculated probability of survival for completion of a mission of 50,000 cycles at 300, 500, and 800 N is presented in the Table 2 . All CAD/CAM hybrid ceramic systems presented no significant difference on the probability of survival at 300 N, although Shofu HC showed notably lower values (79%) than the others (more than 96%). While Shofu Hard, Cerasmart and Enamic crowns kept their survivability higher than 90% at a set load of 500 N, a significantly reduced reliability was demonstrated by Shofu HC crowns (59%). Similarly, the cumulative damage reaching a high-magnitude mission (800 N) would result in significantly reduced reliability to Shofu HC (12%), as well as Enamic (57%) crowns, both significantly different between them. Indeed, Shofu Hard and Cerasmart implant-supported crowns demonstrated no significant difference for all calculated missions corresponding to molar forces range (300–800 N), and both systems maintained the probability of survival higher than 93%.
Shofu Hard | Cerasmart | Enamic | Shofu HC | ||
---|---|---|---|---|---|
300 N | Upper Bound | 100 | 99 | 99 | 89 |
Reliability | 98 aA | 96 aA | 97 aA | 79 aA | |
Lower Bound | 89 | 88 | 88 | 64 | |
500 N | Upper Bound | 99 | 99 | 96 | 71 |
Reliability | 97 aA | 96 aA | 90 aA | 59 bA | |
Lower Bound | 86 | 86 | 76 | 39 | |
800 N | Upper Bound | 98 | 99 | 72 | 26 |
Reliability | 93 aA | 96 aA | 57 bB | 12 cB | |
Lower Bound | 80 | 80 | 38 | 4 |
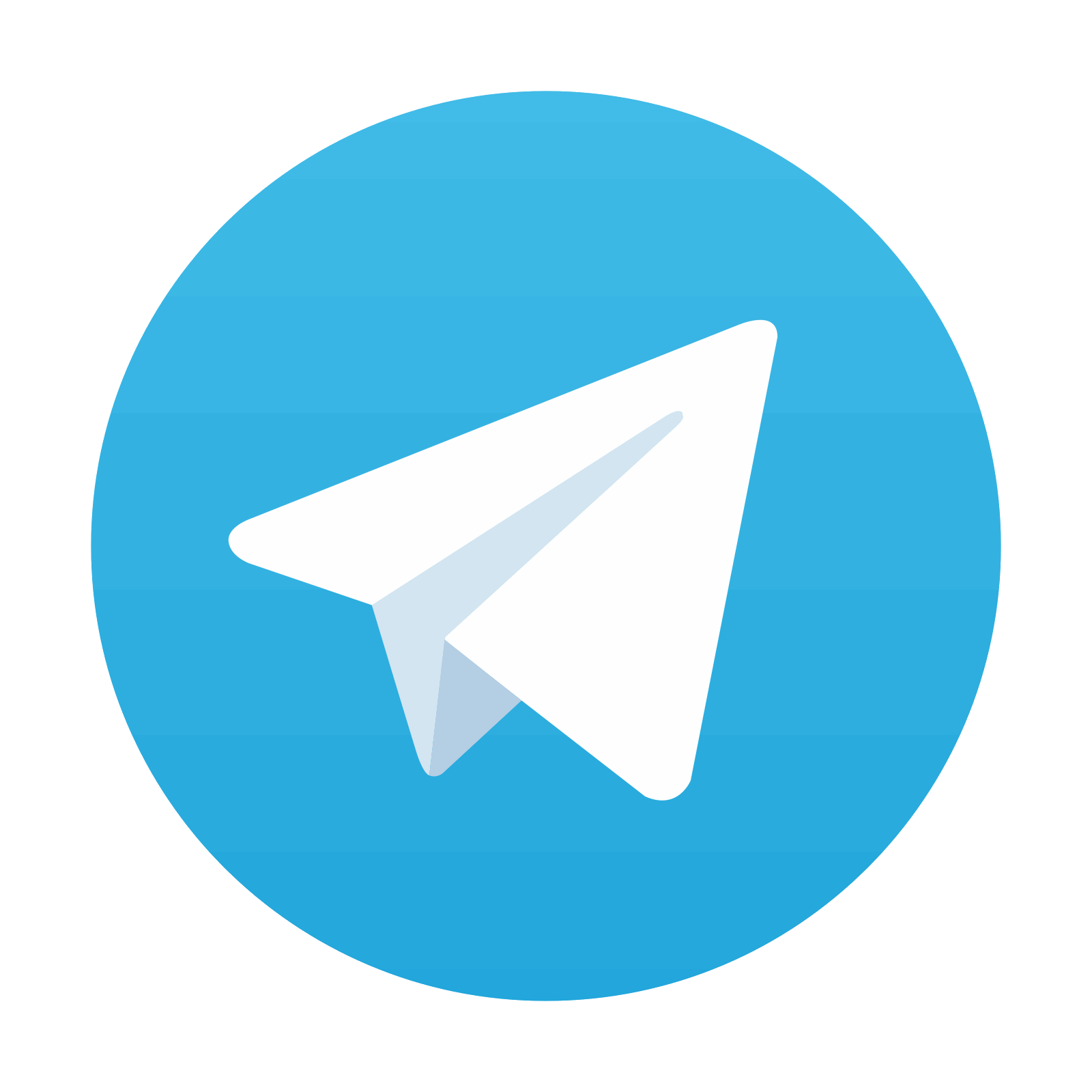
Stay updated, free dental videos. Join our Telegram channel

VIDEdental - Online dental courses
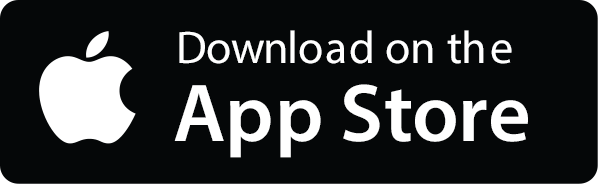
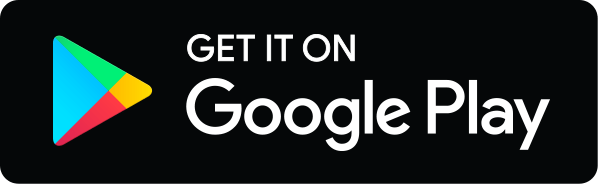
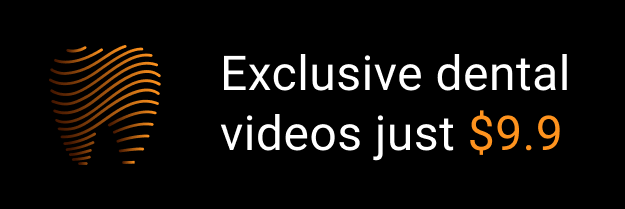