div epub:type=”chapter” role=”doc-chapter”>
J.-W. Choi, J. Y. LeeThe Surgery-First Orthognathic Approachhttps://doi.org/10.1007/978-981-15-7541-9_9
9. Virtual Surgical Planning and Three-Dimensional Simulation in Orthognathic Surgery
3D technology, 3D computer simulation3D printingWafer3D printed waferOcclusion
9.1 Introduction
We are working in the era of the fourth industrial revolution, which includes three-dimensional (3D) computer simulation, computer-aided design–computer-aided manufacturing technology, 3D printing technology, artificial intelligence, augmented reality, virtual reality, and navigation. Some doctors may believe that these technologies are mostly used in industry; however, 3D technology is already a reality in medicine.

Since the 2000s, the rapid prototype model has been commonly used in craniofacial surgical planning, including for orthognathic surgeries. A three-dimensional printing model provides surgeons with tactile, hands-on planning experiences as well as the ability to check bony anatomies and bony interferences in advance of actual surgeries

If we adopt the new three-dimensional (3D) technologies, they will help us reach our final destinations quickly, precisely, and reproducibly. This is the role of 3D simulation and 3D printing technology in medicine
In orthognathic surgery, the traditional dental model setup is a typical example of presurgical simulation, and the occlusal splint or wafer provides a very good example of a surgical guide that connects the simulation with the real surgery.
Now that orthodontists and maxillofacial surgeons have used these simulation processes for a long time, they can adopt the brand-new 3D technologies more easily, in my opinion.
Simulation-guided orthognathic surgery (SGOS) is the process of using 3D patient data to create a stepwise guide for making an accurate diagnosis, creating 3D cephalometric measurements, virtually planning the surgical steps, and predicting the consequences of these steps on the dentoskeletal complex and soft tissue envelope.
I adopted 3D simulation and 3D printing technology for orthognathic surgeries in 2012. Prior to that, even without 3D simulation and 3D printing, orthognathic surgeries were successfully performed, in my practice. However, the adoption of these 3D technologies has allowed me to prepare for the surgery more intensively, more precisely perform operations, and more objectively evaluate the surgical outcomes.
Several reports have recently aimed to establish the basics of this domain. Thus, the broad lines of the technique (starting with data acquisition and passing through segmentation, surgical step simulation, and plan-transport template designs) are now widely accepted [4–6]. Furthermore, the development of simulation software has allowed prediction of soft tissue responses and provided the aesthetic standards for different populations (aesthetic-centered virtual planning) [7–9]. Studies on the efficacy of using virtual surgical planning (VSP) reported higher osteotomy and repositioning accuracies and greater timesaving during the planning and surgical stages than conventional methods [10–13].
As expected, the increased popularity of these techniques has drawn attention to measuring outcome accuracies and comparing them with conventional methods, as well as comparing different techniques [14–16]. However, measuring SGOS accuracy has two considerations. The first is the applicability of comparing VSP to real surgery, as VSP should be measured as a separate entity with its own controlling factors, regardless of its utility in planning the accuracy of surgical techniques (Fig. 9.1). The second is that the absolute difference between measurements mainly depends on large travel distances. Therefore, another method for detecting the accuracy of small movement achievements should be used to investigate the factors affecting VSP applicability accurately. For the understanding of the readers, I introduce how to apply the 3D computer simulation and patient-specific 3D printing technology to the orthognathic surgery. Then I will share my outcomes of my investigation related to simulation guided orthognathic surgery.
9.2 Methods [17, 18]
9.2.1 Data Acquisition

An example of typical vertical facial asymmetry. Digital Imaging and Communications in Medicine data are taken from a computed tomography scan (1-mm thickness) to create three-dimensional volume rendering images
Subsequently, the two sets of data are introduced to the simulation and aligned using a semiautomatic process. In cases where dental landmarks are unclear, an additional data file containing scanned dental arches is merged with the skeletal 3D file. We use two types of software during the study: the Mimics program (version 19, Materialise-NV, Leuven, Belgium) is mainly used for bone segmentation and cephalometric analyses and the Morpheus 3D program (Dental Solution MDS, Seoul, South Korea) is used for soft tissue simulation; both are used in VSP.
9.2.2 Virtual Surgical Planning

Three-dimensional computer modelling is done by combining data from the dental scan with those from the segmentation of each axial scan in the Digital Imaging and Communications in Medicine data
9.2.3 Template Design and Manufacture

While traditional orthognathic surgery uses the location of the mandible for fixation of the maxilla, three-dimensional technology allows the maxilla to be fixed based on the location of the maxilla, itself, using a three-dimensionally printed osteotomy guide and occlusal wafer. This figure also shows the three-dimensional printing guide that is used to stabilize the proximal segments of the mandible
9.2.4 Surgical Intervention

Clinical applications of a three-dimensional printing guide for orthognathic surgery, including a maxillary fixation guide and a three-dimensionally printed guide for stabilizing the proximal mandibular segments
9.3 Postoperative Analysis

A patient with facial asymmetry and increased facial height undergoing three-dimensional, simulation-guided two-jaw surgery. (a) Preoperative appearance. (b) Postoperative appearance. (c) Analysis of esthetic facial measurements and proportions, relative to Korean population standards. (d) Soft tissue response to bony segment repositioning
CT was performed within the first 3 postoperative weeks; the DICOM file was uploaded into the simulation software to create the early postoperative 3D model.
9.3.1 Measurement Protocol (Fig. 9.8)

The simulation-guided orthognathic surgery process. The virtual surgical plan is connected with the real orthognathic surgery via the 3D printing guide
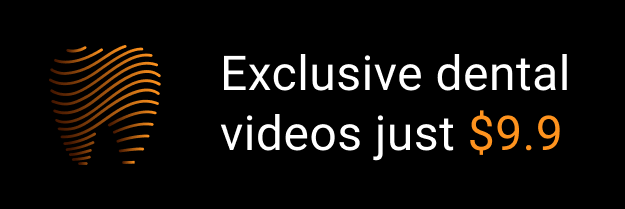