div epub:type=”chapter” role=”doc-chapter”>
J.-W. Choi, J. Y. LeeThe Surgery-First Orthognathic Approachhttps://doi.org/10.1007/978-981-15-7541-9_10
10. Three-Dimensional Photogrammetric Analysis in Orthognathic Surgery
3D camera3D photogrammetryStructured lightPhotogrammetry3D simulationSoft tissue simulationOrthognathic surgeryFrontal soft tissue
10.1 Introduction
10.1.1 Two-Dimensional (2D) Versus Three-Dimensional (3D) Cameras
Two-dimensional photographs have been the standard for planning and evaluating facial aesthetic outcomes for a long time. However, technological advances have made the 3D camera a reality. Different from the conventional 2D photograph, 3D photogrammetry allows the measurement of exact values for various facial soft tissue landmarks; even precise measurements of areas and volumes are possible. I believe that this technology represents a paradigm shift for all medical fields dealing with the face and is something that I have anticipated for a long time.
With recent advances in technology, 3D photogrammetry allows objective and reliable data acquisition with fewer errors. In addition, it facilitates preoperative planning and the simulation of postoperative outcomes.

Presently, there are two types of three-dimensional (3D) cameras available on the market. The structured light-type consists of a single projector and a single camera. This system provides highly accurate 3D mesh data but has difficulty capturing a movable object. The stereophotogrammetry type consists of two cameras. This system is able to capture movable objects accurately but the accuracy of the 3D mesh data is reduced because it is unable to find all corresponding points if points with characteristic features are absent, like in patients with smooth skin
The structured light type consists of a single projector and a single camera. This system is able to capture 3D mesh data accurately but has difficulty capturing movable objects. Conversely, the stereotype system consists of two cameras and is able to capture moveable objects; however, the accuracy of its 3D mesh data is reduced because it is unable to locate all the corresponding points on a structure if these points do not possess adequate characteristics, such as on a patient with a smooth face.
When we compare these systems in practice, the structured light type requires the use of an industrial camera to capture multiple images rapidly. Therefore, the texture quality is satisfactory, 3D mesh precision is high, and the speed of the 3D image processing is high.

While the structured light-type three-dimensional camera provides an image of satisfactory texture quality, the three-dimensional photogrammetric camera produces an image of high texture quality
The price of the industrial cameras used in structured light photogrammetry is falling and the resolution is improving. Thus, the texture quality is getting better. While the stereotype systems are less likely to improve further because the texture quality is already high, the structured light-type systems have the possibility of continuous improvements in texture quality and mesh precision.

Comparing 3D imaging processing speed, The structured light-type three-dimensional camera can process an image in less than 25 s, whereas the stereotype camera requires more than 2 min
However, why are we skeptical of this new technology in real practice? There have been some obstacles to the broad adoption of this technology. The resolution of the 3D camera is not the same as that of a standard digital single-lens reflex camera and the image processing time of 3D images is too long. Additionally, the absence of user-friendly software has contributed to the failure of this technology to be commonly adopted. Finally, how can we use the preoperative simulation images in real surgery?
In this chapter, I will explain how I have been using 3D photogrammetry in my practice.
10.1.2 3D Photogrammetry in Orthognathic Surgery
Orthognathic surgery, used to correct dentofacial anomalies, has two key goals: correction of malocclusion and good postoperative facial aesthetics. Orthognathic surgery can alter facial soft tissue contours by changing skeletal tissue and, therefore, can be used to create a more attractive face. However, the effect of skeletal surgery on soft tissue profiles is not easy to predict [1].

Traditional two-dimensional lateral cephalometric analysis. Preoperative and postoperative views

Traditional two-dimensional photogrammetry versus three-dimensional photogrammetry. Three-dimensional photogrammetry provides precise objective measurements of various soft tissue landmarks

Three-dimensional camera based on stereophotogrammetry. (a) A three-dimensional stereophotogrammetric camera system (second generation, Vectra, Canfield, USA). (b) The process of axis calibration. During this procedure, correct frontal views can be obtained. The yaw, pitch, and roll of three-dimensional images led to these calibrations

Pre- and postoperative three-dimensional camera images based on the white structured light method. Although the three-dimensional photographs were not taken with natural head positions, they could be corrected, unlike two-dimensional photographs
10.2 Methods
From now on, I will introduce one of my investigations associated with the use of 3D camera in terms of the evaluation of the soft tissue changes before and after the orthognathic surgery. The study involved 25 consecutive patients with skeletal Class III dentofacial deformities. Between January 2008 and December 2009, these patients underwent two-jaw rotational setback surgery, using posterior maxillary impaction without maxillary advancement, at the Seoul Asan Medical Center. The patients were all Asians and had a mean age of 22 years (range, 17–32 years). Patients who underwent conventional maxillary advancement and mandibular setback and those who underwent anterior maxillary vertical reduction were excluded, as were patients with syndromic or disease-initiated dentofacial anomalies, such as secondary cleft-related dentofacial deformities [2].
10.2.1 Imaging Methods

Precision measurement validation of the three-dimensional camera. Three-dimensional camera measurement errors were investigated using a skull model to compare the differences between the actual and the three-dimensional measurements. For example, actual skull model lengths of 20 mm, 30 mm, and 50 mm were calculated to be 20.164 mm, 30.241 mm. and 50.567 mm, respectively, on the three-dimensional images
10.2.2 Landmark Identification

Three-dimensional soft tissue landmarks. These landmarks included the trichion, nasion, nasal tip, subnasale, stomion, and menton for vertical measurements, and the bizygomatic points, bigonial points, medial and lateral canthus, and oral commissures
10.2.3 Measurement of Actual Distances and Surface Areas on the 3D Images
Definitions of facial soft tissue landmarks
Category |
Landmarks |
Definitions |
---|---|---|
Facial proportion |
Upper 1/3 |
Trichion ~ Upper margin of eyebrow |
Mid 1/3 |
Upper margin of eyebrow ~ Subnasale |
|
Lower 1/3 |
Subnasale ~ Menton |
|
Mx & Mn |
Mx. Height |
Subnasale ~ Stomion |
Mandible height |
Stomion ~ Menton |
|
Transverse width |
Zygomatic width |
The length between the most lateral points in zygomatic arch (suborbitale level) |
Bigonial width |
The length between the most lateral points in mandible angle (stomion level) |
|
Nose |
Alar width |
The length between the alar |
Nasion—nasal tip |
Nasion ~ Nasal tip |
|
Nasal tip—subnasale |
Nasal tip ~ Subnasale |
|
Lip |
Upper vermilion area |
Redline in midpoint of upper lip ~ Stomion |
Lower vermilion area |
Stomion ~ Redline of lower lip |
|
Lip length |
The length between oral commissures |
|
Upper vermilion height |
The upper margin of upper red vermilion ~ stomion in midline |
|
Lower vermilion height |
Stomion ~ the lower margin of lower red vermilion in midline |
|
Surface areas |
Upper facial area |
The facial surface areas from subbrow to stomion level |
Lower facial area |
The facial surface areas from Stomion to menton level |
|
Symmetry |
Medial canthus- Rt. Oral commissure |
Endpoint of medial canthus—most latera point of oral commissure |
Medial canthus—Lt. oral commissure |
Endpoint of medial canthus—most latera point of oral commissure |
|
Lateral canthus—Rt. Oral commissure |
Endpoint of lateral canthus—most latera point of oral commissure |
|
Lateral canthus—Lt. Oral commissure |
Endpoint of lateral canthus—most latera point of oral commissure |
10.3 Results
10.3.1 Cephalometric Changes
I present my research data about the application of 3D photogrammetric analysis in clock wise rotational orthognathic surgery based on PNS impaction. This result will help you understand the impact of the 3D camera in orthognathic surgery. It allows me not only to analyze the lateral soft tissue profile, but also to analyze the frontal soft tissue profile objectively. I analyzed the surgical outcomes of 25 patients who underwent clock wise rotational orthognathic surgery based on PNS impaction ranging from 3mm to 8 mm. [21]. Jaw rotational orthognathic surgery resulted in satisfactory postoperative results in all 25 patients. The average mandibular setback was 10.7 mm (range, 5–17 mm), and there was no evidence of major upper airway hindrance. In 7 cases, reduction or advancing genioplasty procedures were performed, with an average reduction of 2.31 mm and an average advancement of 2.02 mm. The average maxillary posterior impaction was 4.5 mm. The pivot points of the two-jaw rotations were mostly A points. None of the patients underwent maxillary advancement. Thus, the average SNA increased from 77.4° to 77.8° although the average SNB decreased from 89.2° to 81.1°. The average occlusal plane increased from 8.7° to 11.4° because of the two-jaw rotations with posterior maxillary impaction (Fig. 4a–d).
10.3.2 Vertical Facial Proportions (Table 10.2)
Summary of facial proportion changes after two-jaw rotational setback orthognathic surgery
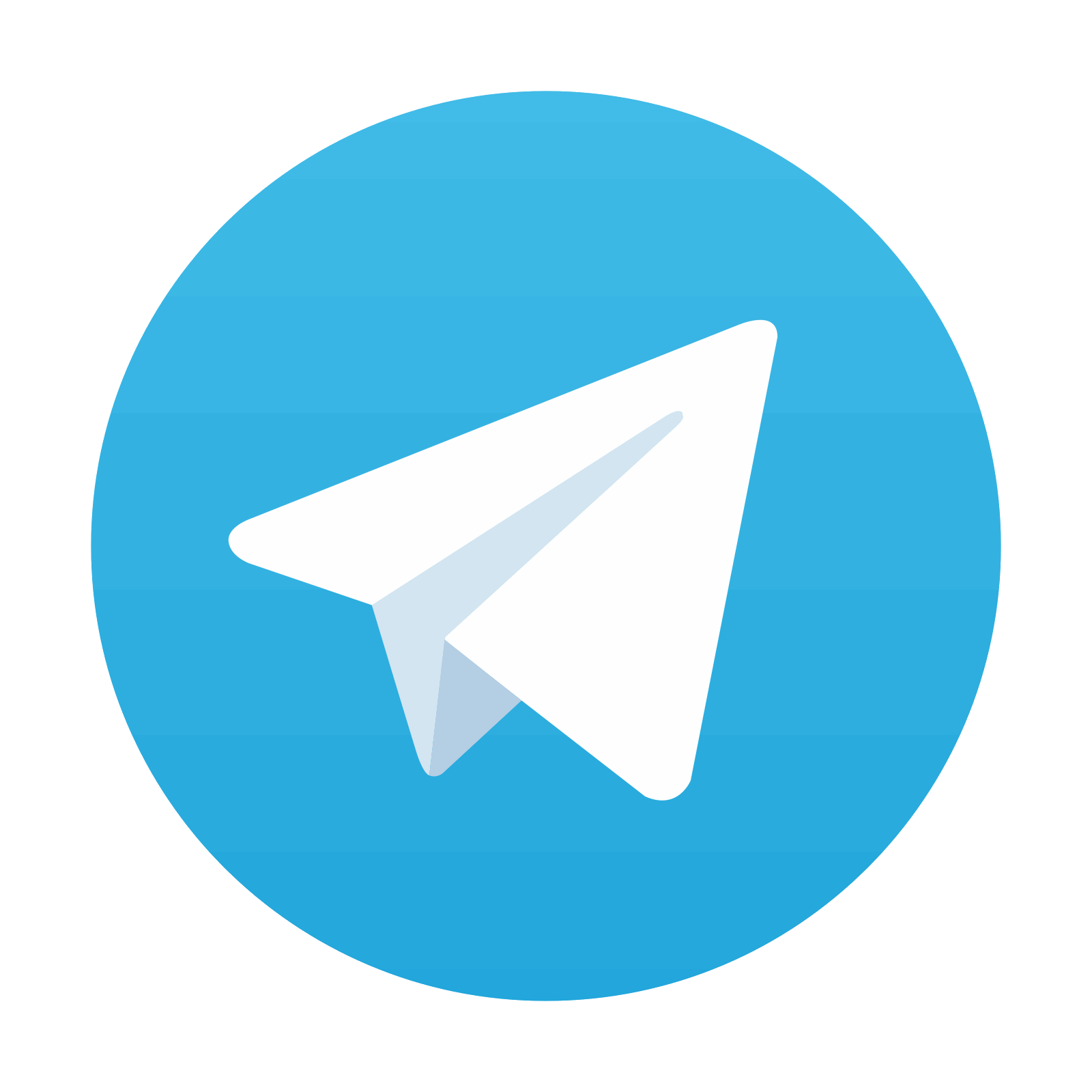
Stay updated, free dental videos. Join our Telegram channel

VIDEdental - Online dental courses
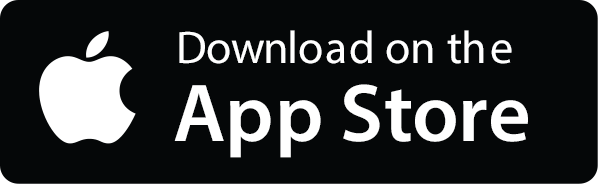
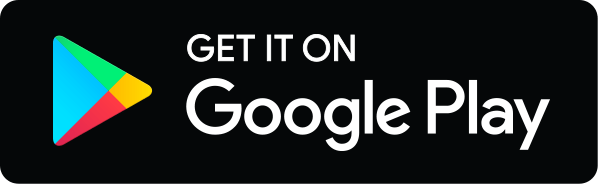
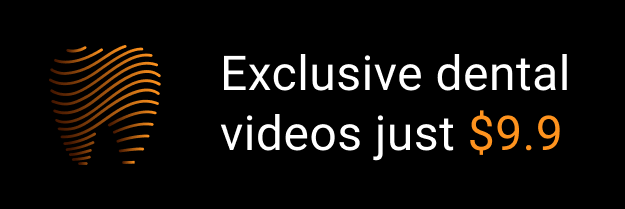