Abstract
Objective
The aim of this study was to elucidate whether marginal performance (in terms of % of continuous margins) can, or not, be predicted from μTBS values. A relationship was attempted between μTBS and % of continuous margins (% CM) before and after loading of class V restorations made with five restorative systems from both etch & rinse and self-etch approach.
Methods
Resin–dentin slabs and as well as class V cavities with cervical margins in dentin were prepared and restored using five adhesive systems (Scotchbond Multipurpose Plus (SMPP), James 2, Experimental Bond, Xeno III and iBond) with their corresponding restorative composites. Bond strength and percentages of continuous margins were calculated before and after thermal and mechanical loading. Statistical correlation was calculated between bond strength/marginal adaptation before loading and bond strength/marginal adaptation after loading of the same adhesives.
Results
Ranking of materials according to their performance was test method dependent. A low weak correlation was found between bond strength and percentages of marginal adaptation.
Significance
No correlation could be established between strength of the bond and percentages of continuous margins. A direct comparison of bond strength with marginal adaptation data may be misleading.
1
Introduction
Since the introduction of adhesion into the dental field its main goal has been to increase the performance of dental restorations in terms of preservation of tooth tissues and longevity. Restoration retention and marginal sealing are two among several clinical parameters on which the evaluation of adhesive fillings is based . Both criteria are closely associated with the strength of the bond between biomaterial and tooth. Two largely used methods of evaluating in vitro the quality of this bond are microtensile bond strength tests and the quantification of tight margins, i.e. marginal integrity or marginal adaptation. Bond strength assumes that the stronger the adhesion between the tooth and biomaterial, the better it will resist stress imposed by resin polymerization and oral function. Marginal integrity follows the concept that if the forces generated by polymerization shrinkage or by functional load exceed the strength of the bond, an observable gap will be formed at the margin of the restoration.
When selecting an adhesive system, practitioners would logically assume that an adhesive system providing high bond strengths is able to “guarantee” a certain clinical performance, which involves also the restorations’ marginal quality. It is here where an interpretation of bond strength data has been undertaken by relating it with the morphology of the adhesive interface. For instance, several studies attempted to establish a relationship between μTBS and microleakage , between μTBS and nanoleakage , between shear bond strength and microleakage and between percentages of marginal gaps and microleakage or dye penetration .
The relationship between μTBS and marginal adaptation is still not fully understood. Although it has been reported that marginal gap formation can be avoided with bond strength values exceeding 20 MPa , there is no study available that elucidated if marginal performance (in terms of % of continuous margins) can, or not, be predicted from μTBS values. In other words, based on the current knowledge it is still not possible to know if a material that provides high bond strength values is also able to provide restorations with high percentages of continuous margins.
Therefore, it was the purpose of the present study to clarify if a relationship exists between μTBS and percentages of marginal adaptation in a class V cavity model restored with five contemporary adhesives and their corresponding restorative composites, before and after thermo-mechanical load. The null hypothesis tested was that there is no correlation between microtensile bond strength and the percentages of continuous margins attained by a given restorative system, both before and after thermo-mechanical loading.
2
Materials and methods
The set-up used in the present study is graphically represented in Fig. 1 . Forty intact caries-free human molars were stored in 0.1% thymol solution for a maximum of 2 months following extraction until being used in the present study. After scaling and pumicing, the teeth were randomly assigned to ten experimental groups; five groups would be used for μTBS and five for the evaluation of the marginal adaptation ( Table 1 ).

Adhesive (batch #) Rest. Comp . (batch #) |
Manufacturer | Classification |
---|---|---|
SMPP (3BC,3AH,3NJ) Filtek Supreme (3BR) |
3M-ESPE, St. Paul, MN, USA | Etch & rinse (3 steps) 35% H 3 PO 4 , Primer, Bond |
James 2 (7310,7413) ELS (7140) |
Saremco Dental, Rebstein, Switzerland | Etch & rinse (2 steps) 35% H 3 PO 4 , Single bottle |
EB (25273,25487) Grandio (391267) |
VoCo, Cuxhaven, Germany | Self-etch (2 steps) Primer, Bond |
Xeno III (0311001490) Ceram X Duo (040100270) |
Dentsply, DeTrey, Konstanz, Germany | Self-etch (1 step) Liquid A and B to mix before application |
iBond (010046) Venus (030023) |
Heraeus Kulzer, Wehrheim, Germany | Self-etch (1 step) Single bottle |
2.1
Microtensile bond strength
Twenty-five molars were randomly assigned to 5 experimental groups. The teeth were prepared by cutting them perpendicular to their longitudinal axis using a slow-speed diamond saw (Isomet, Buehler Ltd., Lake Bluff, IL, USA) under water cooling in order to obtain mid-crown located dentin surfaces. A standard smear layer was created at the dentin surface by the use of 500 grits SiC paper. The teeth were subsequently bonded using a 3-step etch & rinse adhesive (Scotchbond Multipurpose Plus (SMPP), 3 M ESPE, St. Paul, MN, USA), a 2-step etch & rinse adhesive (James 2, Saremco Dental, Rebstein, Switzerland), a 2-step self-etch adhesive (Experimental Bond (EB), VoCo, Cuxhaven, Germany) and two 1-step self-etch adhesives (Xeno III, Dentsply DeTrey, Konstanz, Germany) and iBond (Heraeus Kulzer, Wehrheim, Germany). The composition and application procedure of each material are detailed in Table 2 . The adhesives were applied onto the entire dentin surface according to the manufacturers’ recommendations and light-cured with a halogen light-curing unit (Optilux 501, Kerr/Demetron, Danbury, CT, USA) with a constant relative power density output of >800 mW/cm 2 (Curing Radiometer Model 100, Serial No. 134089, Demetron Research Corp. Danbury, CT, USA). Two 2 mm thick layers of the corresponding composite resin were applied to the bonded surface and light-cured for 40 s each. After polymerization, the restored teeth were stored in distilled water for 24 h at 37 °C. Then the tooth-composite specimens were sectioned perpendicularly to the resin–dentin interface with a diamond saw (Isomet, Buehler Ltd., Lake Bluff, IL, USA) to produce 1.5 mm × 1.5 mm slabs. The slabs were hand-trimmed under stereo-microscopic observation (ensuring that the narrowest area was located at the bonding interface) in order to obtain a bonded area of 0.7 mm 2 . The slabs were then attached to the grips of a custom-made holder with cyanoacrylate adhesive (Zapit, DVA Inc., Corona, CA, USA) and stressed to failure at a crosshead speed of 1 mm/min using a universal testing machine (Vitrodyne V-1000 Universal Tester, John Chatillon and Sons, Greensboro, NC, USA). The tensile bond strength value of each slab was calculated as the force (N) at failure divided by the bonded area (mm 2 ) and expressed in MPa. Slabs that failed during the sectioning process were excluded from the results.
Adhesive system | Composition | Application procedure |
---|---|---|
SMPP | Etchant : 35% H 3 PO 4 | H 3 PO 4 etching in E and D for 15 s. |
Primer : Aqueous solution of HEMA and polyalkenoic acid | Rinse and dry with an applicator, leave surface moist and shiny. Apply the primer, dry for 5 s, see shiny surface. Apply bond, remove excess with little air and with an applicator, lightcure 10 s. | |
Adhesive : BIS-GMA, HEMA, photoinitiator system | ||
James 2 | Etchant : 35% H 3 PO 4 | Apply H 3 PO 4 60 s in E and D. Rinse at least for 30 s, dry well. Apply adhesive, leave 20 s and remove excesses with a new brush, do not blow. Lightcure 40 s. |
Primer + Bond : HEMA, UDMA, polyalkenoate methacrylized, hydroxypropylmethacrylate, glycerinedimethacrylate, catalysts, inhibitors, pigments | ||
EB | Water, ethanol, dimethacrylates, HEMA, carboxylate-methacrylates, phosphorusester-methacrylates, photoinitiators | Mix 1 drop of primer + 1 drop of adhesive for 5 s. Apply mixture on E/D and scrub in for 20 s. Dry 5 s. Lightcure 20 s. |
Xeno III | Liquid A : HEMA, purified water, ethanol, BHT, highly dispersed silicon dioxide | Mix A + B for 5 s. Apply 3 layers, leave for 30 s. Dry carefully until seeing a shiny surface, then remove the solvent, so dry well at the end. Lightcure 20 s. |
Liquid B : Pyro-EMA, PEM-F, UDMA, BHT, camphorquinone, ethyl-4-dimethylaminobenzoate | ||
iBond | UDMA, 4-META, acetone, water | Apply 3 consecutive layers. Dry slightly to evaporate the solvent until no movement is seen on the surface, obtain a glossy surface and then dry extremely well to evaporate water. Lightcure 20 s. |
2.2
Marginal adaptation
After scaling and pumicing, fifteen molars were randomly assigned to five experimental groups ( Table 1 ) and mounted on custom-made specimen holders with their roots in the center using cold-polymerizing resin (Technovit 4071, Heraeus Kulzer GmbH, Wehrheim, Germany). Prior to the mounting procedure the root apices of each tooth were sealed with two coats of nail varnish. In order to simulate intratubular fluid flow, a cylindrical hole was drilled into the pulpal chamber approximately in the middle third of the root and a metal tube with a diameter of 1.4 mm was then adhesively luted using a dentinal adhesive (Syntac Classic, IvoclarVivadent AG, Schaan, Liechtenstein). The pulpal tissue was not removed. This tube was connected by a flexible silicone hose to an infusion bottle placed 34 cm vertically above the test tooth. The infusion bottle was filled with horse serum diluted in a 1:3 ratio with 0.9% NaCl in order to simulate dentinal fluid under normal hydrostatic pressure of about 25 mm Hg. Twenty-four hours before starting the cavity preparations, by using a three-way valve, the pulp chambers were evacuated with a vacuum pump and subsequently filled with bubble-free, diluted horse serum. The intrapulpal pressure was subsequently maintained at 25 mm Hg throughout the testing, i.e. during cavity preparation, restoration placement, finishing and loading of the specimens.
One V-shaped standardized class V cavity was prepared on the buccal and on the lingual surface of each tooth with cervical margins in dentin. The cavity preparation was performed using 80 μm diamond burs (Diatech Dental, Coltène-Whaledent, Altstätten, Switzerland) under continuous water cooling, which was replaced by a new one after four dental preparations. The dimensions of the V-shaped cavities were 3.0–3.5 mm in diameter, 2.5–3.0 mm in height and 1.5 mm in depth. The entire cavity was finished using 15 μm finishing diamond burs (Diatech Dental, Coltène-Whaledent, Altstätten, Switzerland). Then the cavity preparations were checked for marginal imperfections such as fractures or chipping under a stereo microscope (Wild M5, Wild AG, Heerbrugg, Switzerland) at 12× magnification and corrected if necessary. The same adhesive systems and corresponding restorative composites were applied strictly following manufacturers’ instructions. After placement and light-curing of the adhesive with a halogen light-curing unit (Optilux 501, Kerr/Demetron, Danbury, CT, USA), the composites were inserted into the cavity in two layers; the first one cervically up to one half of the cavity and the second layer occlusally, filling the other half of the cavity. Both layers were light-cured for 40 s each. Immediately after polymerization, the restorations were finished and polished by using flexible aluminum oxide discs with different grain sizes (SofLex PopOn, 3 M ESPE AG, Seefeld, Germany). After storage in the dark in a 0.9% saline solution at 37 °C, the restored teeth were loaded in a computer controlled chewing machine. Thermal and mechanical loading was applied simultaneously . Thermal cycling was carried out in running water with temperatures changing 3000× from 5 °C to 50 °C with a dwelling time of 2 min each. The mechanical stress comprised a total of 1.2 millions load cycles transferred to the center of the occlusal surface with a frequency of 1.7 Hz and a maximal load of 49 N. The load was applied using a natural lingual cusp taken from an extracted human molar. The dentinal fluid simulation under stress was achieved by a modification of the stress apparatus test chambers. A correspondingly higher position of the serum infusion bottle compensated for the slight pressure generated by the circulating water in the test chambers . Immediately after completion of the polishing procedure ( before loading ) and after loading respectively, impressions were made of each class V restoration with a polyvinylsiloxane material (President light body, Coltène-Whaledent, Altstätten, Switzerland). Subsequently, gold coated epoxy replicas were prepared for the computer assisted quantitative margin analysis in a scanning electron microscope (XL20, Philips, Eidhoven, Netherlands) at 200× magnification.
2.3
Statistical analysis
The statistical software used for data evaluation was SPSS 14.0 for Windows. As the distribution of data was not normal (Kolmogorov–Smirnov test) and variances among specimens unequal (Levene’s test), non-parametric methods were used. Median values were calculated and statistical analysis was run based on the mean rank instead of mean and standard deviation. Analysis and report of data as medians is normally the rule in non-parametric tests. The mean ranks of marginal adaptation before and after loading were calculated from the raw data reported in a previous study . Differences among groups in “bond strength”, “marginal adaptation (MA) before load” and “MA after load” were assessed with Kruskal–Wallis one-way analysis of variance by ranks. Multiple comparisons between rank means were performed with Bonferroni post hoc test, with the p value adjusted to 0.0025. Spearman’s rank correlation coefficient or Spearman’s rho (the non-parametric version of Pearson correlation) was calculated based on the ranks of data of the 5 groups to know how closely x (μTBS) and y (MA before and after load) were associated in a straight-line relationship. The level of confidence was set to 95%.
2
Materials and methods
The set-up used in the present study is graphically represented in Fig. 1 . Forty intact caries-free human molars were stored in 0.1% thymol solution for a maximum of 2 months following extraction until being used in the present study. After scaling and pumicing, the teeth were randomly assigned to ten experimental groups; five groups would be used for μTBS and five for the evaluation of the marginal adaptation ( Table 1 ).
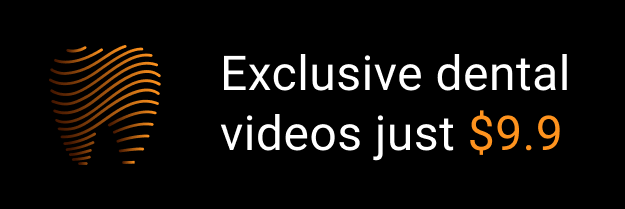