Abstract
Objectives
Polymerization shrinkage of a composite in a high configuration-factor (C-factor) cavity leads to polymerization shrinkage stress. In the prevention of high polymerization shrinkage stress, a low-shrinking silorane composite (Filtek Silorane, FS, 3M ESPE) was recently marketed. Therefore, we investigated the effect of C-factor (flat surface vs. class-I cavity) and different composite application protocols on the bonding effectiveness of a low-shrinking composite to human dentin.
Methods
A low-shrinking silorane-based composite (FS) and a conventional methacrylate-based composite (Filtek Z100, 3 M-ESPE) were bonded to standardized occlusal class-I cavities (4 mm × 4 mm × 2.5 mm) and to flat mid-coronal dentin surfaces using the two-step self-etch adhesive ‘Silorane System Adhesive’ (3 M-ESPE). Eight experimental groups were formed, according to the following treatment protocols: Z100 ‘flat’(1), Z100 ‘cavity’(2), FS ‘flat’(3), FS ‘flat/bulk-filled’(4), FS ‘cavity/bulk-filled’(5), FS ‘cavity/layered-filled’(6), FS ‘cavity/flowable cured’(7) and FS ‘cavity/flowable uncured’(8). For each group, at least 5 teeth were used. After 1 week of water storage, the teeth were sectioned to 1 mm × 1 mm (non-trimmed) sticks to measure the micro-tensile bond strength (μTBS).
Results
No statistical difference in μTBS was recorded between Z100 ‘flat’(1) and FS ‘flat’(3). Bonding to class-I cavity dentin lowered the μTBS in all groups, but this was only statistically significant for FS ‘cavity/bulk-filled’(5) and FS ‘cavity/flowable uncured’(8).
Significance
Using the two-step self-etch Silorane System Adhesive, the conventional composite Filtek Z100 and the low-shrinking composite Filtek Silorane bonded equally well to dentin. Bulk-filling with Filtek Silorane (FS ‘flat/bulk-filled’(4)) significantly decreased the μTBS, suggesting that factors other than polymerization shrinkage influenced the μTBS.
1
Introduction
The majority of composites used in restorative dentistry have their common basis in the radical polymerization of methacrylates. By conversion of monomer molecules into a polymer network, van der Waals spaces are exchanged for shorter covalent bindings. This causes considerable shrinkage of the resin composite, basically due to a closer packing of molecules. This shrinkage is clinically undesirable as it puts stress to the vulnerable interface. The magnitude of the resultant stress depends in the first place upon the cavity configuration , the elastic modulus of the composite and the polymerization conversion rate . Clinically, the effects of polymerization shrinkage stress can be minimized using techniques as layering and incremental curing , but none of these techniques can eliminate the stress completely. Naturally, the surest way to avoid shrinkage stress is to use non-shrinking resins.
Recently, a low-shrinking composite, commercialized as Filtek Silorane (3M ESPE, Seefeld, Germany), was introduced. So-called siloranes replace the methacrylates in the resin matrix of dental composites . The ring-opening chemistry of the resin reduces shrinkage of the composite below 1 vol% . Filtek Silorane comes with a two-step self-etch adhesive, commercialized as ‘Silorane System Adhesive’ (SSA). First, a hydrophilic self-etch primer (Silorane System Adhesive Self-Etch Primer, SSA-Primer) is applied and light-cured separately prior to the application of a hydrophobic adhesive resin (Silorane System Adhesive Bond, SSA-Bond). SSA-Bond is methacrylate-based and is therefore compatible with conventional methacrylate composites as well. Further details on how SSA-Bond links to the silorane composite is currently not known, but according to the technical information provided by 3M-ESPE, SSA-Bond contains a hydrophobic bifunctional monomer in order to match the hydrophobic silorane resin . In addition, TEM adhesive–composite interfacial characterization did not disclose any separations between SSA-Bond and the silorane composite, while a clear incompatibility existed with the experimental precursor of SSA (before being referred to as ‘Hermes Bond’, 3M ESPE) .
Because of the low-shrinking nature of this silorane composite, the cavity configuration and the need for an incremental application technique is expected to be less critical. The hypotheses tested were therefore that (1) the cavity configuration and (2) the composite application technique do not affect the bonding effectiveness of a low-shrinking silorane-based composite to dentin. We determined the micro-tensile bond strength (μTBS) of Filtek Silorane in two different cavity configurations and using five different application techniques in comparison to that of a conventional resin composite (control).
2
Materials and methods
The study set-up is illustrated in Fig. 1 . Non-carious human third molars were stored in 0.5% chloramine solution at 4 °C and used within 1 month after extraction. The cusps of the crown were flattened using a gypsum trimmer, to have a flat reference plane for the standardized cavities. All teeth were mounted in gypsum blocks in order to ease manipulation. The teeth were randomly divided in eight experimental groups, with at least five teeth per group. In five groups, a standard box-type class-I cavity (4 mm × 4 mm) was prepared with a depth of 2.5 mm, using a high-speed handpiece with a cylindrical medium-grit (100 μm) diamond-bur (842, Komet, Lemgo, Germany) mounted in the MicroSpecimen Former (University of Iowa, Iowa City, IA, USA). The resulting C-factor of these cavities is 3.5, considerably higher than the C-factor of the flat control specimens (0.17). The 2.5 mm depth was chosen in order to ensure that the cavities could be filled and cured in bulk . In the other three groups, the 2.5 mm coronal part of the crown was removed using a slow-speed diamond saw (Isomet 1000, Buehler, Lake Bluff, IL, USA) so that the dentin depth achieved was equal to that of the bottom of the occlusal class-I cavity. The teeth were mounted in a chuck and a standard smear layer was produced by removing a thin layer of the surface using a similar cylindrical medium-grit (100 μm) diamond-bur (842, Komet) mounted in the MicroSpecimen Former. Next, the specimens were subjected to the following composite application protocols: Z100 ‘flat’(1), Z100 ‘cavity’(2), FS ‘flat’(3), FS ‘flat/bulk-filled’(4), FS ‘cavity/bulk-filled’(5), FS ‘cavity/layered-filled’(6), FS ‘cavity/flowable cured’(7) and FS ‘cavity/flowable uncured’(8). A detailed description of these protocols is given in Table 1 . Shade A3 was used for both composites. In the FS ‘flat/bulk-filled’(4) group, a silicone mould with the same dimensions as the class-I cavity was used to mimic the same polymerization kinetics as the ‘cavity’ groups, but with the same lower stress built-up (no bonding to silicone) as for the ‘flat’ surfaces. In FS ‘cavity/layered-filled’(6), an incremental composite application technique was used to optimize the adaptation of the silorane composite to the cavity-bottom. In FS ‘cavity/flowable cured’(7), a thin layer of an experimental silorane flowable composite (Exp. Silorane Flow, 3 M-ESPE) was applied and cured at the bottom before bulk-filling the cavities in a standardized way, by extruding the flowable resin out of the syringe with a uniform diameter up to a length of 7 mm (about 13 mg per cavity). The same application technique was used in FS ‘cavity/flowable uncured’(8), except that the experimental silorane flowable was not cured separately. The rationale for using flowable composite at the cavity-bottom was also to optimize the adaptation of the composite to the cavity-bottom. In all six experimental (FS) and two control (Filtek Z100) groups, the two-step self-etch Silorane System Adhesive was used to rule out differences between adhesive techniques. A detailed description of the system and its application method is provided in Table 2 . All the products were applied according to the manufacturer’s instructions. Light-curing was performed using a LED-curing device (L.E. Demetron 1, Demetron/Kerr, Danbury, CT, USA), which was checked for output, dust and composite remnants prior to use.

Group | Description | Total curing time (s) | |
---|---|---|---|
Filtek Z100 | ‘flat’(1) | The composite was applied to a flat surface and cured in three horizontal layers. | 2 × 20 + 40 |
‘cavity’(2) | The composite was applied in a cavity and cured in bulk. | 40 | |
Filtek Silorane | ‘flat’(3) | The composite was applied to a flat surface and cured in three horizontal layers. | 2 × 20 + 40 |
‘flat/bulk-filled’(4) | The composite was applied to a flat surface in a silicone mould and cured in bulk. | 40 | |
‘cavity/bulk-filled’(5) | The composite was applied in a cavity and cured in bulk. | 40 | |
‘cavity/layered-filled’(6) | The composite was applied in a cavity and cured in three horizontal layers. | 2 × 20 + 40 | |
‘cavity/flowable cured’(7) | A thin layer of an experimental Silorane Flowable was applied and cured separately, after which the composite was applied and cured in bulk. | 20 + 40 | |
‘cavity/flowable uncured’(8) | A thin layer of an experimental Silorane Flowable was applied, but not cured, after which the composite was applied and cured in bulk. | 40 |
Composition (wt%) | Application method | |
---|---|---|
Silorane System Adhesive Self-etch primer | 15–25% 2-hyroxyethyl methacrylate (HEMA); 15–25% bisphenol-a-diglycidyl ether dimethacrylate (BIS-GMA); 10–15% water; 10–15% ethanol; 5–15% phosphoric acid-methacryloxy-hexylesters; 8–12% silane treated silica; 5–10% 1.6-hexanediol dimethacrylate; <5% copolymer of acrylic and itaconic acid; <5% (dimethylamino) ethyl methacrylate; <3% DL-camphorquinone; <3% phosphine oxide. | (1) Shake the bottle briefly before dosing, so that the SSA-Primer becomes less viscous and flows to the dosing tip. |
SSA-Primer | (2) Place one drop of SSA-Primer into depression 1 of the dosing well, then close the boding well protect the SSA-Primer from light and prevent the evaporation of the solvent. | |
LOT 7AB EXP: 2009–02 | (3) Use the enclosed black disposable applicator to apply the SSA-Primer to the entire surface of the cavity and massage over the entire area for 15 s. | |
pH = 2.7 | (4) Use a gentle steam of air until the SSA-Primer is spread to an even film and does not move any longer. | |
(5) Cure the SSA-Primer for 10 s. | ||
Silorane System Adhesive Bond | 70–80% Substituted dimethacrylate; 5–10% Silane treated silica; 5–10% triethylene glycol dimethacrylate (TEGDMA); <5% Phosphoric acid-methacryloxy-hexylesters; <3% DL-camphorquinone; <3% 1.6-hexanediol dimethacrylate. | (1) Shake bottle briefly before dosing so that the SSA-Bond because less viscous and flows to the dosing tip. |
SSA-Bond | (2) Place one drop of SSA-Bond in depression 2 of the dosing well and close the dosing well to protect SSA-Bond from light. | |
LOT 7AB EXP: 2009–02 | (3) Use the enclosed green disposable applicator to apply SSA-Bond to the entire area of the cavity. | |
(4) Use a gentle steam of air until SSA-Bond is spread to an even film and does not move any longer. | ||
(5) Cure SSA-Bond for 10 s. |
After 1 week of water storage at 37 °C, the teeth were sectioned perpendicular to the adhesive–tooth interface using an automated water-cooled diamond saw (Accutom-50, Struers, Ballerup, Denmark) to obtain rectangular 1 mm × 1 mm non-trimmed micro-specimens for micro-tensile bond strength (μTBS) testing. The sticks were kept moisturized until tested. They were attached to a modified notched Ciucchi’s jig with cyanoacrylate glue (Model Repair II Blue, Sankin Kogyo, Tochigi, Japan) and stressed at a crosshead speed of 1 mm/min until failure in a LRX testing device (LRX, Lloyd, Hampshire, UK) using a load cell of 100 N. The μTBS was expressed in MPa, as derived from dividing the imposed force (N) at the time of fracture by the bond area (mm 2 ). When specimens failed before actual testing, a bond strength of 0 MPa was included in further statistical analyses. The actual number of pre-testing failures (ptf) was explicitly noted as well.
The mode of failure was determined light-microscopically at a magnification of 50× using a stereo-microscope (MSA 166305, Wild Heerbrugg, Switzerland). Some representative fracture surfaces were processed for scanning electron microscopy (Feg-SEM, Philips XL30, Eindhoven, The Netherlands) using common SEM specimen processing techniques, including fixation in 2.5% glutaraldehyde in cacodylate buffer solution, dehydration in ascending concentrations of ethanol, chemical drying using hexamethyldisilazane, and gold-sputter coating .
The μTBS-data were statistically appraised with a one-way ANOVA test; however, the origin of the tooth was added to the statistical model as a random effect factor. Post hoc pairwise comparisons (Tukey–Kramer) were conducted at a significance level of 5%. Failure analysis data were summarized by calculating the mean percentage of interfacial failure. All statistical analyses were carried out using the Statistica software (StatSoft, Tulsa, OK, USA).
2
Materials and methods
The study set-up is illustrated in Fig. 1 . Non-carious human third molars were stored in 0.5% chloramine solution at 4 °C and used within 1 month after extraction. The cusps of the crown were flattened using a gypsum trimmer, to have a flat reference plane for the standardized cavities. All teeth were mounted in gypsum blocks in order to ease manipulation. The teeth were randomly divided in eight experimental groups, with at least five teeth per group. In five groups, a standard box-type class-I cavity (4 mm × 4 mm) was prepared with a depth of 2.5 mm, using a high-speed handpiece with a cylindrical medium-grit (100 μm) diamond-bur (842, Komet, Lemgo, Germany) mounted in the MicroSpecimen Former (University of Iowa, Iowa City, IA, USA). The resulting C-factor of these cavities is 3.5, considerably higher than the C-factor of the flat control specimens (0.17). The 2.5 mm depth was chosen in order to ensure that the cavities could be filled and cured in bulk . In the other three groups, the 2.5 mm coronal part of the crown was removed using a slow-speed diamond saw (Isomet 1000, Buehler, Lake Bluff, IL, USA) so that the dentin depth achieved was equal to that of the bottom of the occlusal class-I cavity. The teeth were mounted in a chuck and a standard smear layer was produced by removing a thin layer of the surface using a similar cylindrical medium-grit (100 μm) diamond-bur (842, Komet) mounted in the MicroSpecimen Former. Next, the specimens were subjected to the following composite application protocols: Z100 ‘flat’(1), Z100 ‘cavity’(2), FS ‘flat’(3), FS ‘flat/bulk-filled’(4), FS ‘cavity/bulk-filled’(5), FS ‘cavity/layered-filled’(6), FS ‘cavity/flowable cured’(7) and FS ‘cavity/flowable uncured’(8). A detailed description of these protocols is given in Table 1 . Shade A3 was used for both composites. In the FS ‘flat/bulk-filled’(4) group, a silicone mould with the same dimensions as the class-I cavity was used to mimic the same polymerization kinetics as the ‘cavity’ groups, but with the same lower stress built-up (no bonding to silicone) as for the ‘flat’ surfaces. In FS ‘cavity/layered-filled’(6), an incremental composite application technique was used to optimize the adaptation of the silorane composite to the cavity-bottom. In FS ‘cavity/flowable cured’(7), a thin layer of an experimental silorane flowable composite (Exp. Silorane Flow, 3 M-ESPE) was applied and cured at the bottom before bulk-filling the cavities in a standardized way, by extruding the flowable resin out of the syringe with a uniform diameter up to a length of 7 mm (about 13 mg per cavity). The same application technique was used in FS ‘cavity/flowable uncured’(8), except that the experimental silorane flowable was not cured separately. The rationale for using flowable composite at the cavity-bottom was also to optimize the adaptation of the composite to the cavity-bottom. In all six experimental (FS) and two control (Filtek Z100) groups, the two-step self-etch Silorane System Adhesive was used to rule out differences between adhesive techniques. A detailed description of the system and its application method is provided in Table 2 . All the products were applied according to the manufacturer’s instructions. Light-curing was performed using a LED-curing device (L.E. Demetron 1, Demetron/Kerr, Danbury, CT, USA), which was checked for output, dust and composite remnants prior to use.
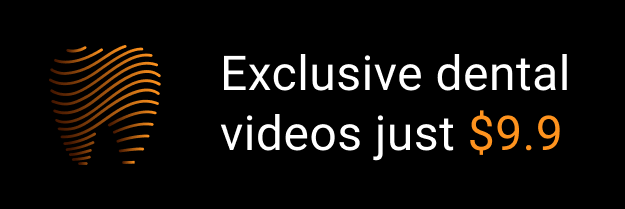