Fig. 10.1
Hess [76] intricacies of canal anatomy
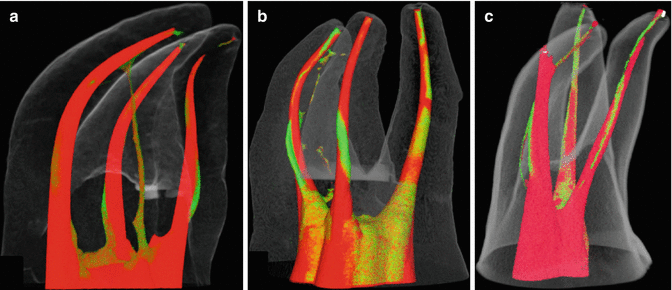
Fig. 10.2
Micro CT scans of canals following rotary file instrumentation (red) overlaid preoperative scans (green) [126]
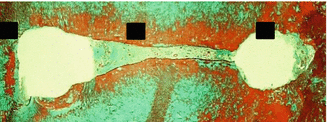
Fig. 10.3
Cross section of mesial root of mandibular molar demonstrating remaining tissue following root canal preparation utilizing NiTi rotary files [73]
The ability of an irrigant to dissolve pulp tissue, kill bacteria, and remove smear layer are well known and reported in the literature. Some irrigants are better suited for some jobs than others. Research has shown that the preparation (hand files and/or rotary NiTi files) of an infected root canal with standard needle irrigation (using sodium hypochlorite – NaOCl) and even placement of calcium hydroxide greatly reduces the number of viable bacteria in the canal [29, 30, 31, 38, 39, 46, 53, 121, 125, 132, 138, 142, 144, 145]. However, it does not eliminate all of them. Removal of biofilm is also limited, especially in the isthmuses and canal ramifications [28].
The flow characteristics of various size and shaped needles (open-ended versus side-vented) have also been described in the endodontic literature. Shen et al. [140] described how the velocity of fluid flow is affected by the needle design. Open-ended or beveled needles deliver fluid at a quicker pace than side-vented needles. This increase in velocity may aid in removing debris from the canal walls. The side-ported needles have their maximum velocity at the site of the port and the energy dissipates apically. Open-ended/beveled needles deliver irrigation solution about 1–2 mm past the end of the needle. This is a benefit if the needle cannot reach the apex of the root canal but a drawback if it does (potential irrigation accident). The side-ported needles allow solution to only reach about 1 mm past the end of the tip and still have similar risks and benefits of the open-ended needles. Shen et al. [140] also calculated the fluid pressure of the irrigant. This pressure may be of benefit in cleaning the canal walls by dislodging material such as biofilm. Of course the three dynamic parameters (fluid flow pattern, velocity, fluid wall pressure) are all affected by the fluid flow rate placed into the needle. Unfortunately, research has continuously shown that traditional needle irrigation fails to clean isthmuses, lateral canals, and cul-de-sacs to any extent ([15, 22, 69, 73, 74, 82, 94, 112, 151, 170]). Activation of the endodontic irrigant appears to be a necessary step in the more complete cleaning of the root canal system. A survey conducted by Dutner et al. [65] on the use of irrigants and adjunctive devices to aid irrigation found that almost 50 % of respondents use some type of irrigation aid. Of that group, 48 % used ultrasonics and 34 % utilized some form of sonic (subsonic) activation.
Ultrasonic Activation
Richman [128] first reported the application of ultrasonics in endodontics. He used a Cavitron® ultrasonic dental unit and concluded that since these cases were treated without untoward postoperative sequelae, the use of ultrasonics in root canal therapy held great promise.
In a series of articles published in the endodontic literature from 1976 to 1985, Martin and Cunningham [48–50, 104–109] reported on the use of ultrasound as a primary method of canal preparation and debridement in root canal therapy. The studies evaluated the efficacy of the endosonic method, its ability to eliminate bacteria from the canal, and its effect on extrusion of debris. Martin and Cunningham [106] concluded that endosonic root canal preparation was superior to hand preparation in mechanical and chemical debridement, disinfection, and final canal shaping. The ultrasonically energized file was reported to rapidly instrument the canal wall more efficiently with less operator fatigue. The “ultrasonically activated” irrigant facilitated cleansing and disinfecting actions within the root canal system.
Other studies of ultrasound as a primary method of instrumentation did not support the claims of Martin and Cunningham [107] that ultrasound removes more tissue from the canal than hand instrumentation. These studies [52, 67, 92, 154] found no difference in tissue removal between ultrasound and hand instrumentation. Also, when antibacterial effects were evaluated, no difference was found between the two instrumentation techniques [16, 60]. The overall performance of ultrasound as a primary method of instrumentation was not found to be superior to hand instrumentation. There was also a reported increase risk in straightening and perforating canals.
Martin and Cunningham [106] attributed the success of ultrasonic instrumentation to the interaction of the ultrasonic energy and the irrigating solution. They called this interaction the “synergistic system.” The irrigating solution achieves its active biological-chemical effects when it undergoes ultrasonation. The authors defined the primary effects of ultrasound as being cavitation and acoustic streaming. Transient cavitation was said to occur when the ultrasonic energy creates a bubble which grows to a certain point and then collapses. This collapse creates a pressure-vacuum effect which cleans irregularities in canals and kills microorganisms. The oscillatory effect of the ultrasonic instrument, which vigorously agitates the irrigating solution, is defined as resonant or stable cavitation. Combined with these effects of cavitation is a dispersal of physical energy which leads to physical acoustic (sound wave) streaming. This acoustic streaming purportedly enhances cleansing and disinfection.
When an ultrasonic wave is projected in liquid, negative pressure is created and causes the liquid to fracture, a process known as cavitation. Cavitation creates bubbles that oscillate in the projected ultrasonic waves. As the ultrasonic waves continue, these bubbles grow larger and become very unstable, eventually collapsing in a violent implosion. The implosions radiate high-powered shockwaves that dissipate repeatedly at a rate of 25,000 ~ 30,000 times per second (25–30 kHz). Additionally, the implosion of cavitation bubbles creates temperatures that exceed 5,000 ° C and pressures that exceed 500 atmospheres. The shock waves that are generated by the implosion travel at speeds over 500 mph within the fluid and this current is called acoustic streaming (www.tmasc.com, http://bluewaveinc.com) [21, 157]. Acoustic streaming can also be derived from the ultra-high-frequency oscillation of the ultrasonic tip/file placed in a fluid. Cleaning an object requires dissolving a contaminant (removing substance/object from a wall and putting it into solution) and then displacing the saturated layer of the contaminant so that fresh cleaning solution can come in contact with the unsaturated surface of the contaminant. The ultrasonic cavitation implosion effect is incredibly effective in doing this. The cavitation implosion effect is especially effective on unsmooth and out of reach surfaces that are normally inaccessible through conventional means such as irrigation alone.
To gain an insight into the mechanisms involved in ultrasonic instrumentation, Ahmad et al. [2] investigated the phenomena of cavitation and acoustic streaming as seen within the root canal space. In this initial study, the authors combined the phenomenon of resonant or stable cavitation, as described by Martin and Cunningham [106], with the phenomenon of acoustic streaming. These terms were combined because the rapid vortex-like motion associated with the vibrating file can also be associated with small gas bubbles set into oscillation by the fluctuating pressure field generated by the ultrasonic file. The group looked at transient cavitation using a photometric-sensitive image intensification system. This detection system monitored the light produced by the violent collapse of cavitation bubbles. A rectangular container filled with methylene blue dye and a dispersed film of polystyrene spheres was used to detect acoustic streaming. These spheres were illuminated so that patterns of acoustic streaming could be detected. Forty extracted maxillary anterior teeth were divided into four groups and instrumented either by hand or ultrasonically (Cavi-Endo®), using either water or 2.5 % sodium hypochlorite as an irrigating solution. The teeth were split longitudinally and evaluated for presence of a smear layer using a scanning electron microscope (SEM). It was determined that transient cavitation did not occur with the Cavi-Endo® unit and endosonic files. However, cavitation was produced when a scaler tip was inserted into the unit. The endosonic files produced acoustic streaming. When the amount of remaining debris was evaluated, there was no statistically significant difference between ultrasonic and hand instrumentation when either water or sodium hypochlorite was used as an irrigating solution. The authors concluded that acoustic streaming was more important to cleaning than cavitation. It was also concluded that the recommended technique of ultrasonic instrumentation did not produce sufficient acoustic streaming to effectively clean the canal. Dampening of the files may have caused the limitation in the production of acoustic streaming in the constricted canal system.
Ahmad et al. [3] continued the investigation of ultrasonic debridement by examining acoustic streaming. The authors defined acoustic streaming as the generation of time-independent, steady unidirectional circulation of fluid in the vicinity of a small vibrating object. Using the same method to detect acoustic streaming as described in the previous study [2], different size files were studied at different power settings. The power generated by the files was estimated by measuring the transverse displacement amplitudes that were produced. Transverse displacement amplitude was defined as half of the total distance moved by the pinpoint of light that appeared as a thin transverse line when a file oscillated. Twenty extracted maxillary anterior teeth were divided into two groups and instrumented with the second group using a modified technique in which a #15 endosonic file was allowed to freely vibrate at working length for 5 min. The results showed that each file generated an acoustic streaming field comprised of a primary field consisting of rapidly moving eddies in which the fluid element oscillated about a mean position, and a superimposed secondary field consisting of patterns of relatively slow, time-independent flow (Fig. 10.4). Approximately four clusters of eddies were generated by the #15 and 20 endosonic files. In the primary field, the direction of rotation of the fluid elements in each eddy was opposite to that of its immediate neighbor. The secondary field showed symmetrical longitudinal flows on both sides of the file (Fig. 10.5). Fluid was generally transported from the apical to coronal end of the file. The streaming velocity was greatest at the apical and least at the coronal end of the file. Smaller files generated relatively greater acoustic streaming, the velocity of which increased with increased power. These results were later confirmed by Jiang et al. [83]. Canals instrumented with the modified method were found to exhibit cleaner surfaces. The authors concluded that the freely vibrating file produced hydrodynamic shear stresses large enough to remove debris and the smear layer from the walls of the root canal, resulting in enhanced cleansing action. This hydrodynamic shear stress was proportional to streaming velocity. Therefore, the authors deduced that since streaming velocity was highest at the apical tip of the file, a concentration of stresses in the vicinity of the tip facilitated debridement.
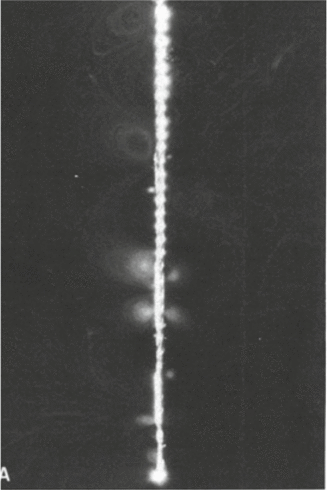
Fig. 10.4
Photo of acoustic streaming around a size 15 endosonic file [3]
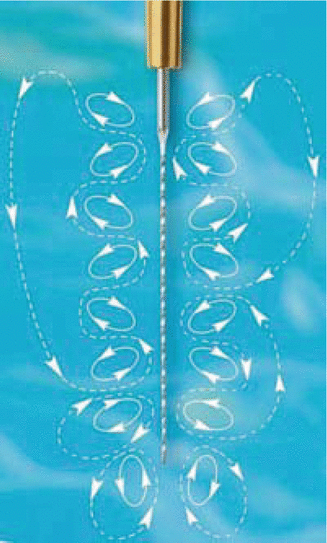
Fig. 10.5
Depiction of the waves generated around the vibrating ultrasonic file (ACTEON North America/Clinical Research Dental)
In another investigation into the mechanisms of ultrasound, Ahmad et al. [4] examined the effects of acoustic cavitation in debridement of root canals. The authors concluded that cavitation should not be regarded as an important mechanism in root canal debridement. Walmsley [166] also investigated the mechanisms of ultrasound in root canal treatment. His results agreed with Ahmad et al. [2], as he concluded that cavitation had little if any bearing on the debridement activity of ultrasound. This conclusion was based on his postulation that although the displacement amplitudes of the vibrating file were adequate to produce cavitation, the streamlined shape of the endosonic file was not conducive to generating a sound pressure field large enough to produce cavitation. Walmsley [167] also concluded that because of the transverse nature of the vibration pattern of the activated file, the effectiveness of ultrasonic instrumentation is limited by the dampening of the file against the root canal wall. Acoustic streaming is an effective mechanism in disrupting debris within the canals but is reduced when loading occurs against canal walls. Also, the synergistic activity of ultrasound and the irrigating solution does not take place when the file is not allowed to vibrate freely. Recently however, Jiang et al. [83] and Macedo et al. [97] showed that, within a simulated root canal system, cavitation did occur around the tip of an ultrasonically activated file, but that canal size (in relation to the file size) did impact the amount of cavitation produced.
Ahmad et al. [5] also reported that ultrasonic files can generate acoustic streaming both in the free field and in a small channel. Higher-velocity streaming was observed when smaller size files were employed and when the file was precurved (for curved canals). Light file-wall contact did not totally inhibit streaming, while severe file-wall contact inhibited movement of the file and, as a result, no streaming was observed. The positions and length scales of the streaming vortices appeared to be influenced by the presence of boundaries. In the free field, two rows of vortices were situated along the sides of the file (Fig. 10.6a), while in the small channel, the vortices were positioned above the surface of the file (Fig. 10.6b). These results indicated that it is possible for acoustic streaming to occur in a confined space, as in a root canal, provided that severe file-wall contact is avoided. They recommended that allowing the file to freely vibrate during some stage of treatment should be carried out in order to generate streaming in the root canal.
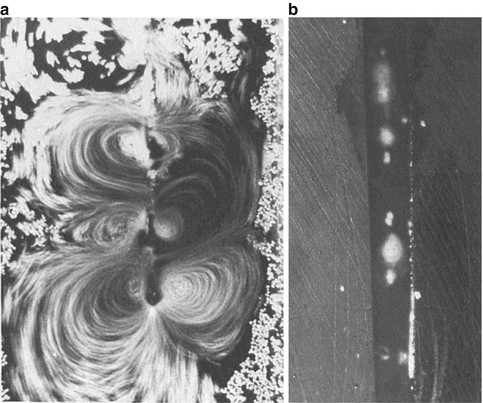
Fig. 10.6
(a) Acoustic streaming as generated around a free-moving file and (b) within a simulated root canal space [5]
Roy et al. [133] used sonoluminescence as an indicator of transient cavitation activity and photographic analysis was utilized as a means for detecting steady streaming, microstreaming, and stable cavitation with ultrasonic files. Measurements failed to indicate any strong correlation between registered driving power and the propensity to produce transient cavitation. Files that were pitted or possessed salient edges were very effective at generating transient cavitation. When observed, transient cavitation activity generally occurred near the tip of the straight file, provided the wall loading did not inhibit file motion. In all cases studied, steady acoustic streaming and stable cavitation were observed to varying degrees, depending on the amount of file to wall contact. Although the imposition of file-wall contact served to inhibit the production of transient cavitation, this action had relatively little effect on the ability of a file to produce a nominal level of streaming, microstreaming, and stable cavitation. They concluded that it was not prudent to ascribe enhanced cleaning effects to any one phenomenon, for it is likely that several factors are involved to varying degrees depending on the local conditions of application. Boutsioukis et al. [26] confirmed that an ultrasonically activated file contacts the root canal wall at least 20 % of time during activation. They reported that the depth of penetration of the file, the power utilized to activate the file, and the size of the root canal preparation all affected the amount of contact. However, they did report that cavitation in the fluid was detected even though there was file-wall contact.
Ultrasonic Energy Generation
There are two main types of ultrasonic energy generators used in dentistry which differ in their mode of operations. The magnetostrictive generator utilizes the principle of magnetostriction in which certain materials expand and contract when placed in an alternating magnetic field. Alternating electrical current (AC) from the ultrasonic generator is first converted into an alternating magnetic field through the use of a coil of wire inside the ultrasonic handpiece. The alternating magnetic field is then used to induce mechanical vibrations at an ultrasonic frequency in resonant strips of nickel or other magnetostrictive material that are attached to the surface to be vibrated [45]. Because magnetostrictive materials behave identically to a magnetic field of either polarity, the frequency of the electrical energy applied to the transducer (coiled wire) is half of the desired output frequency. This form of ultrasonic generation requires two transformations of energy: electrical to magnetic and magnetic to mechanical. During these energy transformations, heat is generated as energy is lost. Therefore, the efficiency of this type of generator is affected (as low as 50 %) and cooling measures are required to dissipate the heat generated. The frequency at which magnetostrictive generators operate is also limited. Due to size restrictions, they operate below 30 KHz [45]. To increase the frequency would require enlarging the wire coils and resonant metal strips, as well as increase the need for cooling, to the point of clinical infeasibility.
The piezoelectric generator, on the other hand, converts AC electrical energy directly into mechanical energy through the use of the piezoelectric effect. When electrical energy is applied to ceramic piezoelectric materials (i.e., barium titanate or lead zirconate titanate), there is a conversion and amplification of electrical energy into mechanical energy by way of vibration of the material within the ultrasonic handpiece. This vibration is then directly transmitted into the ultrasonic tip. This method allows piezoelectric transducers to operate well into the megahertz frequency range. Piezoelectric generators are more efficient (95 %) than magnetostrictive units due to the fact that magnetostrictive units require the two conversions of energy [45].
Passive Ultrasonic Irrigation (PUI)/Ultrasonically Activated Irrigation (UAI)
The terminology for the activation of irrigating fluids in root canals can be a bit confusing. Weller et al. [168] compared the efficacy of ultrasonics as a primary method of instrumentation and as an adjunct to hand instrumentation versus hand instrumentation alone. The authors concluded that ultrasonic instrumentation is not an alternative to hand cleaning but acts as an aid to increase debridement efficacy after hand instrumentation. In this study, the ultrasonic instrument was still used as an adjunct in canal preparation. Later research [12, 36, 69, 74, 82, 94, 112] looked at the use of ultrasonic instrumentation in a more passive manner, that is, it was utilized after hand instrumentation and without the intent to enlarge, instrument, or impact the walls of the root canal. Thus, the term passive ultrasonic irrigation (PUI) came to be. The “passive” portion indicated no active or intentional removal of dentin. Unfortunately, even though no intent is made to contact or alter the root canal walls, contact of the oscillating ultrasonic instrument on the wall occurs (see above). Due to this, the phrase ultrasonically activated irrigation (UAI) was recently suggested by Boutsioukis et al. [26]. Unfortunately the reader must be aware that these terms can and will be used interchangeably in the dental literature and that they represent the same general technique.
Research into PUI/UAI has looked at the ability of the technique to remove tissue and debris, bacteria, biofilm, calcium hydroxide and other medicaments, and smear layer. Research has also looked at the impact of using PUI/UAI in curved canals, the use of a smooth instrument versus an endodontic file, and the effects the size of the instrument and canal preparation size have on cleaning/debridement results. In general, PUI/UAI consists of the use of a size 15 or 20 endodontic-type file or wire attached to an ultrasonic handpiece from which ultrasonic energy is supplied. The depth of the file within the canal and the manner in which irrigating solution is supplied during the process has also been evaluated.
Available products that a clinician can utilize to provide PUI/UAI include file-holder tips (Brasseler). These tips allow for the insertion of a hand file (k-type file, r-type file, spreader, etc.) or a specially designed hand file-type inserts (diamond coated, fluted, smooth-sided, etc.) and secured for use in the canal (Fig. 10.7). Also available is the Irrisafe™ ultrasonic tip (Fig. 10.8) produced by Satelec Acteon which comes in different lengths and diameters and includes a port for the delivery of irrigating fluid, and the Sonofile tips (Fig. 10.9) by Satelec which are similar to the Irrisafe™ files but without the irrigation port.
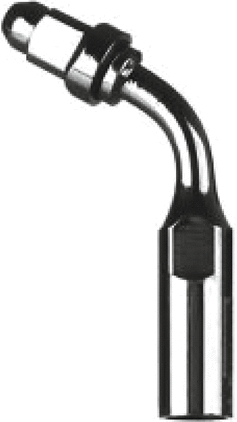
Fig. 10.7
Brasseler file holder E12 (Brasseler)
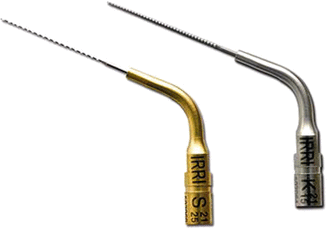
Fig. 10.8
Satelec Acteon Irrisafe™ tips. Note serrated wire with non-cutting sides and irrigation port near attachment hub (Satelec)
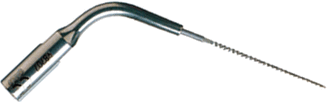
Fig. 10.9
Satelec Sonofile with no irrigation port (Tulsa Dental Products)
Debris and Smear Layer Removal
The effectiveness of PUI/UAI following canal preparation to remove tissue and debris has been extensively studied. In general, PUI/UAI has been reported to be more effective than simple syringe and needle irrigation. As previously stated, Weller et al. [168] was the first to report on the benefits of ultrasonic activation of irrigant following hand instrumentation. They reported that the combination was superior to either technique alone. Goodman et al. [69] and Lev at al. [94] reported that the addition of 3 min of PUI/UAI per canal (using NaOCl as an irrigant) significantly improved the cleanliness of the isthmuses of the mesial roots of mandibular molars in vitro at the 1 and 3 mm levels from the canal apex. Metzler and Montgomery [112] found similar results using 2 min of PUI/UAI. Cameron [36] also reported that canals had less tissue and debris following the use of EDTAC/NaOCl and 1.5 min of PUI/UAI in vitro. These studies were followed up by Haidet et al. [74] and Archer et al. [12] who studied the use of 3 min of PUI/UAI per canal in vivo following hand instrumentation and found that isthmus and canal cleanliness was significantly improved at the levels 1–3 mm from the apex as compared to needle irrigation with NaOCl.
Utilizing ex vivo models with artificially prepared grooves, different preparation tapers, and lateral canals filled with dentin debris, various studies have shown that PUI/UAI improved debris removal from the hard-to-reach areas. Looking at the influence of the size of the canal preparation on cleaning with PUI/UAI, Lee et al. [92, 93] and van der Sluis et al. [162] reported that the greater the taper of the canal, the more debris that is removed with the PUI/UAI file. Rödig et al. [129, 130], however, found that apical size had no impact on canal cleanliness when utilizing PUI/UAI. This result contradicted findings that larger apical preparations improved the efficacy of NaOCl [79]. In another study, van der Sluis [163] reported that a smooth wire (such as a finger plugger placed in a file holder) could remove debris as well as a file design. This supported the previous work of Cameron [34] and Goodman et al. [69]. Jiang et al. [84] reported that the direction the ultrasonic file oscillated may affect cleaning. They stated that improved results were achieved when the vibration was directed at the site of a groove to be cleaned. In terms of irrigant penetration, several studies have looked at the ability of PUI/UAI to improve the dispersement of an irrigant into lateral canals. De Gregorio et al. [55] reported that irrigant penetrated artificially made lateral canals much better when PUI/UAI was utilized than needle irrigation or negative pressure irrigation. Spoorthy et al. [148] reported similar results. Al-Jadaa et al. [8] reported similar results (improved debris removal from artificial lateral canals) between PUI/UAI and needle irrigation when controlling for the increase in temperature of the NaOCl irrigant (approximately 30 ° C) caused by the ultrasonic activation.
Clinically, these studies can be translated into improved canal cleanliness in the areas generally untouched by hand and/or rotary files, i.e., isthmuses, lateral canals, canal fins, and cul-de-sacs. In vivo research has indicated that isthmuses and canals are more thoroughly cleaned when PUI/UAI is utilized following canal preparation [12, 74, 112]. Empirically, this increased ability to remove debris and tissue should lead to improved clinical outcomes. An initial study by Liang et al. [95], evaluating 86 patients 10–19 months after root canal treatment, showed an improvement in the reduction and resolution of apical pathosis following the use of PUI/UAI compared to needle irrigation. However, the difference was not found to be significant. More research with longer follow-up times is needed.
In terms of smear layer removal, results have varied with slightly more studies indicating that PUI/UAI helps remove smear layer. These varied results may be due to the use of different types and concentrations of irrigants. When NaOCl was utilized alone, studies have reported almost complete smear layer removal from various levels of the root canal [7, 33, 34, 35, 81, 159]. These studies, again, utilized various concentrations of NaOCl, ranging from 0.5 to 12 %, and different exposure times to the ultrasonic energy (10 s to 5 min). When NaOCl was combined with EDTA, the research has shown a marked improvement in smear layer removal [11, 20, 66, 90]. Several studies, however, did not find PUI/UAI to be very effective in removing smear layer even when NaOCl and EDTA were utilized [1, 42, 44, 134, 156]. The use of water as an irrigant has been reported not to enhance smear layer removal with the addition of PUI/UAI ([33, 34, 75, 159]). This would indicate that the cavitation and acoustic streaming effects alone cannot account for smear layer removal. The difficulty in studying smear layer removal is that it relies on the assessment of SEM images. Only very small portions of a large area of canal wall are evaluated and, often, different conditions can appear on the same image. This makes grading of the images difficult and potentially unreliable depending on the evaluators and the number of images evaluated.
The amount of irrigant, delivery method, and delivery time of irrigants has also been evaluated. Intermittent flushing is a more popular method as compared to external continuous flushing for PUI/UAI. A new method for continuous irrigation utilizing an ultrasonically activated needle was developed and will be discussed later in the chapter. The intermittent flushing process encompasses the use of an irrigating needle/syringe which is utilized to initially fill the root canal and access opening with irrigant and then replenish the irrigant after applications of ultrasonic energy within the canal. This technique is more time consuming due to the stop-and-go process. The need to replenish the irrigant is due to the fact that dentin debris, tissue, bacteria, and biofilm saturate the irrigating solution and increase the viscosity of the solution to the point where no ultrasonic activity may occur in the solution. This effect was reported by Weller [168] and Moorer and Wesselink [115]. Research has shown that refreshing NaOCl during PUI/UAI increases the reaction of NaOCl [98, 161] and improves cleaning of canals. These studies also indicated that an increase in the time of exposure of the canals to PUI/UAI improved cleanliness in ex vivo models.
Continuous flushing of irrigant, as achieved by utilizing the Irrisafe™ tips with its irrigation ports, requires a delivery system that is able to direct irrigant into the tooth and allow for replacement of saturated or contaminated irrigant. Ideally the irrigant replacement should occur to the level of root canal apex. Also, the formation of aerosol as the irrigant contacts the coronal aspect of the ultrasonic file may lead to patient exposure to the NaOCl beyond the rubber dam or by inhalation. Unfortunately research has shown that with this type of system the time of exposure plays a more critical factor since extra time is needed to completely flush the canals in a rather uncontrolled manner [64, 121]. Lev et al. [94] reported that, in terms of cleaning, 1 min of PUI/UAI per canal was equivalent to 3 min per canal for canal cleanliness, but that 3 min provided cleaner isthmuses when utilizing a continual flushing system. Further research into the effect of time is needed when more standard PUI/UAI techniques are developed. The size of the endodontic access opening may also play a factor in the ability of the irrigant to reach the canal. However, no research has looked at this.
Studies looking at the use of PUI/UAI to remove either calcium hydroxide or other paste fillers from root canals have given mixed results. Complete removal of a medicament is necessary since there is a potential to prevent sealing of the canal due to interference with the filling material by the remaining paste [47, 77, 102]. The addition of PUI/UAI to remove calcium hydroxide and Ledermix was found to improve overall removal, but did not assure complete removal of all material [131]. Wisemann et al. [169] reported similar results. Capar et al. [37] reported that PUI/UAI removed significantly more calcium hydroxide from artificial grooves in the apical third of the root canal as compared to needle irrigation. Complete removal of the paste was not achieved.
The impact of canal curvature on the effectiveness of PUI/UAI has also been reported. Significantly improved cleaning of canals and isthmuses occurred at the apical 5 mm in curved canals versus needle irrigation [69, 82, 112, 135]. Malki et al. [100] report that the flow of irrigant beyond the ultrasonic file tip was not affected by curvature of the canal. Ahmad et al. [5] and Lumley et al. [96] reported improved efficacy when pre-bent files were utilized for PUI/UAI. Amato et al. [10] reported better cleaning of artificially made lateral canals in teeth with PUI/UAI in both straight and curved canals as compared to needle irrigation. However, better cleaning was observed in the straight canals. This could be due to the fact that the ultrasonic file was placed within 1 mm of the apex and contacted the inner wall of the canal at the curvature and the outer wall near the apex therefore leading to diminished or restricted ultrasonic activation of the irrigant. Al-Jadaa et al. [9] reported similar results when comparing the use of straight, pre-bent, and NiTi ultrasonic files placed within 1 mm of the apex of straight and curved canals. In this study, the use of the NiTi file resulted in better debris removal and less transportation versus the straight and precurved stainless steel files/wires.
Bacteria/Biofilm Removal
The removal or reduction in the number of bacteria within the root canal system is one of the primary goals of endodontic therapy. The utilization of ultrasonically activated irrigation to achieve this goal has been researched. A large number of studies have reported a significant reduction in the number of bacteria (as measured by colony forming units – CFU’s) following the use of PUI/UAI [6, 16, 32, 60, 81, 103, 146, 147, 158] when compared to needle irrigation. Only one study failed to show an improvement in CFU reduction [143].
The above studies concentrated on the reduction of free bacteria (planktonic) and not the removal of biofilm. The impact of PUI/UAI on removing biofilms has also been evaluated, but to a lesser extent. Bhuva et al. [17] reported no improvement in removal when utilizing an artificially produced biofilm of E. faecalis. Shen et al. [140] reported an increase in killing of artificial biofilm when PUI/UAI was utilized with chlorhexidine on dentin discs. Case et al. [40] reported similar results when testing ozone – PUI/UAI helped reduce E. faecalis biofilm. Gründling et al. [71] reported that PUI/UAI helped reduce E. faecalis biofilm only when NaOCl was used as an irrigant. Joyce et al. [86] looked at the mechanism of action of ultrasonics on biofilm and stated that PUI/UAI caused deagglomeration of the biofilm via the cavitation effect.
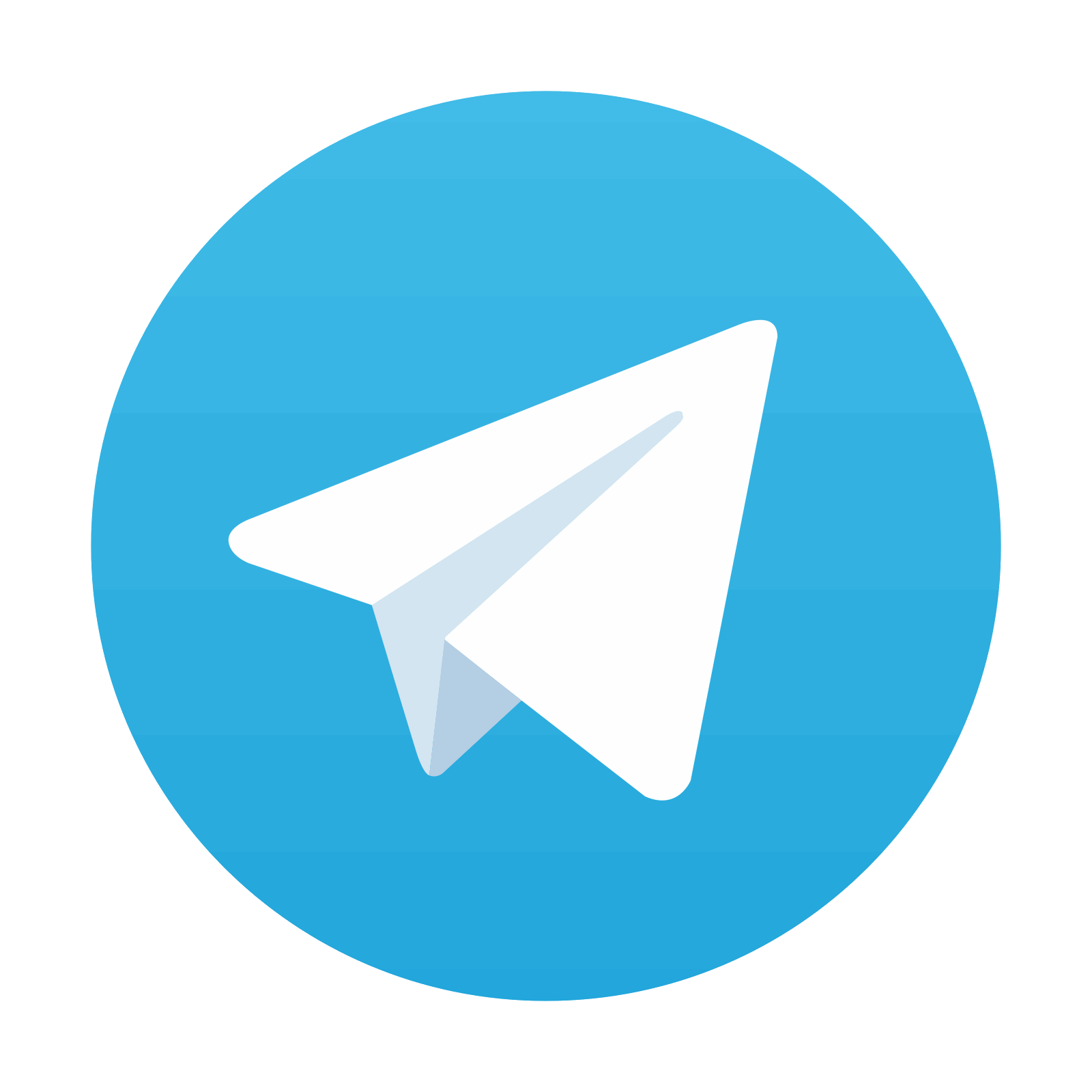
Stay updated, free dental videos. Join our Telegram channel

VIDEdental - Online dental courses
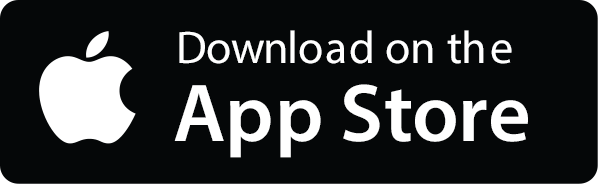
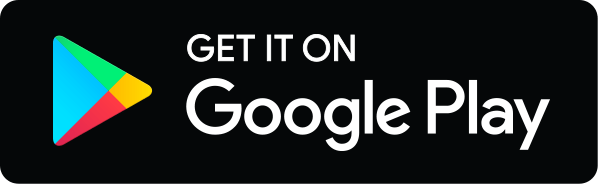