Fig. 12.1
The major salivary glands: (a) Anatomy: The parotid gland lies under the ear and behind the back of the mandible. The duct excretes its contents bilaterally into the buccal mucosa opposite the second upper molars. The sublingual gland lies beneath the tongue and the submandibular gland curls around the lower inside region of the mandible, mostly forward of the angle. The ducts from these two glands release their secretions close together bilaterally beneath the tongue. (b) Composition: The salivary glands are exocrine glands that are composed of serous cells around a single acinus as in a minor gland (1), or group of acini sharing a common collection duct as in a major gland (2), or mucus cells around a single tubule (3), or group of tubules (similar to acini except that cells around the collection duct are in a tubular arrangement; not shown). (a: Figure from Dorland’s Illustrated Medical Dictionary. 31st ed. Elsevier Saunders: 2007, p 790. Figure, “Salivary Glands,” under “Glands” Online at http://medicaldictionary.thefreedictionary.com/_/viewer.aspx?path=dorland&name=gland_salivary.jpg; b: Figure from http://www.siumed.edu/∼dking2/erg/glands.htm#acini)
12.1.1 Whole Saliva: Collection and Composition
Whole saliva is collected by asking volunteers to spit into an ice-cooled vial. If necessary, the flow is stimulated by chewing paraffin wax or washed elastic bands. Pure parotid gland secretions are collected using a Lashley Cup, a small plastic cup held against the duct orifice inside the cheek by a vacuum. The secretion drains into a vial through a tube that fits into a hole in the cup. The flow is stimulated by sucking a sour lemon drop or by applying a drop of sodium citrate to the tongue. The collection of pure submandibular, sublingual, or minor gland secretions requires individually designed equipment.
Whole saliva is a dilute, viscous solution containing electrolytes, proteins, and epithelial cells from the oral mucosa. The major electrolytes are sodium chloride and sodium bicarbonate. Mucin proteins mediate the viscosity, saliva’s most obvious characteristic. Mucins are proteoglycans with many short, negatively charged side-chains, and they are made only by mucous cells: 5-15% of proteins from the submandibular gland, 10-30% of proteins from the sublingual gland, and virtually all the proteins from minor mucous glands. Besides mucins, the major proteins in saliva are α-amylase (α1,4 glucan endohydrolase) and proline-rich proteins . The salivary glands secrete α-amylase into blood as well as saliva. Salivary α-amylase is encoded by a single gene (Amyl) on chromosome 1, but traces of enzymes in saliva modify it, so that a mixture of proteins of similar size and charge (α-amylase isozyme families) appears on gel electrophoresis. The proline-rich proteins are encoded as one of two or three different alleles on each of six adjacent genes on chromosome 12 and also undergo proteolysis in saliva. They therefore give rise to an extremely complex mixture of small polypeptides on gel electrophoresis. Mucins are encoded by MUC5B on chromosome 11 and MUC7 on chromosome 4.
In an individual maintaining oral hygiene, the total protein content of saliva is ∼1.6 mg/mL, much less than in blood plasma. About half the proteins are amylase, 40-45% are proline-rich proteins, and 5-10% are mucins. Stimulated saliva has 20% less protein, but up to a tenfold greater sodium chloride content and up to double the sodium bicarbonate content. Bicarbonate is produced by the same intracellular carbonic anhydrase in acinar cells as in osteoclasts and red blood cells (Chap. 10). The greater sodium bicarbonate content of stimulated saliva makes its pH more alkaline (7.4–7.8) than unstimulated saliva (6.8–7.2).
The salivary glands also secrete urea, which some oral bacteria convert to ammonia and carbon dioxide with an enzyme, urease. The greater content of ammonia results in the oral cavity being better buffered to acids and better protection from caries (Chap. 15, Sect. 3). Calcium and phosphate are also present in saliva at supersaturating concentrations but do not precipitate due to protein chelation. Whole saliva also contains small amounts of various other proteins: proteases, protease inhibitors (cystatins), type IV carbonic anhydrase, statherin, histatins, lysozyme, salivary agglutinin, and immunoglobulin A.
Finally, the salivary glands concentrate sodium nitrate from fruit and vegetables. The sodium nitrate is absorbed into the blood plasma and concentrated 10 – 20 fold by salivary gland cells before secretion. The nitrate in saliva is reduced to sodium nitrite by Eikenella corrodens, a common commensal bacterium in human saliva (Sect. 12.1.5). Sodium nitrite is an important vasodilator, but it cannot cross healthy tissues.
12.1.2 Functions of the Salivary Components
If a major salivary gland is lost from trauma or disease, or if nasal allergies or sinus infections cause persistent mouth-breathing, or if tobacco smoking persists, the oral cavity becomes dry (xerostomia). The oral mucosa and teeth become covered with bacteria and dental caries and periodontal disease become difficult to control. The functions of whole saliva are (1) lubricating the oral tissues and food particles; (2) promoting the clearance of food particles; (3) protecting the oral mucosa and teeth from excessive bacterial colonization; (4) forming an acquired pellicle that protects teeth surfaces from dissolution or over-accretion; and (5) stabilizing teeth surfaces from bacterial acid dissolution. The first three functions are performed mainly by the mucin and water content, aided by amylase and immunity proteins described later, the fourth is associated with other secreted salivary proteins, statherin, and the proline rich proteins, and the last is caused by buffers in saliva, mostly sodium bicarbonate (Table 12.1).
Table 12.1
Functions of saliva effector molecules
Functions
|
Effector molecules
|
---|---|
Lubricate oral mucosa and food particles and inhibit bacterial colonization
|
Mucins
|
Facilitates clearance and inhibits bacterial colonization
|
Water (dilute secretion)
|
Digests starch in food particles, promoting bolus cohesion
|
Amylase
|
Stabilize tooth surface
|
Sodium bicarbonate, carbonic anhydrase, and statherin
|
Form acquired enamel pellicle
|
Proteins
|
Innate immunity that inhibits bacterial growth
|
Peroxidases, histatins, lysozyme, and lactoferrin
|
Innate immunity that enhances bacterial clearance
|
Salivary agglutinin and mucin
|
Acquired immunity that enhances bacterial clearance
|
Secretory immunoglobulin (sIgA)
|
The stability of the tooth surface to spontaneous dissolution and accretion is primarily mediated by statherin, a 43 amino acid polypeptide encoded by a single gene (Stath) on chromosome 4. Its two N-terminal serine residues spontaneously become phosphorylated in saliva, and they attach statherin tightly to exposed tooth enamel, preventing dissolution or calcium phosphate accretion. Nevertheless, all proteins in whole saliva adhere to some extent to enamel, forming an acquired pellicle that replaces abraded enamel cuticle (Chap. 9). Differences in amount or composition of the proteins in whole saliva cause differences in acquired pellicle composition.
Carbonic anhydrase and sodium bicarbonate together neutralize the acids produced by bacterial metabolism of dietary carbohydrate (Fig. 12.2). When salivary carbonic anhydrase is swallowed, it adheres to the mucosal surface of the stomach where it remains active and forms carbonic acid from sodium bicarbonate in the gastric mucosa. A lack of salivary carbonic anhydrase causes acid to remain longer in the stomach, contributing to peptic disease in addition to dental caries (Sect. 15.3.3).
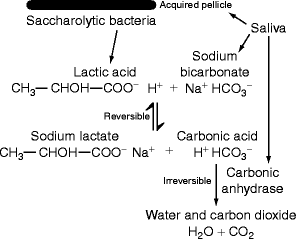
Fig. 12.2
Neutralization of bacterial acids by salivary carbonic anhydrase: Saliva contains proteins that form the acquired pellicle to which saccharolytic bacteria attach and grow anaerobically using dietary carbohydrate as substrate. Lactic acid is produced and reacts reversibly with salivary sodium bicarbonate to make sodium lactate and carbonic acid. Traces of salivary carbonic anhydrase in saliva contribute to the acquired pellicle and also remain in solution. The enzyme converts carbonic acid to water and carbon dioxide. This step is irreversible because carbon dioxide is lost to the environment whenever the mouth is opened. Thus, lactic acid is transformed into its salt, sodium lactate. (Original Figure)
12.1.3 Innate and Acquired Immune Proteins in Saliva
Innate immunity in saliva is mediated by enzymes, peroxidase, lactoferrin and lysozyme, by small peptides histatins and proline-rich proteins, and by two proteins, salivary agglutinin and mucin MG2. All are secreted, but the enzymes may be added independently from leukocytes at the gingival sulcus (Sects. 13.2.3. and 13.2.4). The peroxidase reaction is discussed Chap. 16, Fig. 16.8b. In saliva the cofactor (glutathione) is absent, and the electron-deficient oxygen species released by enzyme action convert chloride (Cl‑1) and thiocyanate (SCN-1) ions to hypochlorite (OCl‑1) and hypothiocyanate (OSCN-1) ions which diffuse into bacteria and inhibit their growth or kill them directly. Lactoferrin is encoded by chromosome 3 and secreted as an aggregate of four identical polypeptides surrounding two ferric (Fe3+) ions. It is electron deficient like hypochlorite or hypothiocyanate and retards the colonization of Gram-negative bacteria and fungi on the oral mucosa. Lysozyme is a glycoside hydrolase encoded by Lyz on chromosome 12. It lyses bacteria by hydrolyzing a glycan bond of peptidoglycan on the surface of Gram-positive bacteria (Sect. 1.4.1) and is distantly related to amylase (GH family 22, Sect. 12.5.1.). Gram-negative bacteria are not readily hydrolyzed by this enzyme because a second, outer membrane covers the peptidoglycan (Sect. 1.4.1).
Histatins are positively charged small polypeptides encoded by two genes on chromosome 2, His1 and His2. They bind to the oral mucosa and teeth where they inhibit bacterial growth. The proline-rich proteins (PRPs) may be positively or negatively charged. The latter, the acidic PRPs, bind to teeth where they likely alter dental biofilm development (Sect. 12.6.1). Salivary agglutinin binds to various oral bacteria and causes them to be swallowed instead of forming biofilms. Mucin MG2 (Sect. 12.3.1) clears fungi similarly.
Acquired immunity is mediated by lymphocytes directly or by their secreting immunglobulins (Ig). An acquired immune response in the oral cavity is induced by antigens, usually a foreign molecule from a bacterium or fungus that colonizes the oral cavity from the environment. High molecular weight surface components of these organisms activate gut-associated lymphoid tracts (GALT), which include the tonsils of the pharynx. Lymphoid cells within these tissues are induced to secrete IgA into the surrounding blood plasma, which passes into saliva and other secretions. In saliva, secreted IgA (sIgA) binds to the antigen that induced its production. The IgA–antigen complex is swallowed and digested in the stomach or small intestine.
12.1.4 Poor Oral Hygiene Adds Bacteria and host leukocyte Products to Saliva
The bacteria in a healthy oral cavity, the commensal oral microbiota, protect it from disease by repelling disease-causing (pathogenic) bacteria. A weak point in this protection is adherence of the commensal microbiota to the acquired pellicle. Microbial biofilms attach to the teeth, and differences in salivary protein composition cause differences in which bacteria make up the commensal oral microbiota. This microbiota extends into the gingival sulcus and induces gingival crevicular fluid (GCF), an inflammatory exudate derived from blood plasma containing activated leukocytes (Sect. 13.1.2). Thus, differences in salivary composition influence how much GCF is produced. The GCF is a better substrate for microbial growth than saliva, and the presence of commensal biofilms on teeth surfaces provides a more favorable environment for the colonization by pathogenic bacteria that are otherwise present in only trace amounts. These bacteria are gram negative and asaccharolytic. They release proteases and cell surface components into the oral cavity along with asaccharolytic metabolic end products, ammonia, amines, and sulfides (Sect. 1.3.1), which give mixed whole saliva (spit) an offensive odor.
Summary
Whole saliva (spit) is a dilute, viscous solution of proteins and shed epithelial cells. The major electrolytes are sodium, chloride, and bicarbonate. Calcium and phosphate are present at a supersaturated concentration. Viscosity is due to mucins, proteoglycans with numerous short glycan chains that lubricate the oral cavity, hold a bolus of chewed food together, and reduce bacterial adherence to teeth. Besides mucins, the major proteins secreted in saliva are amylase and proline-rich proteins. The major electrolytes, sodium chloride and sodium bicarbonate, increase with stimulation of salivary flow, but the protein content decreases. All proteins in whole saliva adhere to some extent to enamel, forming an acquired pellicle that replaces abraded enamel cuticle. Differences in the amount or composition of whole saliva may result in individual, saliva-associated differences in dental caries. Small amounts of carbonic anhydrase protect the teeth from bacterial acid dissolution. Small amounts of statherin are secreted to protect the teeth from accreting salivary calcium phosphate. Various innate and acquired immunity proteins protect from bacterial infections. Innate immunity in secreted saliva is mediated by enzymes, peroxidase, lactoferrin and lysozyme, by small peptides, histatins and proline-rich proteins, and by two proteins, salivary agglutinin and mucin MG2. Acquired immunity is mediated by IgA. A bacterial biofilm (plaque) develops on the acquired pellicle where it induces the exudation of gingival crevicular fluid from underlying capillaries. Asaccharolytic bacteria grow on this fluid and add malodorous metabolic end products to whole saliva.
12.1.5 Physiology and Biochemistry of Saliva Secretion
In the cytosol, polypeptides destined for secretion in saliva are steered to the cytosolic side of the rough endoplasmic reticulum (ER) by the amino acid sequence of their N-terminal domains. This domain interacts with a signal recognition particle (SRP) in the cytosol and stops translation until the ribosomal/SRP complex moves to a ribosomal receptor. Placement on the receptor releases the SRP particle and allows the ribosome to resume translation. Polypeptide synthesis is completed in the ER lumen instead of the cytosol, and moved to the smooth ER, where certain asparagine amide residues are glycosylated (N-linked glycosylation). The glycan is preformed attached to dolichol diphosphate in the cytosol and transferred to the asparagine amide acceptor after switching from cytosolic to luminal face of the ER (Fig. 12.3, upper third). Protein-rich vesicles form and are transported to the cis face of the Golgi (Fig. 12.3, lower two-thirds). As the proteins pass through the Golgi, glycosidases and glycan synthetases greatly alter the N-linked glycans and attach other glycans to the −OH group of certain serine and threonine residues in the protein (O-linked glycosylation, Fig. 12.3, lower two-thirds).
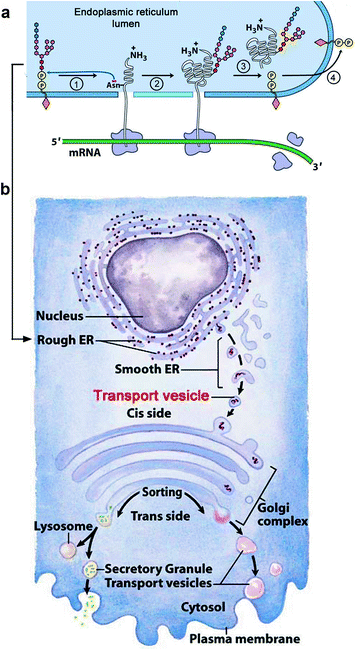
Fig. 12.3
How salivary proteins are secreted (a): N-linked glycans are synthesized and attached to dolichol diphosphate (orange diamond) in the cytosol. The completed glycan is translocated to the lumen of the endoplasmic reticulum (blue). The glycan attached to dolichol diphosphate by its C1 (anomeric or reducing OH group) is N-acetyl glucosamine (GlcNAc; small red hexagon). The completed glycan also contains mannose and glucose residues (small pink and blue hexagons). (1) The glycan (oligopolysaccharide) is transferred to an asparagine residue of the growing peptide also in the lumen of the rough endoplasmic reticulum (ER). (2) Synthesis of the glycan-attached polypeptide amino acid sequence is completed. (3) The free dolichol diphosphate translocates back into the cytosol where a phosphatase removes its outer phosphate residue. (4) In the cytosol, a molecule of UDP-GlcNAc reattaches to give dolichol diphosphate GlcNAc (not shown) onto which an identical set of monosaccharides are added to give another glycan to be transferred as shown in 1. (b): The released glycan-attached polypeptide buds off into vesicles (smooth endoplasmic reticulum, ER) that discharge their contents into the cis-Golgi where the glycans are processed by removing most of the mannose and adding fucose, sialic acid, galactose, and other glycan residues. Mucin polypeptides have a sequence that activates an addition of glycans beginning with N-acetyl galactosamine (GalNAc) to serine and threonine –OH groups in the Golgi. In the trans-Golgi, the contents are either moved to lysosomal vesicles or secreted. (Modified from Figs. 27.34 and 27.35 in Lehninger Principles of Biochemistry. D.L. Nelson & M.M. Cox, 4th Ed. 2005. W.H. Freeman & Co., NY)
Vesicles containing proteins destined for intracellular use in lysosomes or outer membranes bud off from the trans face of the Golgi. Depending on their contents, some of the vesicles are diverted to nonsecretory vesicles by the presence of phosphomannose residues on their N-linked glycans or by possessing domains rich in hydrophobic amino acids. At the apical surface of salivary acini, the remaining vesicles accumulate as secretory vesicles. These vesicles become surrounded by myofibrils, which move the secretory vesicles to the cell membrane where they fuse and expel the saliva secretion into a small duct. The odor or taste of food provides a neuronal stimulus to the gland’s myofibrils, and this stimulates salivary secretion.
Water is secreted separately. Neurotransmitters activate a 28-kDa integral membrane protein, aquaporin-5 (AQP5), one of 13 aquaporins in mammals. AQP5 is localized in lipid rafts in the plasma membrane of salivary cells and translocates to the apical plasma membrane where the secretory vesicles have accumulated. The extent of secretory vesicle and AQP5 translocation and fusion with the apical membrane depends on the extent of stimulation by cholinergic (acetylcholine) receptors, or alpha-1 (adrenergic) receptors coupled to G proteins and phospholipase C. Cholinergic receptors activate Ca2+ mobilizing receptors and signal both secretory vesicles (protein) and water secretion from salivary glands. Adrenergic stimulation of cyclic adenosine monophosphate (cAMP-dependent protein kinase system) increases secretory vesicle exocytosis immediately and the Ca2+ mobilizing receptors for water secretion afterward. The reason why these two neuronal activation responses are linked is not known. Eating and smelling food, or even just thinking of these things, transports water faster than the secretory vesicle contents; stimulated saliva has more water and less protein.
Summary
Salivary proteins are secreted like other proteins. Polypeptides destined for secretion are relocated by their N-terminal sequence to a ribosomal receptor on the cytosolic side of the endoplasmic reticular (ER) membrane. The polypeptides enter the ER lumen and move from rough to smooth ER where asparagine amide residues are glycosylated with a preformed glycan (N-linked glycosylation). Vesicles bud off and are transported to the Golgi where glycosidases and glycan synthetases modify the N-linked glycans and attach glycans to the -OH groups of serine and threonine residues (O-linked glycosylation). Water is separately secreted through a 28-kDa integral membrane protein, aquaporin (AQP5). AQP5 translocates from lipid rafts in the plasma membrane to the apical membrane where the secretory vesicles have accumulated and become surrounded by myofibrils. Smelling or ingesting food stimulates saliva, making it more dilute. The smell or ingestion activates cholinergic and adrenergic receptors at autonomic nerve endings around the salivary acini, causing faster AQP5 translocation through the cell membrane. Water is thus transported faster and independently of secretory vesicle contents, which are more forcibly expelled by the stronger myofibril contractions.
12.1.6 Salivary Mucin Composition
Mucin proteins account for the high viscosity of saliva in the oral cavity and, like the connective tissue proteoglycans, have many serine and threonine-linked glycan residues that absorb a large volume of water. A sulfate (-SO4)−1 group is also attached in the Golgi to the -OH group of certain glycan residues following synthesis of the chain, usually galactose, N-acetylgalactosamine or N-acetylglucosamine. Sulfation also accounts for the negative charge of the connective tissue proteoglycans (dermatan sulfate, chondroitin sulfate, etc.). Sialic acid residues terminate some of the short mucin glycan chains and contribute also to the strong net negative charge of salivary and other mucins. The connective tissue proteoglycan chains are longer and fewer and use uronate instead of sialate as the negatively charged glycan.
Human submandibular and sublingual glands secrete two mucin glycoproteins, MG1 and MG2. MG1 is a very large polypeptide composed of more than 5,500 amino acid residues (mol wt ∼590 kDa). Its N- and C-terminal regions each contain two N-glycosylated sites (Fig. 12.4a
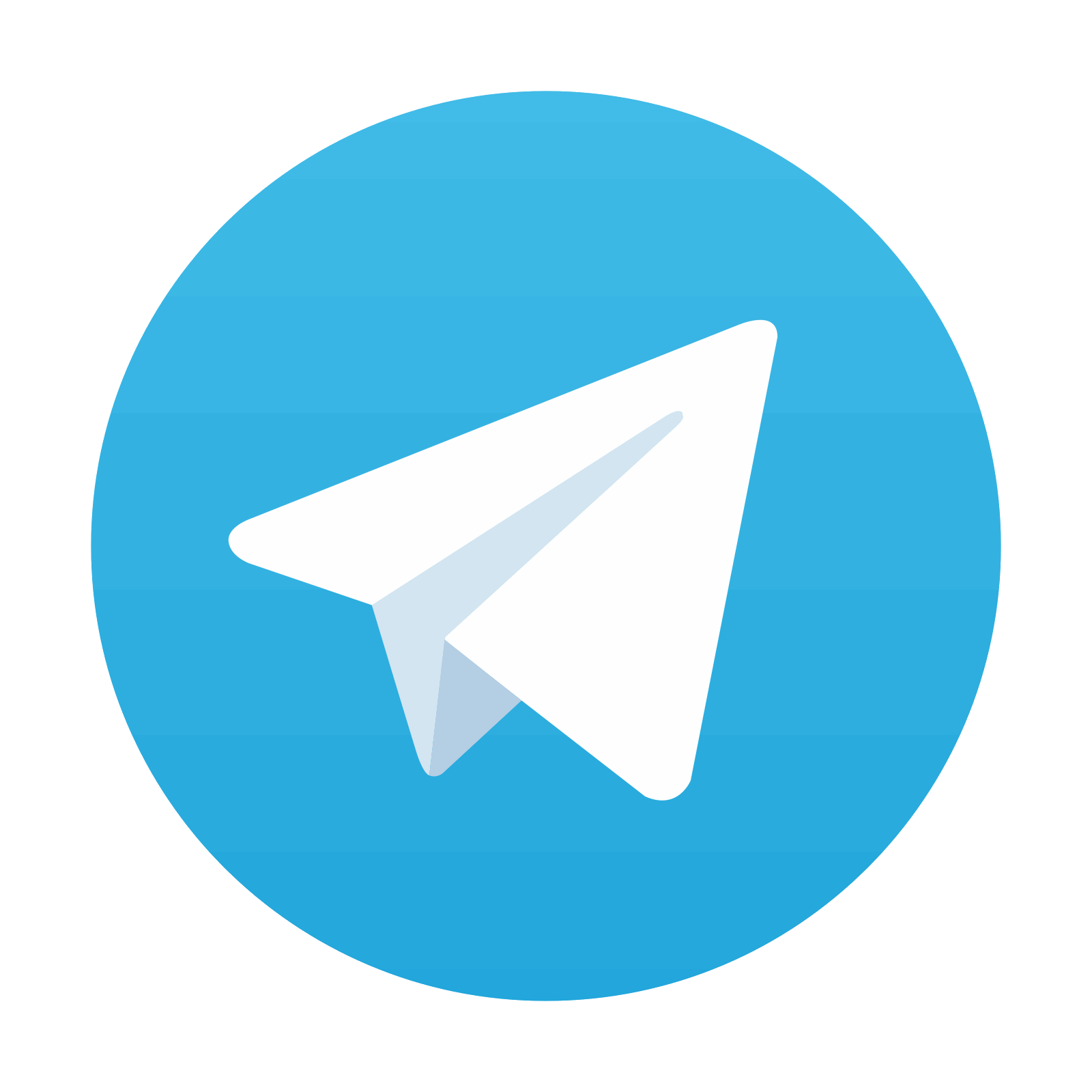
Stay updated, free dental videos. Join our Telegram channel

VIDEdental - Online dental courses
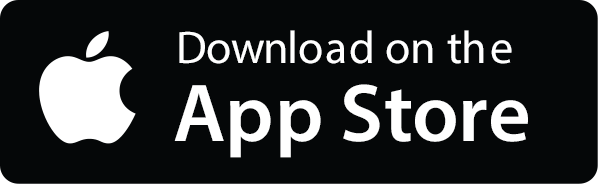
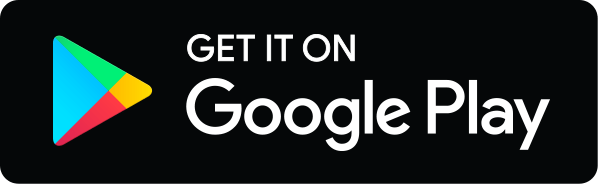