Abstract
Objectives
Firstly, to assess light output, from a representative range of dental light curing units (LCUs), using a new portable spectrometer based instrument (checkMARC™) compared with a “gold standard” method. Secondly, to assess possible inconsistency between light output measurements using three different laboratory-grade thermopile instruments.
Methods
The output of four blue-dental LCUs and four polywave blue-and-violet-LCUs was measured with two spectrometer-based systems: a portable spectrometer instrument and a benchtop Integrating Sphere fiber-coupled spectrometer system. Power output was also recorded with three thermopiles according to ISO 10650-2. Beam profile images were recorded of LCU output to assess for spatial and spectral beam uniformity.
Results
Power recorded with the portable spectrometer instrument closely matched the ‘gold standard’ Integrating Sphere apparatus calibrated according to International Standards. Radiant exitance for the eight LCUs differed significantly between the three thermopiles. Light source to thermopile sensor distance influenced recorded power significantly ( p < 0.05), indicating the severe limitations of thermopiles for absolute measurements. Polywave LCU beam profiles demonstrated output spectral heterogeneity.
Significance
Spectrometer-based methods are capable of overcoming the limitations inherent with thermopile-based measurement techniques. Spectrometer based measurements can fulfill the intention of ISO 10650.
1
Introduction
1.1
The challenge of photo-polymerization in dentistry
Effective photo-polymerization is a prerequisite for the long term clinical success and quality of light-activated resin-based composite (RBC) restorations. Amongst the factors which influence the polymerization efficiency of (multi-)methacrylate RBCs, the light curing unit (LCU) is a key extrinsic variable . Whilst quartz tungsten halogen (QTH) LCUs have been the mainstay of dental practice for decades blue light emitting diode (LED) units have replaced them as the most popular type because they are compact, portable, energy efficient and have potentially superior service life .
1.2
The challenges of measuring radiant output of dental LED-LCUs
Unlike point light sources or collimated laser sources, dental LED-LCUs pose unique challenges. Hand-held dental radiometers commonly used for measuring irradiance are inaccurate and may vary within the same model . Results from such ‘dental radiometers’ are influenced by differences in measurement time, spectral sensitivity and mismatch between the instrument sensor aperture and the LCU area .
Attention to detail is required for accurate performance of all radiometric measurements. Meaningful reporting of radiometric data requires appropriate nomenclature and precise physical definitions of key terms such as radiant exitance . The diversity of: (i) LCU types (gun or pen style, corded or cordless, fixed or removable lens cap or fiber optic light guide) and (ii) irradiation protocols available (pulse, soft start and modulated cure) complicate the measurement of their power output, spectral and irradiance characteristics. The following major challenges exist:
- •
The diameter of the LCU optic or exit window may range from <5 mm to >10 mm and there may be non-uniformity of the light beam-profile.
- •
The target surface area (diameter) will vary with different clinical scenarios or laboratory test setup requirements.
- •
The LCU optic – “target” distance can vary in clinical practice from zero to 10 mm and the irradiance declines over this distance.
- •
LED-LCUs may incorporate one or more LED-chips each outputting a different wavelength range.
- •
The irradiation time can vary from 1 s to 20 s or more. Power output varies over time with pulsed or modulated cure protocols.
The available instrumentation for measuring output includes (i) light collection devices (cosine correctors, integrating spheres), (ii) bandpass filters to isolate specific spectral regions, (iii) thermopiles, (iv) spectrometers. The selection of appropriate combinations is challenging for the reasons outlined above. Integrating sphere light collection devices coupled to spectro-radiometers are considered as the gold standard for the optical characterization of dental LCUs in the laboratory. Total radiant power and spectral power as a function of wavelength may be determined. Recently introduced modified integrating sphere instruments (checkMARC™ from Bluelight analytics and the larger BTS256-Hi from Gigahertz-Optik GmbH, Türkenfeld, Germany) make it practical to conduct such measurements in dental practice.
1.3
Consideration of the existing ISO Standard for LED-LCUs
ISO 4049 is concerned with polymer based restorative materials and ISO 10650-2 exists to standardize the requirements and test methods for light curing units (LCUs). ISO 10650-2 outlines a standardized protocol for measuring the output of dental LED-LCUs. It addresses some of the issues mentioned above but fails to deal with others. Because of the relatively slow response-time inherent with thermopile detectors, LCUs with short irradiation times cannot be measured reliably. When ISO 10650-1 was published in 2004 it identified 400–515 nm as the blue region for LCUs. The standard was published before multi-peak or polywave LED-LCUs were marketed, designed to cure materials containing alternative photo-initiators. The cut-off point between violet and blue light may be identified as 425 nm spectrally. The development of high irradiance (>1500 mW/cm 2 ) LED-LCUs with very short irradiation times coupled with the introduction of spatially non-uniform multi-wavelength sources have increased the need to revise these standards for contemporary dental practice.
ISO 10650-2 (2007) specifies measurement of radiant exitance for three discrete wavelength regions using a series of four long-pass filters ( Table 1 ). The ISO 10650-1 source document requires that the entrance aperture of the radiometer be greater than the cross-sectional area of the optic tip (light-guide exit window) and that the edge of the optic tip should be at least 2 mm from the edge of the radiometer entrance aperture so that all radiant emission is measured by the radiometer. The protocol involves sequentially measuring radiant exitance with specified filters and calculating and reporting light transmission in the ranges (a) 190–385 nm, (b) 400–515 nm and (c) above 515 nm. Region (a) includes not only the ultraviolet region but also the near-blue wavelength region of around 380 nm. Unfortunately the 385–400 nm region is unaccounted for, although several dental LED-LCU sources emit considerable radiant energy in this region, since the 385–415 nm bandwidth is important for some alternative photo-initiators. In Section 5.2.2, it specifies that the radiant exitance between 190 and 385 nm should be less than 200 mW/cm 2 . The region above 515 nm reaches approximately to 1100 nm, which is the detection limit of the measurement device specified in the Standard. According to Section 5.2.3, the upper limit for radiant exitance above 515 nm should be not greater than 100 mW/cm 2 . No requirement is given for radiant exitance (or power) in the 400–515 nm wavelength region which is the blue light region for polymerization activation. To fulfill the test requirement, the radiant exitance in the 400–515 nm region must not be less than “the manufacturer’s stated value” when measured in accordance with Section 7.2.
Filter type | Quartz (SQ1) | Schott GG385 | Schott GG400 | Schott OG515 |
---|---|---|---|---|
Transmission | a > 190 nm | b > 385 nm | c > 400 nm | d > 515 nm |
[0,1-2]ISO 10650-2 procedures and corresponding wavelength regions (nm) | a – b | c – d | d | |
190–385 | 400–515 | >515 |
A micrometer, with an accuracy of 0.02 mm, is used to measure the diameter of the optic tip, if it has a circular cross-section, although this may not allow for the presence of any optic cladding. For elliptical cross-section tips the major and minor axes are measured to calculate the optical cross-sectional area. The Standard does not specify LCU-to-detector distance and acknowledges that the results do not represent true radiant exitance or analogously irradiance. Source distance is defined by the radiometer employed and by the optical filter thickness.
1.4
Assessment of alternative spectrometer-based systems for LED-LCUs
The focus of this work was to assess spectrometer-based extra-oral methods for measuring radiant exitance of LED-LCUs. The aim was to see if spectrometer based systems could overcome the limitations inherent in the current ISO 10650-2 test method. A specific aim was to assess how a portable spectrometer instrument (checkMARC™) performed compared to a ‘gold standard’ integrating-sphere based spectrometer method. A secondary objective was to assess how thermopile design impacted on power measurement according to ISO 10650-2.
Hypotheses:
- 1.
Mean power, as measured with the portable spectrometer instrument (checkMARC™), will not vary by more than 5% from the Integrating Sphere/spectrometer ‘gold standard’ reference value for any of the eight representative LED-LCUs.
- 2.
The mean radiant exitance or irradiance values, from each of eight representative LED-LCUs), as determined with the method described in ISO 10650-2 for the wavelength range between 400 nm and 515 nm, using three different laboratory grade thermopiles, will agree between the instruments to within 5%.
2
Materials and methods
Eight contemporary LED-LCUs, representative of the range of different types of unit currently available, were used in this work ( Table 2 ). As shown in the final column of Table 2 , the active or functional optic areas ranged from 0.41 to 1.63 cm 2 . These were determined by analysis of captured images of the end-optics and excluded any optic cladding areas, if incorporated in the optic design. Four of the LCUs were single-peak or monowave blue LED sources and the manufacturers of the other four units (Bluephase G2, Bluephase Style, Smartlite maX and Valo cordless) indicated that their units were broad spectrum and thus were multi-peak or polywave with output in violet as well as blue wavelengths. None of the manufacturers of the above LCUs claimed conformity with ISO 10650-2 for their unit.
Light curing unit (LCU) | Manufacturer | Serial number | Polywave | Stated irradiance (mW/cm 2 ) | Measured active optic area (cm 2 ) |
---|---|---|---|---|---|
Bluephase G2 | Ivoclar Vivadent | 223306 | Y | 1200 ± 10% | 0.646 |
Bluephase Style | Ivoclar Vivadent | 1100001225 | Y | 1100 ± 10% | 0.622 |
SmartLite maX | Dentsply | 003512 | Y | 1200 | 1.626 |
Valo Cordless | Ultradent | C01535 | Y | 1000 | 0.750 |
Elipar S10 | 3M ESPE | 9391120138 | N | 1200 ± 10% | 0.622 |
Flash Max P3 460 4W | CMS dental | 12FMP0072 | N | 5000–6000 | 0.720 |
Radii Plus | SDI | 56497 | N | 1500 | 0.443 |
S.P.E.C. 3 | Coltene/Whaledent | 121110267 | N | 1600 | 0.407 |
Radiant exitance refers to the magnitude of light emitted from unit area of the source, whereas irradiance refers to the radiant power received by a surface. The latter is more clinically relevant given that it refers to the radiant power that impacts the material surface. Although both quantities have the same units: mW/cm 2 , they can only be considered to be numerically equal at zero distance, when the emitted light is received directly from the LCU by the target material or detector. At non-zero distances, the irradiance from most LED devices attenuates with target distance as the light spreads out over a wider area.
Some of the units had more than one output (or mode) setting, but the selected (standard) mode and the respective irradiances, stated by the manufacturer of each unit, are tabulated. Wherever possible, LED-LCUs were measured in the highest power mode which allowed 10 s continuous irradiation to comply with ISO 10650-2. In the case of one LCU (Flashmax P3), where the longest continuous irradiation time was 3 s, the output was measured after 3 s activation, where meter response-time allowed.
2.1
Spectrometer measurements
Two spectrometer systems, each calibrated to international standards, were compared, namely:
- (a)
a ‘gold standard’ integrating sphere system . This consisted of a laboratory-grade integrating sphere (IS: Labsphere sn: 1229110001), 15 cm in diameter, with a 19 mm entrance port linked to a calibrated spectro-radiometer (Ocean Optics USB 4000 sn: USB4F08680). This was used to register total power, within the 360–540 nm range, and spectra for each LED-LCU ( n = 5 measurements per LCU).
- (b)
a portable calibrated instrument (checkMARC™; Bluelight Analytics, Inc., Halifax, NS B3L 4G4, Canada). This featured a 16 mm diameter active light collection area, incorporating a sealed Teflon diffuser window. This allowed light transmission into the light collection chamber attached via a fiber optic connector to an inbuilt (STS, Ocean Optics) spectrometer assembly. The device was calibrated using a NIST reference light source (HL2000, Ocean Optics) and spectral radiant power measurements were taken using SpectraSuite software (Ocean Optics). The total spectral radiant power output from each LCU was divided by the cross sectional active area (cm 2 ) of each light tip to calculate the average irradiance.
The calibrated checkMARC™ radiometer allowed irradiance to be determined for any currently available dental LCU, irrespective of its exit-window dimensions. Irradiance and spectra for each LED-LCU were recorded at zero source-to-sensor distance ( n = 5 measurements per LCU).
2.2
Thermopile measurements
Using three types of thermopile detector, power measurements were also undertaken according to ISO 10650-2. These thermopiles were: (a) PM10-19C (sn: 1168344); (b) PM10 (sn: 0789J11R), Coherent Inc., and (c) S310C-SP4 (sn: 1208132; ThorLabs). They had similar sensor diameters (19–20 mm) but had different well depths: 6, 11.5 and 16 mm respectively. The Coherent PM10-19C had a USB connection that housed the signal processing and microelectronics, eliminating the need for a separate meter.
Circular ISO-specified Schott long-wavelength pass (LWP) filters of diameter 25 mm (SQ1, GG385, GG400, OG515; thickness 3.0 ± 0.1 mm) were placed sequentially (as per Table 1 ) above each thermopile, to take power readings as per ISO 10650-2. With these in position, the LCU optic-to-sensor distances were 9 mm for the PM10-19C, 14.5 mm for the PM10 and 19 mm for the S310C-SP4. Power outputs recorded with the LWP filters were used to calculate radiant exitance in the region 190–400 nm, to determine the output below 400 nm for the LCUs compared with the blue light output in the 400–515 nm range, as specified in the Standard.
Additional measurements, beyond those specified in ISO 10650-2, were undertaken with the thermopiles to understand: (i) how the presence of the LWP filters and (ii) light source-to-sensor distance influenced the power measured for all LCUs and thermopile models. Thus the measurements were repeated at corresponding light source-to-sensor distances for each thermopile/LCU combination, but without any interposed filters. This allowed the influence of the filter on recorded power output to be determined. The light sources were also measured with their exit windows positioned at 1 mm distance from the thermopile sensors when light unit and thermopile design permitted. For this experiment, one of the thermopiles (Thorlabs S310C-SP4) was modified, before recalibration, to incorporate a 19 mm wide recess in the meter housing to allow access for all the LCU optics to 1 mm from the sensor face. This modified sensor was used in conjunction with a PM-200 meter.
2.3
Beam profile measurements
Beam profiling, widely used for characterization of laser beams, has been adapted for dental curing LCUs. The variables considered include performance over clinically relevant distances, spectral inhomogeneity and identification of hot (high irradiance) and cold (low irradiance) areas. Beam profile images were recorded of all the LCUs with the optic rod of each unit placed against the surface of a frosted glass screen target (DG2X2-1500, Thor laboratories, Newark, NJ, USA) and also with the optic at 4 and 10 mm from the target. The method has been described previously . The images allowed an assessment to be made of beam heterogeneity over the active area of the LCU exit window as well as the decrease in beam irradiance with distance onto a central 4 mm diameter target area. Each image was individually calibrated, according to the power recorded for each LCU with the Integrating Sphere, by setting the highest level measured to assume the highest array count in the Beamgage Standard (v.5.6: Ophir Spiricon) software. Spectral irradiance for violet (400–410 nm) and blue (455–465 nm) wavelength regions were recorded and calibrated on separate images with the aid of appropriate narrow (FWHM = 10 nm) bandpass filters. This allowed an assessment to be made of the wavelength distributions for violet and blue wavebands across the exit windows of the LED sources.
2
Materials and methods
Eight contemporary LED-LCUs, representative of the range of different types of unit currently available, were used in this work ( Table 2 ). As shown in the final column of Table 2 , the active or functional optic areas ranged from 0.41 to 1.63 cm 2 . These were determined by analysis of captured images of the end-optics and excluded any optic cladding areas, if incorporated in the optic design. Four of the LCUs were single-peak or monowave blue LED sources and the manufacturers of the other four units (Bluephase G2, Bluephase Style, Smartlite maX and Valo cordless) indicated that their units were broad spectrum and thus were multi-peak or polywave with output in violet as well as blue wavelengths. None of the manufacturers of the above LCUs claimed conformity with ISO 10650-2 for their unit.
Light curing unit (LCU) | Manufacturer | Serial number | Polywave | Stated irradiance (mW/cm 2 ) | Measured active optic area (cm 2 ) |
---|---|---|---|---|---|
Bluephase G2 | Ivoclar Vivadent | 223306 | Y | 1200 ± 10% | 0.646 |
Bluephase Style | Ivoclar Vivadent | 1100001225 | Y | 1100 ± 10% | 0.622 |
SmartLite maX | Dentsply | 003512 | Y | 1200 | 1.626 |
Valo Cordless | Ultradent | C01535 | Y | 1000 | 0.750 |
Elipar S10 | 3M ESPE | 9391120138 | N | 1200 ± 10% | 0.622 |
Flash Max P3 460 4W | CMS dental | 12FMP0072 | N | 5000–6000 | 0.720 |
Radii Plus | SDI | 56497 | N | 1500 | 0.443 |
S.P.E.C. 3 | Coltene/Whaledent | 121110267 | N | 1600 | 0.407 |
Radiant exitance refers to the magnitude of light emitted from unit area of the source, whereas irradiance refers to the radiant power received by a surface. The latter is more clinically relevant given that it refers to the radiant power that impacts the material surface. Although both quantities have the same units: mW/cm 2 , they can only be considered to be numerically equal at zero distance, when the emitted light is received directly from the LCU by the target material or detector. At non-zero distances, the irradiance from most LED devices attenuates with target distance as the light spreads out over a wider area.
Some of the units had more than one output (or mode) setting, but the selected (standard) mode and the respective irradiances, stated by the manufacturer of each unit, are tabulated. Wherever possible, LED-LCUs were measured in the highest power mode which allowed 10 s continuous irradiation to comply with ISO 10650-2. In the case of one LCU (Flashmax P3), where the longest continuous irradiation time was 3 s, the output was measured after 3 s activation, where meter response-time allowed.
2.1
Spectrometer measurements
Two spectrometer systems, each calibrated to international standards, were compared, namely:
- (a)
a ‘gold standard’ integrating sphere system . This consisted of a laboratory-grade integrating sphere (IS: Labsphere sn: 1229110001), 15 cm in diameter, with a 19 mm entrance port linked to a calibrated spectro-radiometer (Ocean Optics USB 4000 sn: USB4F08680). This was used to register total power, within the 360–540 nm range, and spectra for each LED-LCU ( n = 5 measurements per LCU).
- (b)
a portable calibrated instrument (checkMARC™; Bluelight Analytics, Inc., Halifax, NS B3L 4G4, Canada). This featured a 16 mm diameter active light collection area, incorporating a sealed Teflon diffuser window. This allowed light transmission into the light collection chamber attached via a fiber optic connector to an inbuilt (STS, Ocean Optics) spectrometer assembly. The device was calibrated using a NIST reference light source (HL2000, Ocean Optics) and spectral radiant power measurements were taken using SpectraSuite software (Ocean Optics). The total spectral radiant power output from each LCU was divided by the cross sectional active area (cm 2 ) of each light tip to calculate the average irradiance.
The calibrated checkMARC™ radiometer allowed irradiance to be determined for any currently available dental LCU, irrespective of its exit-window dimensions. Irradiance and spectra for each LED-LCU were recorded at zero source-to-sensor distance ( n = 5 measurements per LCU).
2.2
Thermopile measurements
Using three types of thermopile detector, power measurements were also undertaken according to ISO 10650-2. These thermopiles were: (a) PM10-19C (sn: 1168344); (b) PM10 (sn: 0789J11R), Coherent Inc., and (c) S310C-SP4 (sn: 1208132; ThorLabs). They had similar sensor diameters (19–20 mm) but had different well depths: 6, 11.5 and 16 mm respectively. The Coherent PM10-19C had a USB connection that housed the signal processing and microelectronics, eliminating the need for a separate meter.
Circular ISO-specified Schott long-wavelength pass (LWP) filters of diameter 25 mm (SQ1, GG385, GG400, OG515; thickness 3.0 ± 0.1 mm) were placed sequentially (as per Table 1 ) above each thermopile, to take power readings as per ISO 10650-2. With these in position, the LCU optic-to-sensor distances were 9 mm for the PM10-19C, 14.5 mm for the PM10 and 19 mm for the S310C-SP4. Power outputs recorded with the LWP filters were used to calculate radiant exitance in the region 190–400 nm, to determine the output below 400 nm for the LCUs compared with the blue light output in the 400–515 nm range, as specified in the Standard.
Additional measurements, beyond those specified in ISO 10650-2, were undertaken with the thermopiles to understand: (i) how the presence of the LWP filters and (ii) light source-to-sensor distance influenced the power measured for all LCUs and thermopile models. Thus the measurements were repeated at corresponding light source-to-sensor distances for each thermopile/LCU combination, but without any interposed filters. This allowed the influence of the filter on recorded power output to be determined. The light sources were also measured with their exit windows positioned at 1 mm distance from the thermopile sensors when light unit and thermopile design permitted. For this experiment, one of the thermopiles (Thorlabs S310C-SP4) was modified, before recalibration, to incorporate a 19 mm wide recess in the meter housing to allow access for all the LCU optics to 1 mm from the sensor face. This modified sensor was used in conjunction with a PM-200 meter.
2.3
Beam profile measurements
Beam profiling, widely used for characterization of laser beams, has been adapted for dental curing LCUs. The variables considered include performance over clinically relevant distances, spectral inhomogeneity and identification of hot (high irradiance) and cold (low irradiance) areas. Beam profile images were recorded of all the LCUs with the optic rod of each unit placed against the surface of a frosted glass screen target (DG2X2-1500, Thor laboratories, Newark, NJ, USA) and also with the optic at 4 and 10 mm from the target. The method has been described previously . The images allowed an assessment to be made of beam heterogeneity over the active area of the LCU exit window as well as the decrease in beam irradiance with distance onto a central 4 mm diameter target area. Each image was individually calibrated, according to the power recorded for each LCU with the Integrating Sphere, by setting the highest level measured to assume the highest array count in the Beamgage Standard (v.5.6: Ophir Spiricon) software. Spectral irradiance for violet (400–410 nm) and blue (455–465 nm) wavelength regions were recorded and calibrated on separate images with the aid of appropriate narrow (FWHM = 10 nm) bandpass filters. This allowed an assessment to be made of the wavelength distributions for violet and blue wavebands across the exit windows of the LED sources.
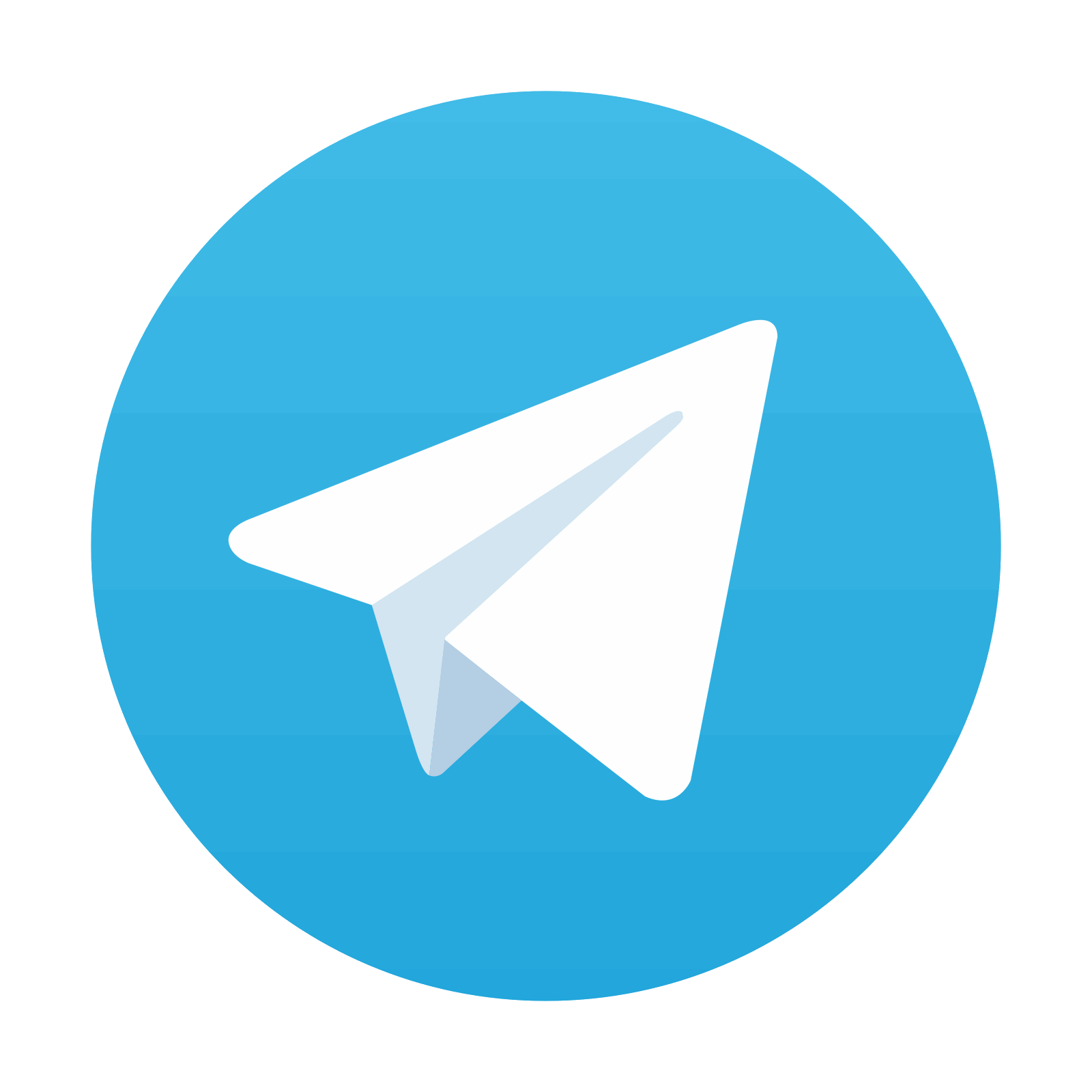
Stay updated, free dental videos. Join our Telegram channel

VIDEdental - Online dental courses
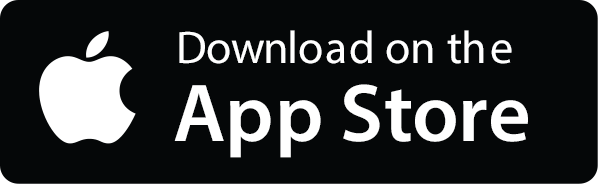
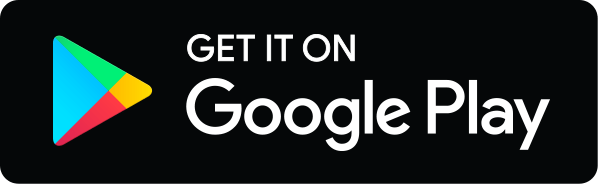