Graphical abstract
Highlights
- •
Leakage is quantified using micro-CT, AgNO 3 infiltration and image segmentation.
- •
Substantial leakage occurs in restorations with interfacial imperfection/debonding.
- •
Leakage occurs mostly at the adhesive-tooth interface of the systems investigated.
Abstract
Objective
To develop a method for quantifying leakage in composite resin restorations after curing, using non-destructive X-ray micro-computed tomography (micro-CT) and image segmentation.
Methods
Class-I cavity preparations were made in 20 human third molars, which were divided into 2 groups. Group I was restored with Z100 and Group II with Filtek LS. Micro-CT scans were taken for both groups before and after they were submerged in silver nitrate solution (AgNO 3 50%) to reveal any interfacial gap and leakage at the tooth restoration interface. Image segmentation was carried out by first performing image correlation to align the before- and after-treatment images and then by image subtraction to isolate the silver nitrate penetrant for precise volume calculation. Two-tailed Student’s t -test was used to analyze the results, with the level of significance set at p < 0.05.
Results
All samples from Group I showed silver nitrate penetration with a mean volume of 1.3 ± 0.7 mm 3 . In Group II, only 2 out of the 10 restorations displayed infiltration along the interface, giving a mean volume of 0.3 ± 0.3 mm 3 . The difference between the two groups was statistically significant ( p < 0.05). The infiltration showed non-uniform patterns within the interface.
Significance
We have developed a method to quantify the volume of leakage using non-destructive micro-CT, silver nitrate infiltration and image segmentation. Our results confirmed that substantial leakage could occur in composite restorations that have imperfections in the adhesive layer or interfacial debonding through polymerization shrinkage. For the restorative systems investigated in this study, this occurred mostly at the interface between the adhesive system and the tooth structure.
1
Introduction
One of the major functions of a dental restoration is to cover the exposed dental tissues following caries removal. A tight seal around the margin will provide protection against pulpal damage and recurrent tooth decay . Despite the many advances in adhesive technologies, it remains a challenge to adequately seal the restoration margins and prevent leakage for a sufficiently long period of time. Therefore, new adhesive systems and composite materials are still being developed which require assessment .
Several techniques have been used to assess interfacial gap formation and microleakage, but the results vary considerably . The majority of them involve the use of dyes as tracers: a dental restoration is immersed in a specific dye solution for a period of time, after which the specimen is sectioned for visual examination of dye penetration around the restorative material . One of the main drawbacks of this method is that it only provides a qualitative assessment, namely, confirmation of the presence or absence of the dye in the particular section studied. A variant of this approach incorporates a non-parametric scale, providing a semi-quantitative score according to the degree of dye penetration. However, the score definitions and the scale used vary among the studies, making the results from them not comparable. This qualitative or semi-quantitative evaluation makes the test itself not very reliable or discriminative. In addition, evaluation can only be carried out in the plane through which the sample is sectioned, which can be misleading because dye infiltration does not occur uniformly throughout the interface (see later). Therefore, it is necessary to develop techniques to accurately quantify interfacial leakage.
X-ray micro-computed tomography (micro-CT) has been used recently to study composite shrinkage, gap formation and microleakage . It allows 3D reconstruction of the entire dental restoration and its surrounding tissues. The different components are identified based on the differences in their ability to attenuate the X-ray, with the differences being converted into a range of greyscale values. Micro-CT has the advantage of being non-destructive, i.e., the specimens do not need to be destroyed, allowing them to be reused for temporal assessment, for example.
In order to use micro-CT to study interfacial integrity in composite restorations, a radiopaque dye is often necessary . This is because the low density of most dental adhesives makes it difficult to distinguish them from any gaps that may form at the tooth-restoration interface. Previous studies exploring marginal infiltration of glass-ionomer sealant and Class-II composite restorations showed that marginal leakage along the interface could be traced when micro-CT is used in combination with a radiopaque dye. However, in both of these studies only a cross-sectional analysis was performed, with a non-parametric scale being applied to assess infiltration. The assessments were therefore only semi-quantitative, as explained above.
3D analysis of polymerization shrinkage of a dental composite and the resulting gap formation has been performed using micro-CT to predict microleakage . In , composites placed in plastic holders with different volumes were studied. To evaluate shrinkage, the volume of the composite before and after curing was calculated after 3D reconstruction. Also, direct measurement of the gap between the composite and the holder was performed to predict leakage. Gap formation and, hence, the predicted microleakage was shown to be non-uniform around the interface, being highly dependent on the C-factor, i.e. the ratio between the bonded and unbonded surfaces, and the composite volume . A later study reported similar results when composite restorations were placed in human molars . These studies showed that 3D quantitative assessment of microleakage could be obtained with micro-CT, which overcame one of the main disadvantages of conventional techniques for microleakage analysis. However, in both of these studies an adhesive was not used in order to simplify the system, possibly because the radiolucency of the adhesive would make it difficult to differentiate it from a gap in the micro-CT images. Needless to say, clinically, the adhesive plays an important role in the formation of interfacial gaps and must therefore be included when microleakage and gap formation are being studied. Additionally, in the latter study the dental restoration was placed only in the dentin portion of the cavity, leaving bonding to the enamel surface out of the investigation. The reason for this omission was the difficulty to separate the gray values of enamel and those of the restoration in the CT images.
In order to properly assess leakage, it is important to replicate the clinical situation as closely as possible in any in vitro study by involving all the components in a restored tooth. To overcome the limitations associated with the techniques mentioned above, the aim of this study is to propose an improved and more comprehensive method using micro-CT to quantify interfacial leakage in dental restorations.
Recently, interfacial debonding of composites restorations that occurred during polymerization was monitored in situ using the Acoustic Emission (AE) technique . AE events were recorded in real time from human molars with Class-I cavities restored with either Z100™ (Z100) or Filtek™ LS (LS) (both from 3 M ESPE, St. Paul, MN, USA) during curing. The number of AE events recorded from the specimens restored using Z100 was much higher than that found in the specimens restored with LS. The results were confirmed by micro-CT imaging which showed clear interfacial debonding in the former but not the latter. These AE results will be compared directly with the volumetric calculation of the dye penetrant obtained in this study.
2
Materials and methods
2.1
Sample preparation
Twenty human third molars were selected for this study. The use of human molars was approved by the University of Minnesota Institutional Review Board. The teeth were cleaned by removing the soft tissues and hard deposits attached to the surface and then kept in 0.1% thymol solution. Class-I cavity preparations, approximately 2-mm high, 2-mm wide and 4-mm long, were made on the occlusal surface of each tooth. The teeth were divided randomly into two groups of 10. Group I was restored with Z100 ( Table 1 ), which was considered for the purpose of this paper as a high-shrinkage composite. Group II was filled with LS ( Table 1 ), a low-shrinkage composite by comparison. For Group I, total etching of the tooth cavity was performed with 37% Scotchbond™ Etchant for 20 s, followed by rinsing with the triple syringe for 40 s. Adper™ Single Bond II (3 M ESPE, St. Paul, MN, USA) was then used as the bonding system. For Group II, Filtek™ LS System Adhesive (3 M ESPE, St. Paul, MN, USA), a self-etch primer and bonding system, was used. The manufacturer’s guidelines were followed closely when applying the bonding systems. After placing the bonding system, the corresponding composite, either Z100 or LS (both A2 shade), was placed incrementally into the cavity. Each layer of composite, which was no more than 2-mm thick, was cured for 40 s, utilizing an Elipar™ S10 LED curing light (3 M ESPE, St. Paul, MN, USA) with an irradiance of 1200 mW/cm 2 . Thereafter, each restoration was polished by using a polishing cup.
Product | Composition | Batch number |
---|---|---|
Z100™ restorative | Silane treated ceramic, triethylene glycol dimethacrylate (TEGDMA), bisphenol a diglycidyl ether dimethacrylate (BISGMA), 2-benzotriazolyl-4-methylphenol | N362970 |
Filtek™ LS Low shrink posterior restorative | Silane treated quartz, 3,4 epoxycyclohexylcyclopolymethylsiloxane, bis-3,4 epoxycyclohexylethyl-phenyl-methylsilane, yttrium trifluoride, mixture of other by-products mixture of epoxy-mono-silanole by-products, mixture of epoxyfunctional di- and oligo-siloxane by-products, mixture of alpha-substituted by-products borate(1-), tetrakis(pentafluorophenyl)-[4-(methylethyl)phenyl](4-methylphenyl)iodonium | N296926 |
Adper single bond plus | Ethyl alcohol, silane treated silica (nanofiller), bisphenol a diglycidyl ether dimethacrylate (BISGMA), 2-hydroxyethyl methacrylate(HEMA), glycerol 1,3-dimethacrylate, copolymer of acrylic and itaconic acids, water, diurethane dimethacrylate (UDMA) diphenyliodonium hexafluorophosphate, ethyl 4-dimethyl aminobenzoate (EDMAB) | N509492 |
Filtek™ LS system adhesive self-etch primer and bond | Primer: bisphenol a diglycidyl ether dimethacrylate (BISGMA), 2-hydroxyethyl methacrylate (HEMA), Phosphoric acid-methacryloxy-hexylesters mixture, ethanol, water, silane treated silica, 1,6-hexanediol dimethacrylate, (dimethylamino)ethyl methacrylate, copolymer of acrylic and itaconic acid, Phosphine oxide, Dl-camphorquinone, ethyl 4-dimethyl aminobenzoate, methyl alcohol Bond: substituted dimethacrylate, silane treated silica, triethylene glycol dimethacrylate (TEGDMA), phosphoric acid methacryloxy-hexylesters mixture, Dl-camphorquinone, 1,6-hexanediol dimethacrylate |
N348567 N391675 |
Each sample was then positioned in a plastic holder and fixed with acrylic resin with the purpose of avoiding sample movement during the experiment ( Fig. 1 ). The holder was specially machined to generate an interlock with the supporting base attached to the stage inside the micro-CT chamber so that the sample could be placed in the same position and orientation for scanning before and after treatment with silver nitrate. The crown was covered with nail polish, leaving a gap of ∼1 mm around the tooth-restoration interface. This allowed the silver nitrate to penetrate the interfacial gaps that might form and prevented it from entering the interface through other defects on the crown surfaces.
2.2
Scanning and AgNO 3 treatment
Scanning of the specimens was performed using a Micro-CT machine (XT H 225, Nikon Metrology Inc., Brighton, MI, USA); see Fig. 1 . The scanning parameters used were 90 kV, 90 μA, 708 ms of exposure, 720 projections and 4 frames per projection. The position of each specimen within the micro-CT machine for the baseline scan was stored in a file that could be loaded in a subsequent scan to return it back to the same position ( Fig. 1 ). The total scanning time was approximately 30 min for each specimen. A small piece of wet cotton was used to cover the specimen to prevent it from drying and cracking during scanning.
To reveal any interfacial defects or debonding between the restoration and the tooth structure after curing, the specimens were submerged in a radiopaque silver nitrate solution (AgNO 3 , 50% w/w) overnight ( Fig. 1 ). The pH of the AgNO 3 solution was measured as ∼3.3.
Both groups were subjected to a second scan after submersion in the AgNO 3 solution ( Fig. 1 ). The same scanning parameters as those for the baseline scans were used to ensure consistency in the greyscale values. The baseline sample position was then loaded to ensure that each specimen was rescanned in the same position within the micro-CT machine to minimize misalignment of the two image sets.
2.3
Image processing
3D reconstructions were done using the software CT Pro 3D (Nikon metrology, Inc., Brighton, MI, USA). The same volume of interest (VOI) was used for both the baseline and post-treatment scans to give the same spatial resolution. Initial visualization and 3D rendering was performed using VGStudio MAX 2.1 (Volume Graphics GmbH, Heidelberg, Germany). All the reconstructed images were scrutinized, slice-by-slice, for silver nitrate penetration, as indicated by a bright line formed by the radiopaque dye along the interface. For comparison, a series of bucco-lingual cross-sectional images were retrieved from both the baseline and the post-treatment scans for each of the samples.
It was not possible to completely isolate the voxels corresponding to the penetrant in the post-treatment images ( Fig. 2 a ) by simply selecting the narrow range of gray values for this material because some of the components of the restoration also had similar gray values. This can be seen in Fig. 2 b in which the silver nitrate and the dense components in the restoration are highlighted by the same false color. To overcome this issue, an image correlation function was used to align the images taken before and after dye penetration as- shifting of images in space was likely the result of play in the fixture and/or the moving table of the micro-CT system . The image alignment was followed by image subtraction to remove the composite restoration and dental tissues from the images, leaving behind the silver nitrate only ( Fig. 2 c). Thus, the gray values of the baseline images were subtracted from those of the post-treatment images. The image processing was carried out using MATLAB and the Image Processing Toolbox (Mathwork ® ) . The image subtraction procedure resulted in new image stacks, which could then be rendered to reveal the thin layer of silver nitrate in 3D space ( Fig. 2 d). The final subtracted images were exported as bmp extension files for further analysis.
In theory, only the voxels corresponding to the silver nitrate penetrant would have non-zero gray values after image subtraction. However, the presence of noise resulted in regions with non-zero gray values that were not occupied by silver nitrate. To better quantify the silver nitrate penetration, the resulting subtracted images were further processed using SkyScan CT-analyzer Version 1.1 (Bruker microCT, Kontich, Belgium). First, a region of interest was defined to remove most of the background information. A threshold gray value was then selected to remove the remaining background and to reduce the noise for the subsequent image binarization step. The threshold value was chosen such that the amount of silver nitrate identified at the tooth-restoration interface was not altered significantly while removing the remaining background and noise elsewhere, as shown in Fig. 3 a and b . The resulting binary images were visually compared with the post-treatment images to further confirm that the threshold value selected was appropriate. 3D rendering of the silver nitrate penetration was then performed, followed by calculation of its volume and the mean value for each group of specimens.
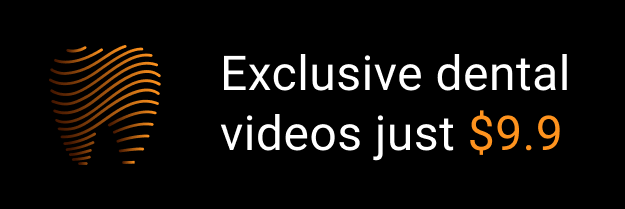