Highlights
- •
Acidogenicity of S. mutans biofilms was reduced by glass ionomer cements (GICs).
- •
Composition of S. mutans biofilms was affected by GICs.
- •
Anti-cariogenic biofilm activity of GICs was correlated with fluoride release rate.
Abstract
Objectives
The aim of this study was to evaluate acidogenicity and composition of Streptococcus mutans biofilms on glass ionomer cements (GICs) and then to determine the relationship between the anti- S. mutans biofilm activity and fluoride release rate of the GICs.
Methods
S. mutans biofilms were formed on discs prepared using five commercial GICs. Acid production and fluoride release rates of the biofilms on the GIC discs during biofilm formation (0–94 h) were determined. Next, 94-h-old S. mutans biofilms on GIC discs were analyzed to evaluate the biofilm composition (dry weight, bacterial cell number, and extra-cellular polysaccharide (EPS) amount) using microbiological, biochemical, and confocal laser scanning microscopy (CLSM) methods. Lastly, relationships between the fluoride release rate and changes in acidogenicity and composition of the biofilms were determined using a linear-fitting procedure.
Results
All of the tested GICs released fluoride ions. Of the GICs, the two that showed the highest fluoride release rates strongly affected acidogenicity, dry weight, and EPS formation of the biofilms. Furthermore, they reduced the bacterial and EPS bio-volumes and EPS thickness. However, the number of colony forming units (CFUs) of the biofilms was higher than that of the control. Generally, changes in the acidogenicity and composition (except for CFU count) of the biofilms on the GICs followed a negative linear-pattern of fluoride release rate-dependence ( R = −0.850 to −0.995, R 2 = 0.723–0.990).
Significance
These results suggest that the anti-cariogenic biofilm activity of GICs is closely correlated with their fluoride release rate during biofilm formation.
1
Introduction
It has been well documented that biofilm formation occurs on the surfaces of different restorative materials within a short time after placement in the oral cavity . The biofilms on the microgaps between restorative materials and tooth tissues can lead to secondary caries, which is responsible for half of all restorations fail within 10 years . Similar to the dental caries process, the biofilms on these microgaps cause dissolution of the adjacent tooth surfaces and reduce the longevity of the restorative materials .
Although several studies have shown that the level of mutans streptococci is not necessarily related to the development of dental caries , many other studies have revealed Streptococcus mutans as one of the main causative pathogens for dental caries . S. mutans can adhere to and accumulate on the tooth surface using extra-cellular polysaccharides (EPSs) produced by glucosyltransferases (GTFs) from sucrose . Furthermore, this bacterium can metabolize dietary sugars to organic acids and withstand rapid and substantial fluctuations in the environmental pH . The combination of these virulence properties allows S. mutans to effectively colonize the tooth surface and be sustained in the oral cavity.
To prevent secondary caries, fluoride-releasing restorative materials such as glass ionomer cements (GICs) and resin composites have been developed. Recently, several researchers have shown that nanocomposites containing calcium phosphate nanoparticles may reduce secondary caries . Of the fluoride-releasing restorative materials, GICs have been used in various areas of restorative dentistry for several decades because of their biocompatibility and cariostatic properties . Furthermore, GICs can reduce demineralization in adjacent hard tooth tissues . Several studies have shown that the effectiveness of GICs may be related to their ability to release fluoride .
Biofilms on GICs can degrade material properties and roughen their surfaces, which in turn promote further biofilm formation, as well as material surface deterioration . Recently, several studies have investigated bacterial adherence to and biofilm formation on GICs and have shown that these materials can affect bacterial adherence, acidigenicity, and biofilm formation . However, although it is important to obtain information about whether GICs can affect composition of biofilms on GICs, little has been reported. Furthermore, there has been no study on the precise relationship between fluoride release level from GICs and changes in virulence and composition of biofilms on GICs.
Considering the advantages and widespread use of GICs in restorative dentistry, it would be worthwhile to test the hypotheses that fluoride level released from GICs can change virulence and composition of cariogenic biofilms. Therefore, the aim of this study was to investigate the changes in virulence, especially acidogenicity, and composition of cariogenic biofilms formed on GICs and then to determine the relationships between fluoride release level from GICs and changes in acidogenicity and composition of cariogenic biofilms using an S. mutans biofilm model.
2
Materials and methods
2.1
GIC disk preparation
Table 1 lists the materials used in this study. Five GICs were selected: Glaslonomer FX-II (Gla), Ketac Fil Plus Aplicap (Keta), Riva self-cure HV (Riva), GC Fuji Filling LC (GC), and GC Fuji II LC (GC2). The GICs used in this study were all commercially available, and the shade of all materials was A2 or A3. These GICs were used to prepare disk-shaped specimens according to the manufacturer’s recommendations.
Product name | Curing | Composition (wt.%) | Manufacturer |
---|---|---|---|
Glaslonomer FX-II | Self-cured | – Glass polyalkenoate. | Shofu, Inc., Japan |
– Powder (15 g), liquid (8 ml, 10 g). | |||
Ketac™ Fil Plus Aplicap™ | Self-cured | – Glass powder (>99%). | 3 M ESPE, Dental Products, Germany |
– Liquid: copolymer of acrylic acid-maleic acid (35–55%), water (40–55%), tartaric acid (5–10%). | |||
Riva self-cure HV | Self-cured | – Polyacrylic acid (20–30%). | SDI Limited, Australia |
– Tartaric acid (10–15%). | |||
– Fluoroaluminosilicate glass (90–95%). | |||
– Polyacrylic acid (5–10%). | |||
GC Fuji Filling LC | Light-cured | – Paste A: Alumino-fluoro-silicate glass (amorphous) (75–85%); 2-hydroxyethyl methacrylate (10–12%); Urethanedimethacrylate (2–5%). | GC corporation, Japan |
– Paste B: Distilled water (20–30%); polyacrylic acid (20–30%); urethanedimethacrylate (12–15%); silicone dioxide (fumed/amorphous) (10–15%). | |||
GC Fuji II LC (improved) | Light-cured | – Powder: Alumino-fluoro-silicate glass (95–100%). | GC corporation, Japan |
– Liquid: Polyacrylic acid (20–25%); 2-hydroxyethyl methacrylate (30–35%); proprietary ingredient (5–15%); 2, 2, 4, trimethylhexamethylenedicarbonate (1–5%). |
Disk-shaped specimens (12 mm in diameter and 1.2 mm in thickness) were prepared using polytetrafluoroethylene (Teflon) molds with a metal holder and glass slides to cover each face. Gla, Keta, and Riva specimens were self-cured. GC and GC2 specimens were light-cured for 20 s on each face using a light curing unit (G-Light, GC Corp., Japan). After curing, all specimens were polished sequentially with # 800 to # 1200 sand papers. Then the specimens were placed in a desiccator at room temperature. Hydroxyapatite discs (12 mm in diameter and 1.2 mm in thickness; Clarkson Chromatography Products, Inc., South Williamsport, PA, USA) were included as a control in this study.
2.2
Biofilm formation on GIC discs
The microorganism used for this study was S. mutans UA159 (serotype c). S. mutans biofilms were formed on saliva-coated GIC or hydroxyapatite (HA) discs placed in a vertical position in 24-well plates, as detailed elsewhere . Briefly, the saliva-coated discs were generated by incubation with filter-sterilized (0.22 μm low protein-binding filter) human whole saliva for 1 h at 37 °C. For biofilm formation, the saliva-coated discs were transferred to a 24-well plate containing 1% sucrose (v/v) ultrafiltered (10 kDa molecular-weight cut-off) tryptone yeast-extract (UTE) broth with S. mutans UA159 (2–5 × 10 6 colony forming units (CFUs)/ml). The biofilms were grown undisturbed for 22 h to allow initial biofilm growth. From this time point (22 h), the culture medium was changed twice daily (9 AM, 6 PM) until it was 94 h old. The culture medium was changed a total of six times. The pH value and fluoride concentration in the old culture medium were determined during the experimental period (until biofilms reached 94 h of age). The 94-h-old biofilms were used to analyze the biofilm composition. Each assay was performed in duplicate in at least six different experiments ( n = 12).
2.3
Determination of fluoride concentration and pH value during biofilm formation
To evaluate fluoride release from GICs, the concentration of fluoride in the old culture medium was determined during the experimental period (22, 31, 46, 55, 70, 79, and 94 h). For the determination of fluoride concentration, a total of 2.8 ml of each medium was mixed with 280 μl of total ionic strength adjustment buffer (TISAB III). The fluorometer (Thermo Fisher Scientific, Orion, MA, USA) was calibrated using four standard solutions (0.1, 1, 10, and 100 ppm F − ). To evaluate the acidogenicity of S. mutans biofilms on GICs, the pH values of the old culture medium were also determined during the experimental period (22, 31, 46, 55, 70, 79, and 94 h) using a pH electrode. To calculate acid production or fluoride release rates at 0–22, 22–31, 31–46, 46–55, 55–70, 70–79, and 79–94 h, the concentrations of H + or fluoride in the old culture medium was divided by the respective incubation time. In addition, the mean rates of acid production and fluoride release during biofilm formation (0–94 h) were also calculated using the respective acid production or fluoride release rates (0–22, 22–31, 31–46, 46–55, 55–70, 70–79, and 79–94 h).
2.4
Analysis of 94-h-old S. mutans biofilm composition
2.4.1
Microbiological and biochemical analyses
The 94-h-old biofilms on the test materials were transferred into 2 ml of 0.89% NaCl and sonicated in an ultrasonic bath for 10 min to disperse the biofilms. The dispersed solution was re-sonicated at 7 W for 30 s after adding 3 ml of 0.89% NaCl (VCX 130PB; Sonics and Materials, Inc., Newtown, CT, USA). An aliquot (0.1 ml) of the dispersed solution was serially diluted and plated onto brain heart infusion (BHI) agar plates to count the CFUs. For the determination of the dry weight and amount of water-insoluble extracellular polysaccharides (water-insoluble EPSs), the remaining solution (4.9 ml) was centrifuged (3000 × g ) for 20 min at 4 °C. The biofilm pellet was resuspended and washed twice in the same volume of water. The washed pellet was lyophilized and weighed to determine the dry weight. After weighing, the water-insoluble EPSs were extracted from the dry pellet using 1 N sodium hydroxide before determination of polysaccharide amount, as detailed elsewhere .
2.4.2
Confocal laser scanning microscopy study
In addition to the microbiological and biochemical analyses, a confocal laser scanning microscopy (CLSM) study was performed to evaluate bacterial cells and EPSs of the 94-h-old biofilms on GICs, as described by Chau et al. . Briefly, Alexa Fluor ® 647-labeled dextran conjugate (10,000 MW; absorbance/fluorescence emission maxima 647/668 nm; Molecular Probes, Inc., Eugene, OR, USA) was added to the culture medium during biofilm formation at a concentration of 1 μM. After 94 h, the bacterial cells in the biofilms were labeled by means of 25 μM SYTO ® 9 green-fluorescent nucleic acid stain (480/500 nm; Molecular Probes, Inc., Eugene, OR, USA) for 30 min . CLSM imaging of the biofilms was performed using the LSM 510 META microscope (Carl Zeiss, Jena, Germany) equipped with argon-ion and helium–neon lasers. Two independent experiments were performed, and seven image stacks (512 × 512 pixel tagged image file format) per experiment were collected. The biofilms were quantified from the confocal stacks using the image-processing software COMSTAT . This software was written as a script in Matlab 5.1 (The MathWorks, Natick, MA, USA) equipped with the Image Processing Toolbox. In this study, the bio-volume and thickness of bacteria and polysaccharides were analyzed. Bio-volume is defined as the volume of the biomass (μm 3 ) divided by the surface area of the substratum (GIC or hydroxyapatite discs) (μm 2 ).
2.5
Statistical analysis
The data are presented as mean ± standard deviation. The intergroup differences were estimated using one-way analysis of variance (ANOVA). Values were considered statistically significant when the p value was <0.05.
To determine the relationships between the mean rate of fluoride release and the mean rate of acid production, dry weight, CFUs, amount of water-insoluble EPSs, bacterial bio-volume, bacterial thickness, EPS thickness, or EPS bio-volume, a linear-fitting procedure was performed using a linear regression analysis program (Origin 7.0; Microcal, Inc., Northampton, MA, USA). The correlation coefficient ( R ) and determination coefficient ( R 2 ) of each fit line were also calculated.
2
Materials and methods
2.1
GIC disk preparation
Table 1 lists the materials used in this study. Five GICs were selected: Glaslonomer FX-II (Gla), Ketac Fil Plus Aplicap (Keta), Riva self-cure HV (Riva), GC Fuji Filling LC (GC), and GC Fuji II LC (GC2). The GICs used in this study were all commercially available, and the shade of all materials was A2 or A3. These GICs were used to prepare disk-shaped specimens according to the manufacturer’s recommendations.
Product name | Curing | Composition (wt.%) | Manufacturer |
---|---|---|---|
Glaslonomer FX-II | Self-cured | – Glass polyalkenoate. | Shofu, Inc., Japan |
– Powder (15 g), liquid (8 ml, 10 g). | |||
Ketac™ Fil Plus Aplicap™ | Self-cured | – Glass powder (>99%). | 3 M ESPE, Dental Products, Germany |
– Liquid: copolymer of acrylic acid-maleic acid (35–55%), water (40–55%), tartaric acid (5–10%). | |||
Riva self-cure HV | Self-cured | – Polyacrylic acid (20–30%). | SDI Limited, Australia |
– Tartaric acid (10–15%). | |||
– Fluoroaluminosilicate glass (90–95%). | |||
– Polyacrylic acid (5–10%). | |||
GC Fuji Filling LC | Light-cured | – Paste A: Alumino-fluoro-silicate glass (amorphous) (75–85%); 2-hydroxyethyl methacrylate (10–12%); Urethanedimethacrylate (2–5%). | GC corporation, Japan |
– Paste B: Distilled water (20–30%); polyacrylic acid (20–30%); urethanedimethacrylate (12–15%); silicone dioxide (fumed/amorphous) (10–15%). | |||
GC Fuji II LC (improved) | Light-cured | – Powder: Alumino-fluoro-silicate glass (95–100%). | GC corporation, Japan |
– Liquid: Polyacrylic acid (20–25%); 2-hydroxyethyl methacrylate (30–35%); proprietary ingredient (5–15%); 2, 2, 4, trimethylhexamethylenedicarbonate (1–5%). |
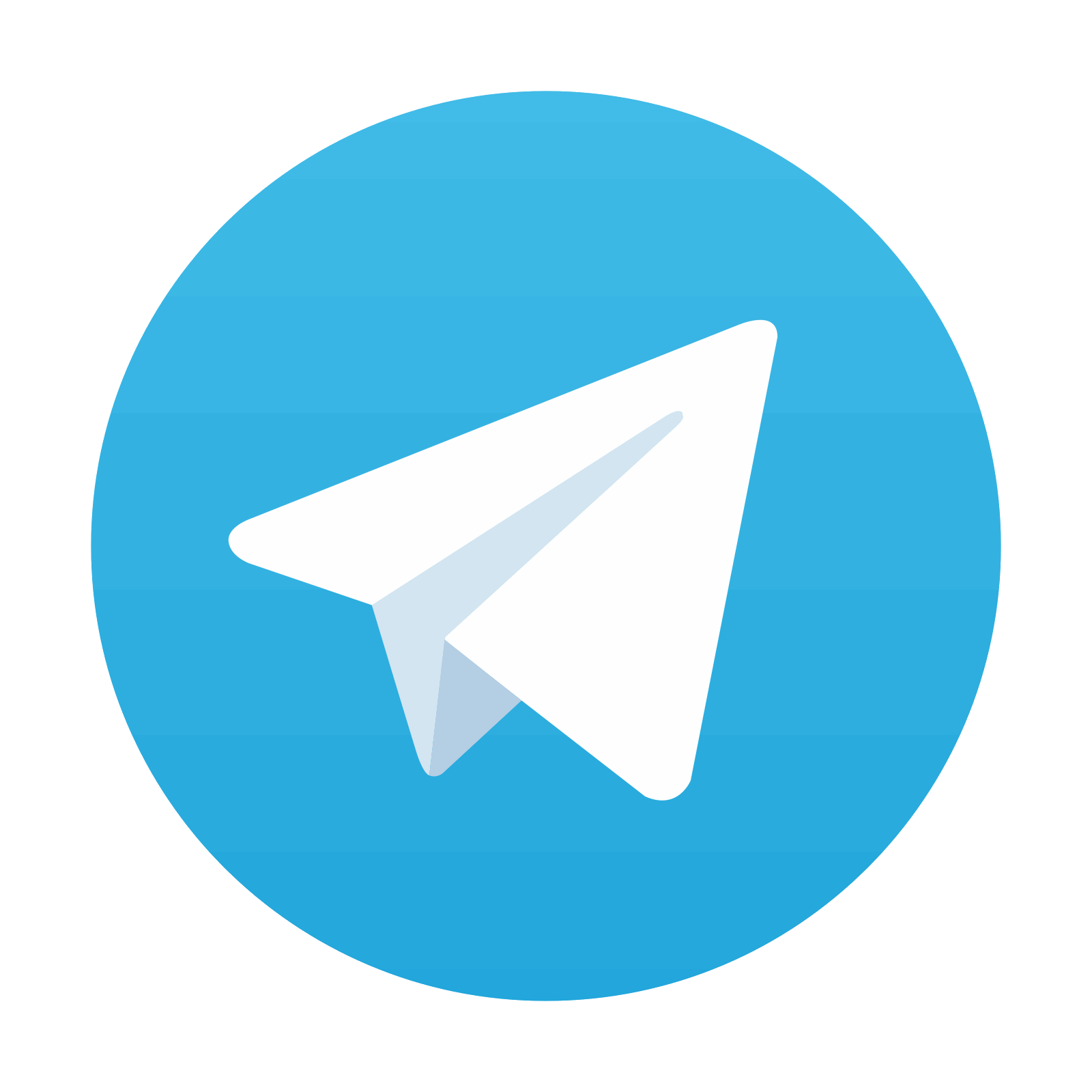
Stay updated, free dental videos. Join our Telegram channel

VIDEdental - Online dental courses
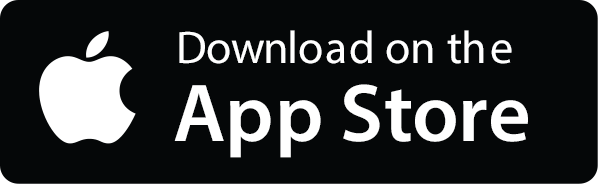
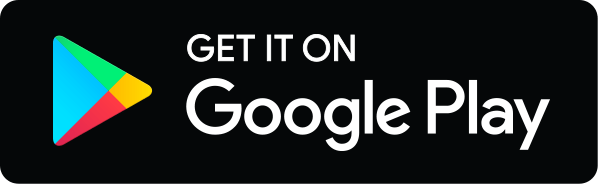
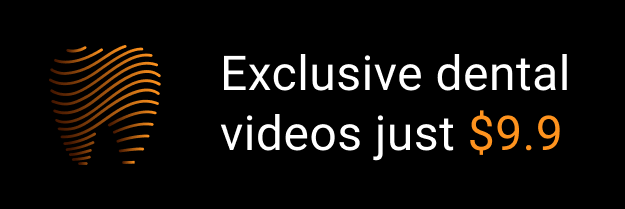