© The Author(s) 2015
Keiichi Sasaki, Osamu Suzuki and Nobuhiro Takahashi (eds.)Interface Oral Health Science 201410.1007/978-4-431-55192-8_26
26. Prospects for Liposome-Encapsulated Nisin in the Prevention of Dental Caries
(1)
Department of Chemistry, National Defense Medical College, Tokorozawa 359-8513, Japan
(2)
Research and Development Division, BioMedCore Inc., Sapporo 001-0021, Japan
(3)
Eco Friendly Institute Ltd., Fukuoka 818-0013, Japan
(4)
Department of Preventive Medicine and Public Health, National Defense Medical College, 3-2 Namiki, Tokorozawa Saitama, 359-8513, Japan
Abstract
Dental caries is a common oral bacterial infectious disease. Its prevention requires the control of the causative pathogens, such as Streptococcus mutans, that exist within dental plaque. Nisin is a proteinaceous bacteriocin produced by Lactococcus lactis that is used to suppress bacterial infections. It has an inhibitory mode of action on a wide range of gram-positive bacteria. Improvements in the medical benefits of antibacterial agents can be achieved if they can be retained in liposomes for a long period after administration. Liposome systems can increase the ability of the encapsulated compounds, which have been widely used to encapsulate many kinds of compounds in various scientific settings. Liposomes can release labile molecules at a moderate rate. Liposome technologies that effectively protect the encapsulated molecules from decomposition have the potential to improve their preventive and therapeutic effects. Therefore, the use of liposomes to administer antimicrobial agents has spurred research into their utility in preventive medicine. The encapsulation of nisin in liposomes can provide means of improving the stability of nisin and its antibacterial effect against S. mutans. The present chapter will review the prospects for liposome-encapsulated nisin for the prevention of oral infectious diseases.
Keywords
Dental cariesLiposomeNisinPreventive medicine Streptococcus mutans
26.1 Introduction
Oral diseases related to cariogenic microorganisms, such as dental caries, affect majority of the world’s populations [1]. Dental caries results from interaction of specific bacteria with dietary carbohydrates in the oral cavity. Streptococcus mutans is a key contributor to the formation of cariogenic glucan biofilms. Nisin is an effective bactericidal agent against gram-positive bacteria [2]. It is a bacteriocin with a molecular mass of 3,354 and is produced by certain strains of Lactococcus lactis subsp. lactis [3]. The bactericidal activity of nisin against cariogenic streptococci was investigated in vitro and the findings indicated that nisin acts as an inhibitor of typical cariogenic streptococci [4, 5]. It may be possible to use liposomes as a carrier for the effective administration of nisin. Liposomes are artificial spherical vesicles that can be created from lipids. They have been developed and evaluated as carriers to deliver encapsulated molecules to target organs and specific cells in vitro and in vivo [6–9]. Investigations on liposomes have led to their use with many substances, and the encapsulated agents are pharmacodynamically and pharmacokinetically much more efficient than the naked compounds. Liposome-encapsulated substances have been used in various applications such as to retain and control the release of antibacterial compounds [10, 11]. They have been used to deliver pharmaceuticals, and their recent application in the preservation of foods by the encapsulation of nisin has spurred further research into their use with food materials [12, 13]. With regard to liposome formulations, a variety of factors, such as lipid composition, cholesterol content, the presence of a charge on the lipid bilayer, and the size of the vesicle, affect the effectiveness of liposomes as preventive and therapeutic agents [6]. Oral delivery of liposomal bacteriocins is a useful for the treatment to prevent dental caries [5]. Encapsulation technologies, which may shield substances such as nisin from degradation by digestive enzymes, and effectively deliver the encapsulated contents at the same time, could be an advancement in the field of preventive medicine [10].
This review article provides brief background information on liposomal nisin and explores its possible applications with a focus on factors affecting its effectiveness and the advantages it offers for human health by preventing dental caries.
26.2 Cariogenic Microorganisms and Dental Caries
The oral cavity harbors many microorganisms that together constitute a complex micro-ecological environment [14]. Dental caries, a chronic infectious disease, is induced by cariogenic microorganisms such as Streptococcus spp. The key virulence factors of the microorganisms, insoluble glucans, can be identified when they colonize dental plaque on the surface of the teeth [4, 15]. S. mutans in particular has been recognized as a decisive factor in dental caries. Insoluble glucans provide specific binding sites for oral bacterial colonization of the tooth surface and confer structural integrity on the extracellular matrix, and therefore they are essential for the formation and accumulation of dental glucan biofilms [16]. Because insoluble glucans are synthesized by glucosyltransferases of S. mutans and these glucans provide a matrix for dental plaque biofilm, the streptococcus is considered to contribute to the formation of cariogenic biofilms [16, 17]. The streptococcus tenaciously adheres to the glucan and is highly acidgenic and acid-tolerant; these are critical virulence properties in the pathogenesis of dental caries [18]. Therefore, dental caries results from the interaction of S. mutans with dietary carbohydrates in the oral cavity; the formation of insoluble glucan biofilms on the tooth surface is a key result of the diet-bacterium interaction [19].
26.3 Nisin as a Tool for Preventive Medicine
Nisin is approved for use in foods and is employed as a food preservative in more than 50 countries [20]. It belongs to a group of bactericidal peptides called type A lantibiotics [21]. Two main natural variants of nisin, nisin A and nisin Z, have been discovered [3]. Nisin Z is widely distributed and contains asparagine instead of histidine at position 27 of the amino acid sequence of nisin A. The two variants have nearly equal bactericidal activity, membrane insertion, and pore-forming ability. Nisin is bactericidal against a broad range of gram-positive microorganisms, such as Staphylococcus aureus and Listeria monocytogenes, and prevents the outgrowth of many Clostridium spp. and Bacillus spp. [11, 13]. However, stability issues such as proteolytic degradation and oxidation result in reduced bioactivity. It is soluble and stable at acidic pH, but at alkaline pH values its solubility decreases and it becomes biologically inactive [22]. Nisin is a peptide composed of 34 amino acids, including one lanthionine, four β-methyl-lanthionine, one dehydrobutyrine, and two dehydroalanine residues. The internal thioester rings formed by lanthionine are responsible for the conformation of nisin [23]. The lanthionine rings act as conserved binding motifs for the recognition of specific targets and create segments of defined spatial structures in the nisin molecule [24]. The bactericidal activity of nisin has been suggested to be a result of electrostatic interaction of the positively charged carboxylterminal end of the molecule with negatively charged bacterial membrane lipids [25].
Nisin antagonizes a broad spectrum of gram-positive bacteria [26, 27]. It has dual activity against spore-forming bacteria, it inhibiting the outgrowth of spores and killing cells in the vegetative state. The didehydro amino acid residues in nisin interact with the membrane sulfhydryl groups of germinating spores [26]. Membrane disruption is considered to be the result of incorporation of nisin into the membrane and subsequent ion channel or pore formation [25]. Membrane potential is abolished in sensitive gram-positive cells as a result of the efflux of K+, amino acids and ATP through the membrane pores [28]. Subsequent leakage of ions causes catastrophic changes in transmembrane potential and internal pH. Nisin interacts with high-affinity pyrophosphate binding sites on the membrane-bound cell wall precursor Lipid II, leading to more effective formation of pores and inhibition of cell wall peptidoglycan biosynthesis [25, 29]. From these investigations, it is evident that nisin should be an effective antibacterial agent [30, 31].
26.4 Nisin Against Cariogenic Streptococci
Some antimicrobial agents, such as chlorohexidine, triclosan, xylitol, and cetylpyridinium chloride, have attracted interest as agents for prophylaxis against dental caries by inhibiting the multiplication of cariogenic microorganisms in the oral cavity [4]. However, these agents may cause some side effects such as discoloration of the teeth and tongue, drug resistance, and low solubility [4]. Because nisin is a lanthibiotic, unlike antibiotics, it does not produce drug resistance or chiasmatic resistance [21]. The bactericidal ability of nisin against cariogenic streptococci, such as S. mutans, has been investigated as a means of preventing dental caries [4]. Findings suggest that nisin has a potential to be used as a bactericidal agent to prevent dental caries. Liposomal nisin was shown to inhibit S. mutans [5]. In addition, nisin did not appear to be toxic to normal human gingival fibroblast and epithelial cells [32]. Therefore, nisin plays a role in inhibiting the viability of cariogenic streptococci.
26.5 Liposomes as a Tool for Preventive Medicine
Liposomes are formulated from phospholipid bilayers and consist of colloidal dispersions of lipids in aqueous buffers [33, 34]. Their formation is based on the interactions between phospholipids and water molecules in which the polar headgroups of phospholipids are exposed to the inner and outer aqueous phases and the hydrophobic carbohydrate tails are forced to face each other in a bilayer [12, 35]. Liposomal encapsulation has been shown to stabilize the encapsulated compound against enzymatic degradation and chemical modification [36]. Liposomes can encapsulate hydrophobic and hydrophilic molecules, prevent the decomposition of the encapsulated molecules, and release the compound at designated target organs [37, 38]. The bioactivity of encapsulated molecules can be maintained by the phospholipid vesicle until it is delivered to the target organ or cells, where the contents will be released [9, 39]. Liposomes can range in size from 40–50 nm to 1–2 μm, depending on the method of formulation, lipid components, and intended use [40]. One of the aims of liposome encapsulation in medicine is to increase the therapeutic index of the bioactive material [36, 41]. Encapsulation techniques have been developed in many fields because of the biocompatibility of liposomes, i.e., its ability to encapsulate both hydrophilic and lipophilic molecules, and its ability to deliver these molecules to target sites [42, 43]. In the food industry, liposomes have been used to deliver antibiotics, peptides, vitamins, and flavors [44, 45]. The encapsulation of bacteriocins, such as nisin in liposomes represents an alternative to overcome problems related to the exploitation of bactericidal activities [5, 40]. Liposomes have been developed and used as carriers for therapeutic agents to improve the delivery of many anticancer and antibiotic compounds [46–48].
The usefulness of conservative therapeutics is restricted by their inability to deliver compounds to target sites. Attempts have been made to overcome this difficulty by providing selective delivery and release of encapsulated compounds. Hydrophobic compounds can be directly encapsulated into liposomes during vesicle formation, and retention is governed by interactions between the compound and lipid. Passive encapsulation of soluble compounds depends on the ability of liposomes to trap aqueous buffer containing dissolved compounds during vesicle formation [49]. The applications of liposomes in medicine and pharmacology can be divided into therapeutic and preventive uses of liposomes composed of various substances [9, 50, 51]. Advances in liposome design lead to applications for the delivery of new technology products such as nucleotides involved in numerous biological functions [48, 52]. There have been many studies on the viability of a range of conservative chemicals formulated in liposomes, frequently resulting in improved biological activity compared with that of the naked form. Many antibiotics are orally available and liposome encapsulation can be developed for labile chemicals with minimal loss of utility. Therefore, liposomes are used in pharmaceutical applications and the encapsulated substances have enhanced efficacy compared with the naked substances. Liposomes are achieving medical acceptance because of the effective shelf life of labile molecules, slow and steady release of the molecules, and delivery of the molecules to required locations [46]. In pharmaceutical applications, liposomes offer drug delivery systems for therapeutic and preventive strategies [53].
26.6 Characteristics of Liposomes
The design of liposomal delivery systems accelerates the treatment on human health [6]. The strategy for liposomes is the development of carrier systems with the ability to deliver and release encapsulated compounds [54]. The characteristics of liposomes in this regard depend on their lipid composition, surface charge, and vesicular size. Because bilayer fluidity and rigidity can affect the release of liposomal compounds, studies have been carried out on the effects of phospholipids of different phase transition temperatures on fluidity and the effects of lipid composition on rigidity of liposomes [55, 56]. Liposomes with many different lipid compositions exist, and those that include phospholipids provide efficient vesicles for individual applications [57].
The aim of lipid selection for liposomes is to provide stabilizing and encapsulation abilities. Phosphatidylcholines have been selected for the preparation of liposome vesicles in many settings. The amount of encapsulated content released from vesicles is proportional to the acyl chain length of the phosphatidylcholine. Dimyristoylphosphatidylcholine (DMPC), dipalmitoylphosphatidylcholine (DPPC) and distearoylphosphatidylcholine (DSPC) have longer saturated acyl chains (14, 16, and 18 carbons, respectively) and their phase transition temperature increases with increasing chain length [58]. The trend in the release of encapsulated compounds from vesicles is in the order of DMPC > DPPC > DSPC, which reflects the fluidity of vesicles composed of DMPC, DPPC, and DSPC with a phase transition temperature of 23, 41, and 55 °C respectively [57]. For example, the configuration of DPPC is a highly ordered gel phase at room temperature; however, above 41 °C the lipid forms a liquid crystalline phase characterized by greater packing disorder because of temperature-induced changes in acyl chain composition [58]. Unsaturated phospholipid species show more permeable and fluidic bilayers, but saturated phospholipids with long acyl chains form a rigid, rather impermeable bilayer structure [33, 37].
The cholesterol in phosphatidylcholine-based liposomes can reduce liposome permeability, because membrane permeability is dependent on fluidity and rigidity [59]. Cholesterol interacts with fatty acids in liposomes by hydrogen bonding, increasing the cohesiveness and mechanical strength of the vesicular membrane [60]. For example, a comparison of nisin-containing phosphatidylcholine liposomes and phosphatidylcholine plus cholesterol showed that cholesterol reduced the release of nisin [38, 61]. The permeability of liposomes can be altered by modifying the cholesterol concentration according to the intended application of the liposomes [57, 62]. Sphingomyelin also increases the rigidity of the bilayer [6]. Therefore, the modification of liposomal composition enables the preparation of preferred kinds of liposomes for various scientific settings.
The effect of charge on lipid behavior is governed by the surface charge density of the liposomes, lipid head groups, and interactions between the encapsulated content and lipid [63, 64]. Liposomes composed of charged polar lipids with higher electrical charges are more stable than those composed of neutral polar lipids. A surface charge on liposomes increases repulsive interactions and reduces the frequency of liposome collisions [65]. With regard to control of the release of encapsulated compounds from liposomes, the charge has been observed to affect vesicle permeability [63]. The use of negatively charged liposomes containing phosphatidylglycerol led to greater effectiveness of the encapsulated molecules compared with the use of neutral or positively charged liposomes. Therefore, potency of the release of encapsulated compounds has been shown to be regulated by polymer size, lipid composition, and surface charge [65].
Liposomes can be used as carriers of many different kinds of compound, such as peptides and nucleotides, because liposomes are spherical vesicles whose membranes are composed of one or more phospholipid bilayers [66]. Phospholipid vesicles are capable of encapsulating labile molecules, and the lipids that they are composed are biodegradable and safe in vivo [67]. Liposome encapsulation of therapeutic and cosmetic agents can extend their activity by improving their stability and permeability and by providing targeted and timed release [68
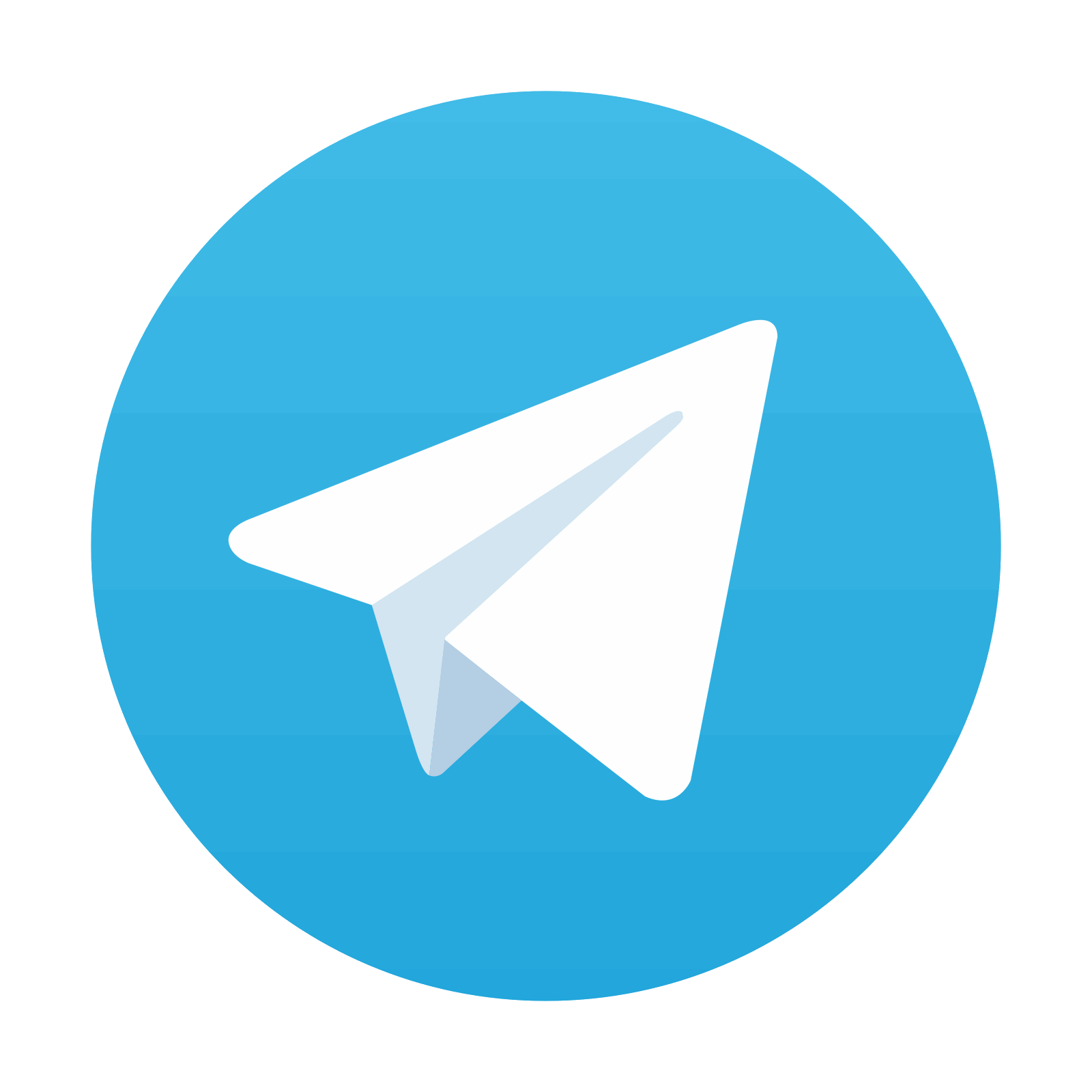
Stay updated, free dental videos. Join our Telegram channel

VIDEdental - Online dental courses
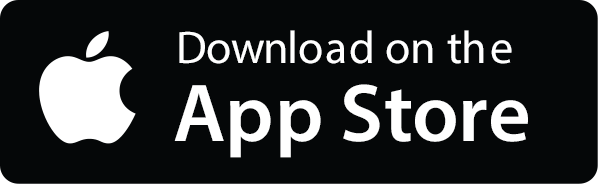
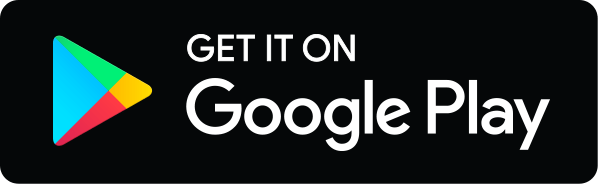