2 Prenatal development of the craniofacial region
Many of these evolutionary changes are reflected in the form and function of the craniofacial region (Fig. 2.1). The essential morphology of the head and face is fundamental to being human and the development of this region is complicated, beginning early in life and requiring the coordinated growth and interaction of all primary cell populations within the embryo.
There is also a further contribution from the neural crest (or fourth germ layer). Ectoderm and endoderm are derived from the epiblast and hypoblast, two fundamental cell populations within the bilaminar disc of the early embryo (Fig. 2.2). Mesoderm is produced during the process of gastrulation and the conversion of a bilaminar embryo into one with three primary tissue layers (Fig. 2.3).
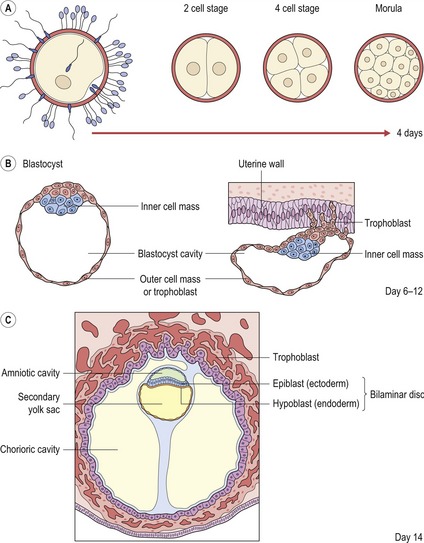
Figure 2.2 Early development of the embryo.
Redrawn from Sandler TW (ed.) (2003), Langman’s Medical Embryology, 9th edn (Baltimore: Lippincott Williams and Wilkins).
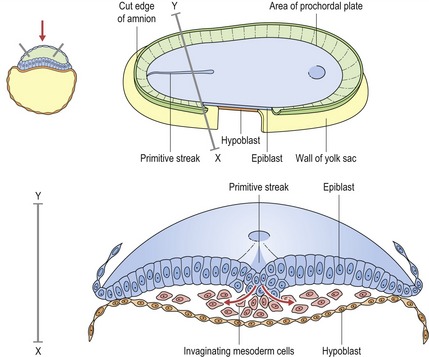
Figure 2.3 Gastrulation.
Redrawn from Sandler TW (ed.) (2003), Langman’s Medical Embryology, 9th edn (Baltimore: Lippincott Williams and Wilkins).
Cranial neural crest cells provide the building blocks for generating much of the skeleton and connective tissue of the head, in addition to the cranial ganglia and peripheral nerves innervating these skeletal structures. In the developing head, neural crest cells arising from the forebrain and midbrain regions populate the upper face, whilst those from the posterior midbrain and hindbrain migrate into the pharyngeal arch system (see Fig. 2.14). Cranial neural crest cells interact with ectodermal and mesodermal cell populations present within these regions, leading to the formation of craniofacial bones, cartilages and connective tissue (Fig. 2.4).
The prechordal plate—a molecular organizer for the brain and face
A region of fundamental importance for early development of the brain and face is the prechordal plate, an area of thickened endoderm that lies beneath the future forebrain of the early embryo (Fig. 2.5).
The prechordal plate acts as a true head organizer, producing molecular signals that pattern the forebrain and subdivide the eyefield into two. In the absence of normal signalling from this region, holoprosencephaly (HPE) or cyclopia can occur. In the most severe cases, the lower forebrain does not form and the upper part remains as a single undivided vesicle, rather than developing into paired cerebral hemispheres (Muenke & Beachy, 2000). This lack of midline development within the central nervous system has important consequences for the face, with a spectrum of facial deformity reflecting the severity of the brain malformation. Failure of forebrain division leads to a lack of separation of the optic primordia and the formation of a single cyclopic eye, which becomes situated below a rudimentary midline facial proboscis or nose-like structure (see Fig. 13.21).
Early organization of the craniofacial region
Primary architecture of the craniofacial region is established during early development and is based upon segmentation (Fig. 2.6). At the future head end of the human embryo, the neural tube is segmented into three vesicles, which will form:
On the lateral side of the head are the pharyngeal arches, which form:
In the lower region of the head is the first pharyngeal arch, which will form:
The pharyngeal arches
In humans there are six pharyngeal arches, which appear progressively during the fourth week of embryonic development. Each arch is covered externally by ectoderm and internally by endoderm, whilst a core of mesodermal tissue exists within. As development proceeds, this central core becomes infiltrated by cranial neural crest cells that migrate into the arches from their site of origin adjacent to the roof of the neural tube. The junction of each arch is in close proximity with its neighbour, producing a pharyngeal cleft of ectoderm externally and a pouch of endoderm internally (Fig. 2.7).
The pharyngeal arches give rise to a number of structures within the head and neck (Fig. 2.8):
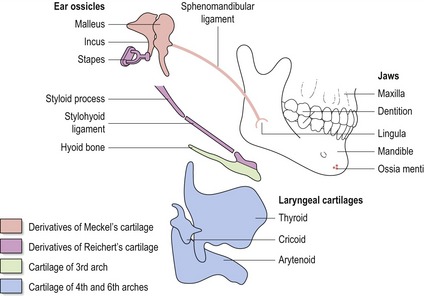
Figure 2.8 Skeletal derivatives of the pharyngeal arches.
Redrawn from Wendell-Smith CP, Williams RL, Treadgold S (1984), Basic Human Embryology, 3rd edn (London: Pitman Publishing).
The embryonic tissue components within each arch give rise to specific structures:
Within the pharyngeal arch system are also characteristic sets of bilaterally symmetrical arteries, nerves and cartilages. Those associated with the first two arches make contributions to the maxillary and stapedial arteries in the adult, whilst those within the lower pharyngeal arches are responsible for generating major arteries within the neck and cardiothoracic region. Neural crest cells that migrate into the third and fourth pharyngeal arches are known collectively as the cardiac neural crest, these cells making an important contribution to remodelling of the pharyngeal arch arteries and to the formation of a functional cardiac outflow tract and cardiothoracic vascular system. Any disruption within the embryonic pharyngeal region can have serious implications for normal development, which is exemplified by a group of related disorders known as the 22q11 deletion syndromes (Box 2.1).
Box 2.1 TBX1: a mediator of normal pharyngeal arch development
T-box genes encode a large group of transcription factors and TBX1 is thought to be a key player in the aetiology of the DiGeorge (DGS; OMIM 601362) and Velocardiofacial (VCFS; OMIM 192430) or 22q11 Deletion syndromes (22q11DS). These syndromes form part of a group of related human dysmorphic disorders that result from deletion or rearrangement of a large region of chromosome 22q11 (Baldini, 2005; Scambler, 2000). 22q11DS subjects are characterized by defects in the cardiac outflow tract and aortic arch, thymic and parathyroid aplasia or hypoplasia, and anomalies of the craniofacial region, which include micrognathia, cleft palate and a characteristic facial appearance. The principle clinical phenotypes present within these syndromes are thought to result from an absence of normal pharyngeal pouch signalling and localized disruption of neural crest migration within the pharyngeal arch system.
Three-dimensional facial scans of individuals with 22q11DS (left) and control subjects (right) aged between 2 weeks and 24 years. The 22q11DS face is only subtly different from the average, but careful examination reveals an exaggerated length of the nose, with a narrow nares and nasal base, but fullness above the tip. The eyes are upslanted and the ears cupped or unusual in shape (Hammond et al, 2005).
Reproduced with kind permission of Professor Peter Hammond, Institute of Child Health, University College London.
The candidacy of TBX1 for 22q11DS has been based upon its location within the deleted region of chromosome 22, its expression domain in endoderm and mesoderm of the developing pharyngeal arches and the finding that mice generated with a targeted deletion in Tbx1 exhibit a spectrum of phenotypic effects encompassing most of the common 22q11DS malformations (Lindsay et al, 2001). More recent genetic experiments in mice, designed to ablate the function of Tbx1 in different regions of the early pharynx at different developmental time points, have provided clues as to the role of this transcription factor during patterning and differentiation of the pharyngeal arch derivatives. In particular, Tbx1 activity influences the proliferation and expansion of endoderm lining the embryonic pharynx; a role that facilitates normal segmentation of the pharyngeal arches and the formation of structures derived from these regions.
Development of the face
Development of the face is a dynamic process, relying upon complex tissue interactions that are closely coordinated, both temporally and spatially (Fig. 2.9). Growth and development of this region is driven by neural crest migration and proliferation, which directs the formation and approximation of a series of swellings or processes. Ultimately, these processes fuse with each other to produce seamless regions of ectoderm and the characteristic features of a face. At the molecular level, a host of signalling molecules, transcription factors and extracellular matrix proteins control the cellular activities underlying these processes (Francis-West et al, 2003).
Human facial development begins at approximately four weeks post conception, with the appearance of five processes, which surround the early oral cavity or stomodeum (Table 2.2):
Frontonasal process |
Forehead including upper eyelids and conjunctiva |
Medial nasal processes |
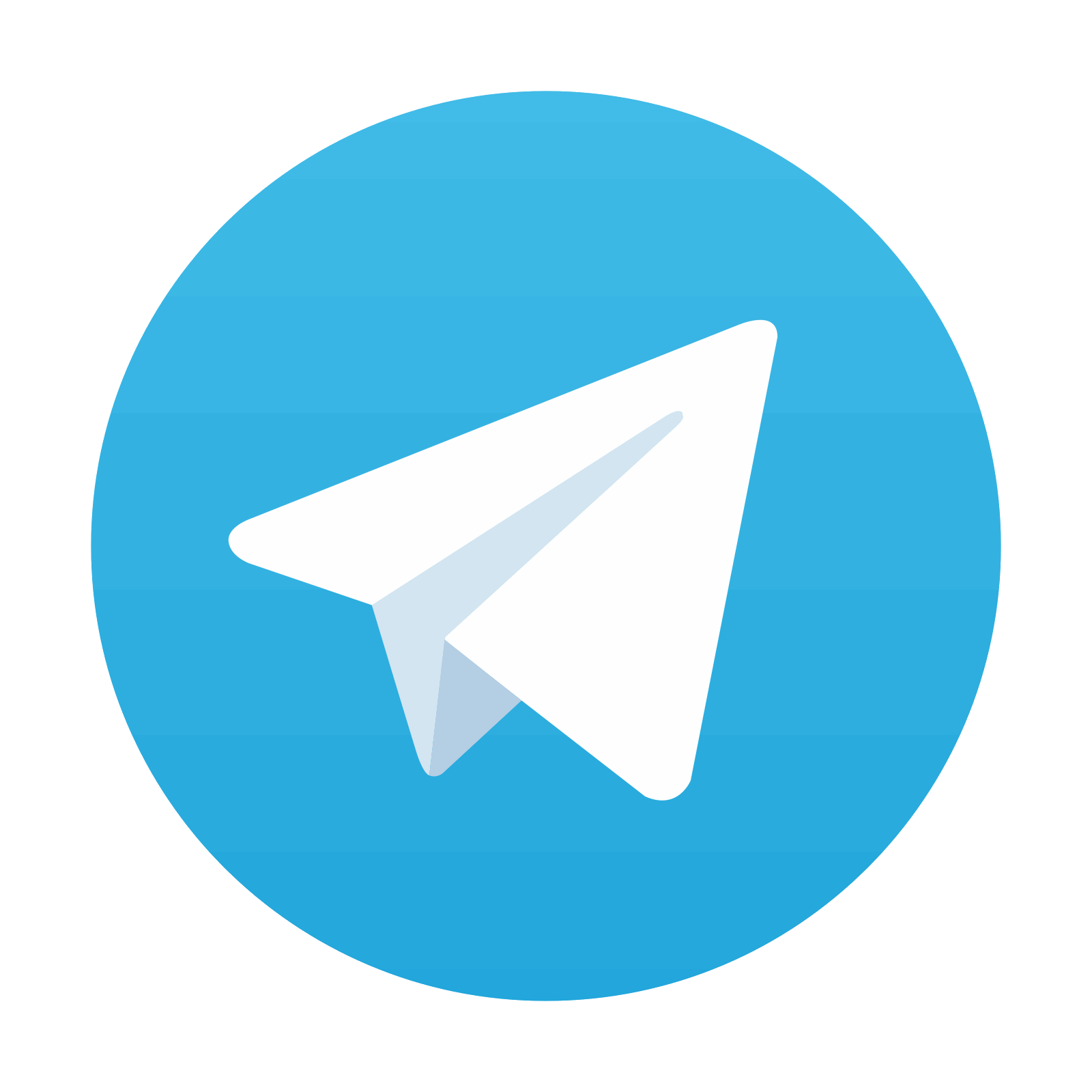
Stay updated, free dental videos. Join our Telegram channel

VIDEdental - Online dental courses
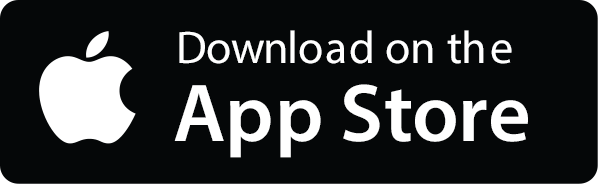
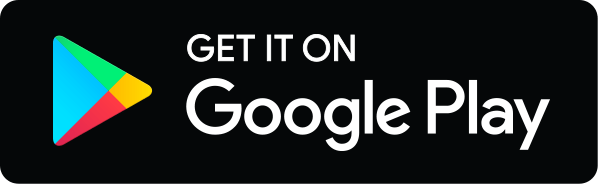