Introduction
The aim of this in-vitro study was to analyze the polymerization capacity of 5 orthodontic composites by determining the degree of monomer conversion (DC).
Methods
Fourier transform infrared spectroscopy was used to evaluate the DC of the orthodontic composites immediately after polymerization and after storage in artificial saliva at 37°C ± 1°C for 30 days. The resin-based adhesive composites investigated were Bisco Ortho (Bisco, Schaumburg, Ill), Heliosit Orthodontics (Ivoclar, Schaan, Liechtenstein), Kurasper F (Kuraray, Okayama, Japan), Light Bond (Reliance Orthodontic Products, Itasca, Ill), and Transbond XT (3M Unitek, Monrovia, Calif), cured with Elipar FreeLight 2 (3M ESPE, St Paul, Minn) for the testing of the DC values. Fifty cylindrical specimens were manufactured in molds. The data were analyzed by 2-factor analysis of variance (ANOVA) and Tukey HSD test.
Results
According to 2-way ANOVA, the DC was significantly influenced by composite type ( P <0.05); after 30 days, there were no differences among the composite types for the DC. The interaction of orthodontic composites and time played a statistically significant role in the DC ( P <0.05), but there was no statistically significant influence of time for the DC ( P >0.05).
Conclusions
The DC was found to change according to composite materials used, and Bisco Ortho showed the most DC performance. The DC of orthodontic composites is a complex process that is affected not only by inorganic filler content of the composite but also the monomer type and many other factors. Sufficient DC values of 5 commercially available orthodontic composites can be achieved with a new-generation light-emitting diode curing light.
The evolution of dental restorative or adhesive resin materials has been impressive in recent years. In the orthodontic field, a wide variety of adhesive resin materials has been developed as bonding composites. Although the developments and improvements of the orthodontic composite materials are satisfying and amazing, an inadequate degree of conversion (DC) of the orthodontic composites still is a problem for orthodontists. In addition, few data are available in the literature for the DC of commercial orthodontic composites and also about the length of time after light polymerization.
Inadequate polymerization of the resin matrix of orthodontic composites results in inferior physico-mechanical properties and clinical performance, such as poor bond strength and color stability, and increased rates of water sorption, solubility, and degradation. Resin matrices of orthodontic composites consist of mainly 2 monomers: bisphenol A diglycidyl dimethacrylate (Bis-GMA) and triethylene glycol dimethacrylate (TEG-DMA). The DC of orthodontic composites is also important, since resins can release Bisphenol A, a Bis-GMA precursor that exhibits estrogenicity and cytotoxicity with resulting potential adverse biologic reactions. Therefore, adequate polymerization is a crucial factor in obtaining optimal physico-mechanical properties and satisfying clinical performance of composites.
Ideally, dental restorative or adhesive resin would have all of its monomer converted to polymer during the polymerization reaction. However, all dimethacrylate monomers exhibit considerable residual monomer in the final product, with a DC ranging from 55% to 75% under conventional irradiation conditions. The results of Eliades et al showed DC values of 48% to 68%, and the results of Usumez et al showed DC values of 36% to 67% for some commercially available orthodontic composites. Clinically, therefore, inadequate polymerization would be such a problem, because of difficulties in illuminating the composite evenly from each side of the bracket and reaching everywhere of the oral cavity with the light source.
The reason for this is believed to be mainly the limitations on the mobility of reactive groups imposed by the rapid formation of a cross-linked polymeric network. For light-cured resin composites, the DC of resin composites depends on the chemical structure of the monomer, filler size, shade and translucency, sufficient light intensity, curing time, distance from the light source, thickness of the increment, depth of cure, and polymerization conditions, including atmosphere, temperature, and photoinitiator concentration.
But the relative contributions of these variables to the DC are still unknown. However, this information might be essential for the development of new strategies to increase the DC. In the literature, there are few investigations about the DC values of orthodontic composites; in most of them, the DC values were measured at 24 hours, but there were no comparisons of differences after 1 month. The information obtained from comparisons might be essential for the development of new strategies to increase the DC. The DC values obtained from these procedures were compared with Fourier transform infrared spectroscopy (FTIR) (1600 series, PerkinElmer, Wellesley, Mass). The objective of this study was to investigate whether both composite resins and storage for a definite period would affect the DC. The null hypotheses tested were that there would be statistically significant differences in the DC of the various orthodontic composites after illumination and that the DC values would increase with time.
Material and methods
The resin-based orthodontic composites investigated were Bisco Ortho (Bisco, Schaumburg, Ill), Heliosit Orthodontic (Ivoclar Vivadent AG, Schaan, Liechtenstein), Kurasper F (Kuraray Medical, Okoyama, Japan), Light Bond (Reliance Orthodontic Products, Itasca, Ill), and Transbond XT (3M Unitek, Monrovia, Calif) ( Table I ). The Elipar FreeLight 2 (3M ESPE, St Paul, Minn) was the light-curing device, with a tip of 8 mm and a light intensity of 1200 mW/cm 2 for preparing the light-polymerizing specimens. Fifty cylindrical specimens (10 for each adhesive group), 6 mm in diameter and 1 mm in depth, were manufactured in molds made of synthetic fluorine-containing resins and placed between glass slides (3 mm) at room temperature. The manufacturers’ instructions were used for preparing, handling, and polymerizing the materials. These slides were gently pressed together to remove excess test material. Standardization of the distance between the light source and the specimen was provided by the thickness of the glass slide, and the glass slides also provided a smooth surface for the testing process. The specimens were divided into 2 group (n = 5) for all brands of composites. The DC values of the first groups were measured immediately after curing, and the DC values of the second groups were measured after 30 days by FTIR. The second groups of specimens were stored in light-proof boxes after the curing procedure to prevent further exposure to light and in artificial saliva at 37°C ± 1°C.
Brand | Basic Ingredients | Filler weight (average %) | Company and lot number |
---|---|---|---|
Bisco Ortho | Bis-GMA, TEG-DMA, and fused silica | 67.5 | Bisco, Schaumburg, Ill Lot: 0600004598 |
Heliosit Orthodontic | Bis-GMA, UDMA, decamethylen-DMA, silicon dioxide, catalysts, and stabilizers | 15 | Ivoclar Vivadent AG, Schaan, Liechtenstein Lot: G21064 |
Kurasper F | Bis-GMA, TEG-DMA, silanated barium glass filler, colloidal silica, catalysts, dl-camphorquinone, and accelerators |
73 | Kuraray Medical, Okoyama, Japan Lot: 41230 |
Light Bond | Bis-GMA, silica-crystalline, silica, fused amorphous silica, and sodium fluoride |
95 | Reliance Orthodontic Products, Itasca, Ill Lot: 0609234 |
Transbond XT | Bis-GMA , Bis-EMA, silane-treated quartz, and silane-treated silica | 77.5 | 3M Unitek, Monrovia, Calif Lot: 6CX6WM0088 |
FTIR was used to evaluate the DC. After curing and 30 days of storage, each specimen was pulverized into a fine powder with a mortar and pestle. Fifty micrograms of the ground powder was mixed with 5 μg of potassium bromide powder (Carlo-Erba Reagenti, Milan, Italy) and pressed to produce thin potassium bromide discs with a press machine. After that, the absorbance peaks were recorded by using the diffuse-reflection mode of FTIR. Scan range was 4400 to 4450 cm −1 , and the scanning speed was 60 cm −1 per minute for the FTIR spectra. The spectral resolution for this work was 16.0 cm −1 . Spectra were also acquired from the same number of unpolymerized adhesives. For this, unpolymerized pastes were smeared onto thin potassium bromide discs. The amount of double vinyl bonds remaining in the specimen exposed to irradiation is shown by the intensity of the peak at 1637 cm −1 referring to the carbon-carbon double bond (C=C) stretching of the vinyl group; this has been used in the study of polymerization of acrylates and methacrylates. The DC was directly related to the decrease of 1637 cm −1 absorption on the FTIR spectra as follows:
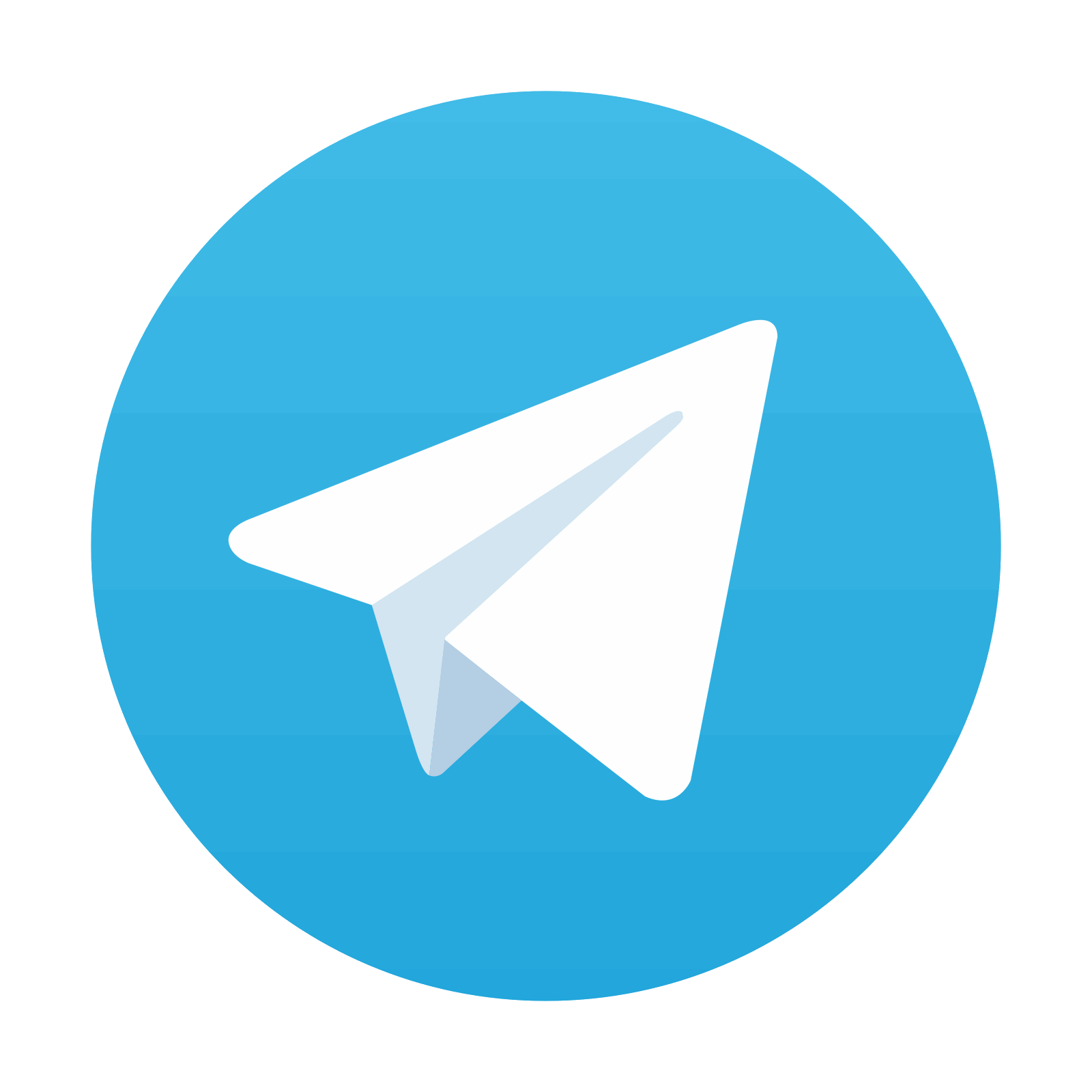
Stay updated, free dental videos. Join our Telegram channel

VIDEdental - Online dental courses
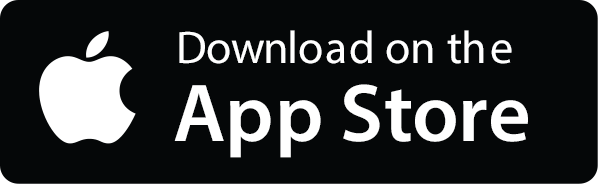
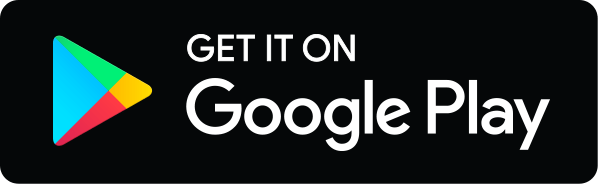