Highlights
- •
Initial polymer structure; influenced by photo-polymerisation variables, impacts the thermal response of resin matrices.
- •
Systems polymerised rapidly are more influenced by thermal perturbation.
- •
Accelerating polymerisation confers reduced chain extension below the glass transition temperature.
- •
Slower polymerised systems display greater relative order before and after the glass transition point.
Abstract
Objective
The structure of the polymer phase of dental resin-based-composites is highly sensitive to photo-polymerisation variables. The objective of this study was to understand how different polymer structures, generated with different photo-polymerisation protocols, respond to thermal perturbation.
Methods
Experimental resins were prepared from a series of Bis-GMA/TEGDMA blends (40/60, 50/50 and 60/40 wt.%), with either Camphorquinone/DMAEMA or Lucirin TPO as the photo-initiator system. Resins were photo-polymerised, in a disc geometry, at either relatively ‘high’ (3000 mW cm −2 for 6 s) or ‘low’ (300 mW cm −2 for 60 s) irradiances ensuring matched radiant exposures (18 J cm −2 ). Specimens were heated, from 20−160 °C at a rate of 5 °C min −1 , whilst simultaneous synchrotron X-ray scattering measurements were taken at 5 °C increments to determine changes in polymer chain segment extension and medium-range order as a function of temperature. For each unique resin composition (n = 3), differential scanning calorimetry was used to measure glass transition temperatures using the same heating protocol. A paired t-test was used to determine significant differences in the glass transition temperature between irradiance protocols and photo-initiator chemistry at ɑ = 0.05.
Results
Resins pre-polymerised through the use of TPO and or high irradiances demonstrated a reduced rate of chain extension indicative of lower thermal expansion and a larger decrease in relative order when heated below the glass transition temperature. Above the transition temperature, differences in the rate of chain extension were negligible, but slower converted systems showed greater relative order. There was no significant difference in the glass transition temperature between different photo-initiator systems or irradiance protocols.
Significance
The evolution of chain extension and medium-range order during heating is dependent on the initial polymer structure which is influenced by photo-polymerisation variables. Less ordered systems, generated at faster rates of reactive group conversion displayed reduced chain extension below the glass transition temperature and maintained lower order throughout heating.
1
Introduction
Photo-initiated dimethacrylate resin-based-composites used as dental restorative materials are subject to physical [ , ] and chemical perturbations [ , ] during their clinical service lifetime that are likely to alter the macro and micro structure of the resin phase. In vivo , a dental composite filling will experience an average intra-oral temperature of approximately 37 °C when no other thermal stimulus is applied [ , ]. However, eating, drinking and breathing induces intra-oral thermal fluctuations which have been shown, using thermocouples, to range between 4−60 °C [ , ] and will be partially transferred to the composite filling material. The thermal response of the resin phase will be influenced by the chemical structure of the constituent monomers [ ], the degree of reactive group conversion and the polymer network structure. The formation of the polymer structure itself has been shown to be highly sensitive to photo-polymerisation variables [ ], especially the reactive group conversion rate [ ]. Previous research has investigated the dynamic response of composites to thermal perturbations by measuring dimensional changes [ , ], performing mechanical tests [ , ], quantifying reactive group conversion and conducting thermal analyses during heating [ ]. Assumptions have then been extrapolated about the evolving polymer structure and correlated with the initial compositional and photo-polymerisation variables. However, these approaches have failed to provide detailed insight into how the initial polymer structure changes at medium-range orders (∼ 4−15 Å) during heating, which will ultimately impact on physical changes in the bulk material. The current study explores for the first time how different initial polymer structures in the most commonly encountered photo-initiated dental resin polymers, generated under different photo-polymerisation protocols, are modified under thermal perturbation.
Studies have demonstrated that altering polymerisation variables, specifically by accelerating the rate of reactive group conversion using high irradiance light sources and by using more efficient photo-initiators, impacts the resultant polymer structure at a range of length scales [ ]. Combined experimental and theoretical studies have suggested that during rapid polymerisation, the conversion rate substantially exceeds the relaxation time [ , ] of constituent polymer structures. Consequently, this likely results in the jamming and clustering of growing chains, preventing efficient relaxation of the developing structure and produces a more rigid network [ ] than obtained at lower polymerisation rates. Theoretical approaches [ ] have demonstrated that faster polymerisation generates a polymer network with significantly greater clustering i.e. with greater spatial heterogeneity with respect to cross-link density, compared with slower rates, where reactive group conversion is more evenly distributed throughout the polymer [ ]. Additionally, rapid polymerisation reduces the lifetime of propagating radical species due to bimolecular termination [ ], limiting the degree of cross-linking. Recently, we have demonstrated that the conformation of polymer segments at medium-range orders, particularly the functional groups of dimethacrylate polymers, are sensitive to the conversion rate [ ]. Accelerating reactive group conversion confers greater polymer segment extension in a shorter period of time. The evolving structure has insufficient time to relax and orientate to lower energy conformations [ ] and consequently greater structural variability (lower order) exists in the network at medium-range length scales. Insight into the conformation of polymer segments at medium-range orders is important as these structures are constituent in cross-links and chain backbones, which play a role in determining second order phase transitions i.e. the glass transition, of the material.
Dimensional changes in the polymer phase of dental composites during heating are predominantly ascribed to structural modifications of the polymer network as the system reaches the glass transition ( T g ) region. Below T g the polymer network structure remains rigid, restricting polymer segmental motions [ ] and demonstrates glass-like behaviour [ , ]. As the system transitions through T g chain molecular translations and rotations increase and the bulk material becomes rubbery [ ]. To date, several studies have investigated the use of post-cure heating to increase the degree of reactive group conversion [ , ] to improve the mechanical properties of the composite material. Vibrational spectroscopy [ , ] and differential scanning calorimetry (DSC) are routinely used to measure additional cure. DSC also provides thermal information regarding phase transitions, heat and degradation and allows for differences in network architecture to be indirectly inferred based on the thermal response of the polymer as a function of the initial polymerisation variables. However, neither approach provides dynamic structural information of medium-range ordering, relevant to second order phase transitions, during heating. Synchrotron X-ray scattering is a powerful technique that can discern subtle structural differences within a dynamic system. Furthermore, a high flux permits time resolved measurements at time scales of the order of heating rates commonly used in conventional thermal studies of polymers [ ], which would not be possible with a laboratory X-ray source.
The aim of this study was to explore how different initial polymer structures, generated by using different photo-polymerisation protocols that impact on the polymerisation reaction rate, are modified by thermal perturbation through a combination of heating, in-situ X-ray scattering and offline differential scanning calorimetry measurements. Specifically, the hypothesis tested was that, based on previous investigations, there would be a difference in polymer chain segment extension and medium-range order as a function of temperature between systems initially polymerised at different conversion rates.
2
Methods
2.1
Preparation of photo-polymerisable dimethacrylate resin blends
Two dimethacrylate monomers, bisphenol-A-glycidyl-methacrylate (Bis-GMA) and triethyleneglycol-dimethacrylate (TEGDMA) (Sigma-Aldrich, Dorset, UK) were proportioned in 60/40, 50/50 and 40/60 (Bis-GMA/TEGDMA) weight percentage (wt.%) ratios and combined to produce mixes. The viscosity of the blend increases with the proportion of Bis-GMA [ ] (see Supplementary material, Fig. S1). The monomer blends were combined with a photo-initiator as either 0.2 wt.% Camphorquinone (CQ) with 0.8 wt.% of its tertiary amine N,N -dimethylaminoethyl-methacrylate (DMAEMA) or 1 wt.% of the Type 1 photo-initiator, Lucirin-TPO (TPO) (Sigma-Aldrich, Dorset, UK), which does not require a co-initiator. The proportioned monomers and photo-initiators were homogenised in a glass beaker using a magnetic stirrer at 50 ± 1 °C for 30 min in dark conditions. The resultant resin blends were stored in dark conditions in sealed containers at 4 ± 1 °C prior to further use. Resin disc-shaped specimens were fabricated by filling a polyvinylsiloxane mould (11 mm diameter and 1 mm in depth) covered with a 1 mm thickness glass slide to minimise the formation of an oxygen inhibition layer. Specimens were photo-polymerised using an EMS Swiss master light curing unit (EMS OPTIDENT, electro medical systems, Nyon Switzerland) placed normal to and in contact with the glass slide. The curing unit has a spectral output range of 390–550 nm to provide significant overlap with the absorption peaks of the Camphorquinone (470 nm) and Lucirin TPO (381 nm) photo-initiators. For each unique composition, the resin was either photo-polymerised at relatively ‘high’ (3000 mW cm −2 for 6 s) or ‘low’ (300 mW cm −2 for 60 s) irradiances to introduce a range of reactive group conversion rates whilst ensuring matched radiant exposures (18 J cm −2 ). The resultant specimens were fabricated two weeks prior to any measurements and were stored in lightproof conditions in sealed containers at 4 ± 1 °C to remove post-cure variability attributed to further light activation [ , ].
2.2
FT-NIR spectroscopy measurements
Fourier Transform Near Infra-red (FT-NIR) spectroscopy was used to quantify the rate of reactive group conversion between relatively high (3000 mW cm −2 ) and low (300 mW cm −2 ) irradiances to confirm differences in rate as a function of irradiance protocol, for selected monomer blends, according to a previously reported method [ ]. Briefly, optical (emitting and receiving) fibers (0.6 mm core diameter) (Hellma Analytics, Essex, UK) were placed either side of the liquid resin sample, which was held within a polyvinylsiloxane annulus (11 mm diameter and 1 mm in depth), at a 45° incline relative to the sample face and connected to a Nicolet 6700 spectrometer (Thermo Scientific, Warrington, UK). During photo-polymerisation, NIR spectra were collected in transmission mode using a white light source and an InGaAs detector. Real-time NIR spectra (4000–10,000 cm −1 ) were collected 10 s prior to irradiance and thereafter during polymerisation in transmission mode (4 cm −1 spectral resolution) with an integration time of 0.3 s, whilst photo-polymerising the resin sample according to protocol described in Section 2.1 . Three measurements were conducted for each unique blend for both irradiance protocols. Data were baseline corrected using Omnic software (Omnic Specta software, version 8.0, Thermo Fisher Scientific, Oxford, UK). Reactive group conversion was determined from the percentage decrease in the aliphatic C CH 2 IR absorption band (6164 cm −1 ) i.e. the conversion of C C to C C bonds, located within the functional end groups of the Bis-GMA and TEGDMA monomers. The rate of conversion was calculated as the first derivative of conversion with respect to time.
2.3
Synchrotron X-ray scattering measurements
Synchrotron X-ray scattering experiments were performed on the I16 beamline [ ] at the Diamond Light Source (Oxford, UK). An incident X-ray energy of 12 keV was used corresponding to a wavelength ( λ ) of 1.033 Å, with a beam size of 30 μm (vertical) × 200 μm (horizontal) defined by vacuum tube slits. Measurements were carried out in air with light excluded.
A bespoke heating module was used to increase the temperature of pre-polymerised Bis-GMA/TEGDMA resin disc specimens produced according to the previously described methods, whilst undertaking simultaneous X-ray scattering measurements ( Fig. 1 ). The heating module consisted of two aluminium plates (25 × 25 mm area) with a circular aperture (3 mm diameter), to allow for the transmission of X-rays, fixed in contact with and perpendicular to a kinematic heating platform. The temperature of the heating platform was remotely controlled via a cryogenic temperature modulator (Model 3.8, Lake Shore Cryotonics, Elliot Scientific Ltd, Hertfordshire, UK). Polymer disc specimens were held in tight contact between two aluminium plates. The temperature of the test specimen was increased through heat transfer from the kinematic stage to the aluminium plates and finally to the resin disc, at a rate of 5 °C min −1 . Three thermocouples were placed in contact with the kinematic stage, aluminium plates and the resin disc respectively, to measure the temperature of each component and ensure a constant heating rate. The heating module and housed sample were orientated with the annulus of the aluminium plate normal to the path of the impinging X-rays.

X-ray scattering measurements were taken at 5 °C increments, from 20 to 160 °C, to characterise structural changes as a function of temperature. X-ray scattering data were collected using a 2D area detector (Pilatus 100 K) with a 487 × 195 pixel format (pixel size = 172 × 172 μm 2 ) fixed to a two theta detector arm. The detector was placed 470 mm behind the sample and collected scattering data at angles of 2 θ = 7, 13 and 20° to cover a q range of 0.2 to 1.8 Å −1 where q = 4πsinθ/λ. Scattering data were collected with a 1 s count time and a 2.7 ms readout time. The transmitted beam was also recorded at each angle and temperature increment using a photodiode to correct for changes to the sample density and thickness. Due to the high user demand of synchrotron facilities which limits the duration of experiments, measurements for each unique sample composition were not repeated.
The contribution of the X-ray beam to the measurements i.e. beam damage was assessed by conducting observations for over 30 min for the pre-polymerised samples at ambient temperature. To aid data analysis, measurements were taken for direct beam, empty sample containers and a silver behenate calibration standard. Scattering patterns were background corrected and normalised to the incident monitor intensity. Data were integrated over 360° to produce a 1D output and were subsequently fitted with a pseudo-Voigt model to determine peak positions and changes in the peak full width at half maximum (FWHM) as a function of temperature using the Dawn software package (version 2.6.0) [ , ]. The peak position(s) correspond to the measured average correlation length(s) whilst the relative structural order representing the distribution of correlation lengths was calculated from changes in the FWHM of the scattering peak(s).
2.4
Thermal analysis
To correlate changes in X-ray scattering during heating with structural transitions, the glass transition temperature ( T g ) and post-cure exotherm for polymerised resin specimens were measured using a differential scanning calorimeter (DSC Q1000, TA Instruments, Ontario, Canada). Samples (n = 3, per composition) of approximately 5 mg were heated, within hermetically sealed aluminium pans (DSC Consumables Incorporated, Minnesota, USA), through a scanning range of 20−160 °C ± 0.05 °C at a rate of 5 °C min −1 under a nitrogen atmosphere. The T g values were obtained from the inflection point in the thermogram (Universal Analysis 2000, TA Instruments, Ontario, Canada), as measured from the extensions of the pre- and post-transition baselines. Reaction exotherm was obtained from the integrated area of exothermic peaks in the thermogram (Universal Analysis 2000, TA Instruments, Ontario, Canada).
2.5
Statistical analysis
For each resin monomer composition, a one tailed t-test was used to determine differences in the rate of reactive group conversion between identical blends polymerised at identical irradiances, for samples using either CQ or TPO as photo-initiator. A one-way ANOVA and post-hoc Tukey tests ( α = 0.05) were also used to identify differences in T g and exotherm values between samples photo-polymerised at different conversion rates. Descriptive analysis was conducted on X-ray scattering data and qualitatively correlated with DSC data to aid interpretation. All statistical analysis was performed in the R programming language (version 3.1.3, 2015, R Foundation).
3
Results
3.1
De-convolving the scattering signal
Fig. 2 (a) shows representative 1-D X-ray scattering spectra for the same sample (40/60 wt.%, Bis-GMA/TEGDMA, TPO photo-initiator, irradiance = 3000 mW cm −2 ) at 20 °C and 160 °C. A broad scattering feature is located at 2 θ ∼ 10° and shifts to lower scattering angles with increasing temperature. Deconvolution of the X-ray scattering spectrum ( Fig. 2 (b)) reveals a high-agreement fit for two scattering peaks located at 2 θ ≈ 10.3° ± 0.0045° and 17.4° ± 0.036°, corresponding to correlation lengths in real space of approximately 5.55 Å and 3.43 Å respectively. When the resin is incrementally heated, the principle scattering peak located at 10.3° shifts to lower values of 2 θ , indicative of an increase in the correlation length. Additionally, the width of the peak fluctuates as a function of temperature indicating temperature dependent changes in medium-range order. The scattering feature at ∼17.4° displays a small shift to wider scattering angles upon heating, corresponding to a decrease in this correlation length, whilst the FWHM value increases linearly (see Supplementary material, Fig. S2).

3.2
The effect of temperature on correlation length
Fig. 3 illustrates the relative change in the principle correlation length for all resin samples as a function of temperature, where the correlation length corresponds to the peak position of the scattering feature at 2 θ ∼ 10.3° in Fig. 2 at each temperature increment. It can be seen that all samples demonstrate a subtle two stage increase in the relative change in the correlation length, with an inflection point located at ∼45−55 °C, as the temperature is increased. Prior to the inflection point, the relative change in the correlation length is approximately linear with respect to temperature and the rate of increase is 0.0110 ± 0.0048 and 0.0076 ± 0.0011 % °C −1 for the CQ and TPO based systems, respectively. After the inflection point, the rate of relative change in the correlation length increases for all samples and remains linear with values of ∼0.025% °C −1 (CQ = 0.0248 ± 0.0038 % °C −1 , TPO = 0.0250 ± 0.0018 % °C −1 ), regardless of differences in the co-monomer ratio, photo-initiator chemistry or the irradiance protocol used. The total relative increase in the correlation length i.e. between the highest and lowest temperature values is ∼3 % for all samples except for ‘40/60 wt.% 300 mW cm −2 (CQ)’, which shows larger initial and final gains in the correlation length during heating, however the steady state percentage increase in correlation length is similar to other resins. Differences between CQ and TPO initiated systems for the rate and total relative change in the correlation length are negligible after the inflection point.
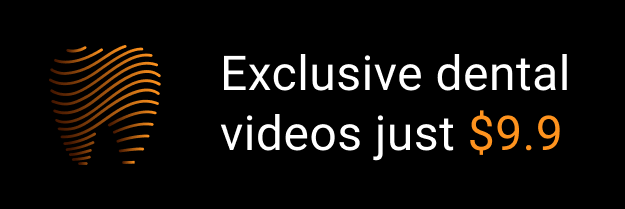