The primary goal of implant dentistry is to restore patients to oral health and full dental function. Often the practitioner has a patient that may have a suboptimal healing outcome. Drugs that the practitioner may use during implant or grafting surgeries can have an impact on the success of these procedures. Likewise, medications that the patient may be taking can also have a positive or negative effect on treatments. Patients desire success, and the clinician can optimize treatments by using pharmacologic adjuncts to increase the probability of attaining that goal. Pharmacologic treatments can be systemic or localized and may be of benefit during the perioperative phase or later. The guiding principles when choosing a pharmacologic adjunct should always aspire to meet the following criteria:
- 1.
There should be a justifiable indication for each medication that is used.
- 2.
Each medication should be used at the lowest effective dose and for the shortest possible period of time.
- 3.
Whenever possible, the selection of a medication regimen should be based on evidence obtained from controlled clinical trials.
- 4.
The clinician must understand that dose (or concentration), route of administration, dosing frequency, and duration are critical pharmacokinetic factors that determine if a treatment is beneficial or detrimental to the success of an implant or bone grafting procedure.
All pharmacologic agents should have their use based upon scientific principles and a proven record of increasing the rates of short- and long-term success.
This chapter will review the following:
- 1.
The rationale supporting the addition of antibiotics to bone grafting materials
- 2.
The proper prescribing of prophylactic antibiotics in relation to implant placement and bone grafting
- 3.
Pharmacologic management of periimplantitis (PI)
- 4.
The effect of bisphosphonates (BPs) on dental implants, with guiding science-based principles for managing the dental implant patient receiving these drugs
- 5.
The effect of mineral, hormonal, and vitamin supplements on dental implants
- 6.
The effects that commonly used nonsteroidal antiinflammatory drugs have on bone healing and development
- 7.
The effect bioactive fatty acids have on bone development
Each of these issues has a varying impact on treatment success and should be considered as warranted by the clinical situation.
▪
ANTIBIOTIC PROPHYLAXIS IN IMPLANT DENTISTRY
Antibiotic (antimicrobial) surgical prophylaxis is often misunderstood and used incorrectly. The true definition refers to the prevention of infectious complications following surgical procedures. The shortest possible duration of antibiotic administration should be used to minimize the risk of serious adverse effects, the development of antibiotic resistance, or the proliferation of nonsusceptible bacteria. The goal of surgical antimicrobial prophylaxis is to achieve sufficient antibiotic tissue concentration before possible contamination of the relevant tissues of the oral cavity and to ensure adequate levels throughout the operative procedure to prevent subsequent bacterial growth. This involves high blood and tissue antibiotic concentrations at the time of surgery, when contamination is possible, followed by a reduction to no antibiotic within 24 hours. Regrettably, antibiotic coverage is often begun 1 day preoperatively and extended for several days postoperatively. This practice has led to unnecessary antibiotic use. The probability of negative side effects and the development of resistant bacteria increase with longer durations of antibiotic administration.
Controversy surrounds the need for presurgical antibiotic prophylaxis with dental implant surgical procedures. It is accepted that antibiotic prophylaxis is recommended for those patients at high risk for endocarditis, as recommended by the American Heart Association (AHA) guidelines, or patients with one or more of the following: immune deficiencies, metabolic diseases, history of irradiation in the head and neck area, and when an extensive or prolonged surgery is anticipated. The overriding question is does surgical antibiotic prophylaxis affect dental implant and/or bone grafting success? Osseous integration and/or bone graft success can be jeopardized by the presence of bacteria in the tissues and the concomitant inflammatory reactions.
Laskin et al. (2000) examined 2973 implants at each stage of implant placement and restoration and correlated the findings with implant failure or loss of osseous integration, up to 36 months after placement. The results demonstrated a significantly higher survival rate at each stage of treatment in patients who had received preoperative prophylactic antibiotics. A previous study examined implant success only to the time of uncovering and found that significantly fewer failures occurred when preoperative antibiotics were used. These findings were contrary to those of Morris et al. (2004) who examined the effect of prophylactic antibiotic coverage with 1500 implants for up to 5 years and found that the use of antibiotics provided no beneficial effects. None of these studies were randomized controlled clinical trials (RCTs), but rather retrospective analyses. The Cochran Database System Review of 2003 found that no RCTs had been reported and concluded that there is no appropriate scientific evidence to recommend or discourage the use of prophylactic systemic antibiotics to prevent complications and failures of dental implants in healthy patients.
The question of single-dose preoperative antibiotic coverage versus long-term postoperative coverage has been addressed. Kahani et al. (2005) conducted a retrospective study comparing implant survival rates following a single-dose preoperative antibiotic regimen with that of a 7-day postoperative antibiotic protocol. They found no significant differences in complication incidence and implant survival rates between the two groups. Therefore, it was concluded that a single-dose prophylactic antibiotic regimen with routine implant procedures would be best. A prospective study was performed by Binahmed et al. in 2005 comparing the efficacy of a prophylactic single-dose antibiotic and a 7-day postoperative antibiotic regimen. No advantage or benefit was found with long-term antibiotic coverage over single-dose preoperative antibiotic regimens.
When considering antibiotic prophylaxis for bone grafting procedures, a prospective placebo-controlled double-blind clinical trial revealed that there was a statistically significant increased risk of having an infectious complication without antibiotic prophylaxis. Phenethicillin 2 g was administered 1 hour preoperatively to the treatment group. Another prospective randomized study involving 124 patients compared the effects of a single 600-mg dose of clindamycin versus clindamycin 600 mg preoperatively and 300 mg every 6 hours for 24 hours postoperatively. It was concluded that there was no statistically significant difference between the single-dose and the 24-hour regimen in connection with postoperative infection in patients undergoing bone grafting procedures.
Clindamycin 600 mg was compared with the beta-lactam phenethicillin 2 g 1 hour preoperatively for bone grafting procedures. It was determined that there was no significant difference between groups regarding postoperative infection.
A suggested treatment protocol for routine implant or bone grafting surgeries in the healthy individual without medical complications would be clindamycin 600 mg either intravenously, intramuscularly, or orally 1 hour preoperatively. The scientific evidence available at this time does not support the use of long-term postoperative therapy. The literature is divided on the impact that prophylactic antibiotic therapy has on implant or grafting success, therefore accounting for the varying opinions on possible benefits. Historically, there has been reluctance by some practitioners to not use clindamycin because of concern regarding potential adverse events, in particular the development of Clostridium difficile diarrhea or pseudomembranous colitis. Reviews of the risk factors for C. difficile infection associated with clindamycin reveal that this concern is unwarranted. C. difficile -associated problems are rare when clindamycin is used in outpatient ambulatory care settings. This unwarranted misconception about clindamycin should be acknowledged, and the true value of clindamycin as a prophylactic antibiotic should be recognized. Clindamycin’s broad-spectrum activity against aerobic, anaerobic, and β-lactamase–producing pathogens plus its high oral absorption, significant soft and bone tissue penetration, and stimulatory effects on the host immune systems make it an excellent choice for antibiotic prophylaxis ( Box 23-1 ).
Preoperative antibiotic prophylaxis:
For patients not allergic to clindamycin (Cleocin): Clindamycin 150 mg
Sig: Take four capsules 1 hour before dental implant surgery
▪
ANTIBIOTICS ADDED TO BONE GRAFTING MATERIALS
It has become common practice to empirically add antibiotics to autogenous, allograft, or xenograft bone grafting materials. Unfortunately, a variety of anecdotal antibiotic bone grafting cocktails has been advocated, with little scientific evidence for their use. The blood supply to a recent bone graft is compromised, and therefore systemic use of antibiotics for either prophylaxis or treatment of an infected bone graft site may be insufficient to provide adequate antibacterial concentration levels. There is great interest in both orthopedics and implant dentistry for the local administration of antibiotics. Locally administered antibiotics may achieve a twenty-fold higher concentration in a contained area versus intravenous administration. This local administration is seen to offer promise in both prevention and treatment of localized osseous infections. Sanders et al. in 1983 reported that bone grafts containing antibiotics had greater predictability than those not containing antibiotics. Mabry et al. reported that the combination of gentamicin and tetracycline mixed with freeze-dried bone allografts increased osseous regeneration. Other studies have shown that the addition of antibiotics to graft material had a depressive effect on bone formation. These conflicting reports may be due in part to potential toxic effects of various antibiotics and their localized concentrations. Studies have demonstrated that some antibiotics are toxic to osteoblast-like cells, especially at higher concentrations. During the initial healing process of the bone graft, osteoblasts are present along with human mesenchymal stem cells (hMSCs), which migrate into the bone graft, where they proliferate and differentiate. If these progenitor cells should fail to mobilize, proliferate, or differentiate, a failure in bone development may occur. Knowing that high concentrations of antibiotics have influence on bone-forming cells and that anaerobes would most likely be the bacteria of concern, the clinician should consider these factors when making a choice of which antibiotic to add to the bone graft. Cavalier use of an inappropriate locally administered antibiotic may lead to toxic effects and result in the failure of the bone graft.
Duewelhenke et al. investigated the effect of 20 antibiotics from different classes and antibacterial mechanisms in cell cultures of primary human osteoblasts (PHO). The mean inhibitory concentrations (ICs) (IC 20 , IC 50 ) for proliferation and metabolic activity were compared ( Table 23-1 ). Cytotoxicity could not be observed 24 hours after treatment of PHO with inhibitors of bacterial cell wall synthesis, aminoglycosides, tetracycline, rifampin, and lincomycin at any concentration. Aminoglycosides and antibiotics that inhibit bacterial cell wall synthesis, with the exception of higher concentrations of cefazolin, did not have an effect on proliferation or metabolic activity. Cefazolin decreased the proliferation of PHO at higher concentrations. Clindamycin and erythromycin demonstrated cytotoxicity at higher concentrations, but not at lower concentrations. At ultralow concentrations, clindamycin and erythromycin did not affect proliferation or metabolic activity, but did have an effect at moderate to high concentrations. Azithromycin and roxithromycin demonstrated cytotoxicity, inhibited proliferation, and decreased metabolic activity even at lower doses.
Antibiotic | Mean IC (Proliferation, Metabolic Activity; m g/mL) | |
---|---|---|
IC 20 | IC 50 | |
Cefazolin | 380, >400 | >400, >400 |
Lincomycin | No effect | No effect |
Clindamycin | 30, 340 | 150, >400 |
Erythromycin | 30, 210 | 180, >400 |
Azithromycin | 20, 80 | 25, 160 |
Roxithromycin | 20, 110 | 70, 210 |
Ciprofloxacin | 70, 260 | 170, >400 |
Moxifloxacin | 80, 190 | 160 >400 |
Tetracycline | 40, * | 180, * |
* Could not be determined as a result of inference with assay.
The fluoroquinolones (ciprofloxacin and moxifloxacin) inhibited PHO proliferation and interfered with metabolic activity at all concentrations. Tetracycline and rifampin inhibited proliferation of PHO. However, both of these agents had a direct effect on the thiazolyl blue tetrazolium bromide (MTT) assay used to assess metabolic activity. Therefore, no conclusions could be drawn as to their effect on metabolic activity.
The observed cytotoxic and cytostatic effects of clindamycin, macrolides, fluoroquinolones, linezolid, chloramphenicol, rifampin, and tetracycline on PHO can be explained by an impairment of mitochondrial energetics. It is known that macrolides and clindamycin accumulate intracellularly. These agents at high localized concentrations could be cytotoxic or cytostatic for bone cells in vivo after local administration. Some of these agents might impair bone metabolism, even in accepted therapeutic serum concentrations. The inhibitors of bacterial cell wall synthesis (with the exception of cefazolin with an unknown eukaryotic target) and aminoglycosides had no effect on PHO cytotoxicity because they are unable to enter the cells in the absence of a specific receptor. Interestingly, lincomycin had no effect on cytotoxicity, proliferation, or metabolic activity, even at higher concentrations.
Aminoglycosides (gentamicin) are the most frequently used antibiotics for local treatment of bone infections and are known to affect mitochondrial protein synthesis. Despite this effect on protein synthesis, Duewelhenke et al. did not find any effect on PHO. This finding is in contrast to Isefuku et al., Pedersen et al., and Yuhan et al. who found that gentamicin at higher concentrations inhibits proliferation and differentiation of hMSCs, which could compromise the bone healing process. Gentamicin applied topically to localized bone infections has been reported to be effective and to circumvent the problems associated with systemic administration. However, gentamicin at 100 μg/mL (0.1 μg/μL) and higher was shown to have a substantial inhibitory effect on hMSCs in vitro and in vivo . Because hMSCs are instrumental for bone formation following bone grafting procedures, the use of gentamicin in concentrations higher than 100 μg/mL may compromise or delay new bone formation.
Kim et al. evaluated the effect of high local concentrations of gentamicin or tetracycline versus normal saline on bone repair, induced by demineralized bone in rats. Gentamicin was used at a concentration of 15 mg/0.5 mL (30 μg/μL) of demineralized freeze-dried bone (DFDB), and tetracycline was used at 30 mg/0.5 mL (60 μg/μL) of DFDB. In this study, antibiotic-treated groups demonstrated cellular necrosis or growth inhibition as opposed to the saline-treated group. This result suggests that saline mixed with bone grafts is an effective material for the regeneration of osseous defects; however, if an infection is present, then the use of gentamicin or tetracycline would be of value. Locally applied gentamicin is used in orthopedics for the treatment of osteomyelitis. It has also been used as gentamicin-containing cement for implantation and is one of the most recognized localized antibiotic treatments for skeletal infections. However, prophylactic addition of gentamicin and tetracycline to bone graft mixtures in an effort to facilitate the (re)generation of bone is questionable.
Lincomycin did not inhibit proliferation or affect metabolic activity at any concentration, and it displays good activity against anaerobes. Lincomycin, when used systemically, is known for causing pseudomembranous colitis and, as a result, has fallen into disuse. Local application at the time of graft placement should circumvent this systemic toxic effect. This is an area of implant therapy that warrants further investigation.
Penicillin and amoxicillin did not affect PHO proliferation or metabolic activity, even at high concentrations, but these two agents have minimal activity against anaerobes. Kuriyama et al. tested 800 bacterial isolates and found that 34% produced β-lactamase, therefore making penicillin or amoxicillin an inferior choice as a prophylactic antibiotic when mixed with bone grafting material. Cefazolin does have greater activity against anaerobes found in the mouth and is more resistant to hydrolysis by β-lactamases.
For cefazolin the mean IC that inhibited proliferation 20% (IC 20 ) is 380 μg/mL. The minimum bacterial inhibitory concentration (MIC 90 ) for 155 isolates for cefazolin is 64 μg/mL. To prepare a solution that is cefazolin 250 μg/mL, dilute 500 mg of cefazolin with 9.5 mL of 0.9% normal saline (final volume 10 mL), then take 250 μL (25 units) of this initial dilution and add to 50 mL of 0.9% normal saline. This final concentration would be approximately 4 times the MIC 90 for the various isolates. The 25 units can be measured by using an insulin U-100 syringe of which 100 units represent 1 mL, therefore 1 unit equals 10 μL. A reasonable final solution volume to add to each gram of grafting material would be 0.3 mL (300 μL) of diluted antibiotic.
For clindamycin the mean IC that inhibited proliferation 20% (IC 20 ) is 30 μg/mL. The minimum dental-related MIC 90 for more than 750 isolates is 0.03 to 0.25 μg/mL. To prepare a solution that is clindamycin 20 μg/mL, dilute 0.5 mL of a 150-mg/mL stock solution with 9.5 mL of 0.9% normal saline (1 : 20 dilution), then using an insulin U-100 syringe, take 133 μL (13.3 units) of this initial dilution and add to 50 mL of 0.9% normal saline. This final concentration would be approximately 140 times the MIC 90 for the various isolates. A reasonable final solution volume to add to each gram of grafting material would be 0.3 mL (300 μL) of diluted antibiotic. Clindamycin would be a preferred agent because of its activity against anaerobes, but it must be used at a subtoxic concentration ( Table 23-2 ).
Antibiotic | Dilution | Add to 50 mL of 0.9% Normal Saline * |
Cefazolin, 500 mg powder | Mix with 9.5 mL of 0.9% normal saline (final volume 10 mL) | 250 μL, 25 units |
Clindamycin, 150 mg/mL | Dilute 1 : 20 (e.g., 0.5 mL stock with 9.5 mL 0.9% normal saline) (final volume 10 mL) | 133 μL, 13.3 units |
* Use 0.3 cc of final diluted antibiotic for every 1 g of bone grafting material.
In summary, lincomycin may be the agent of choice because it does not interfere with proliferation or metabolic activity at any concentration, but there are no clinical studies to confirm in vivo efficacy. Tetracycline and gentamicin have extensive anecdotal clinical data, but are known to inhibit osteoblastic activity and therefore may not be suitable for the prophylactic addition to grafting material. Cefazolin does have documented efficacy and may be a suitable choice, but it does not have an extended anaerobic spectrum. Clindamycin does have a broader anaerobic spectrum of activity and documented efficacy with a large therapeutic index. Therefore, its use is evidence based and a rational choice.
It is obvious that more in vitro and in vivo studies are needed to offer complete guidance in this area. From what is known, it is obvious the final administered concentration of the commonly used anecdotal bone grafting antibiotics, including tetracycline or clindamycin, is very important.
▪
ANTIBIOTICS ADDED TO BONE GRAFTING MATERIALS
It has become common practice to empirically add antibiotics to autogenous, allograft, or xenograft bone grafting materials. Unfortunately, a variety of anecdotal antibiotic bone grafting cocktails has been advocated, with little scientific evidence for their use. The blood supply to a recent bone graft is compromised, and therefore systemic use of antibiotics for either prophylaxis or treatment of an infected bone graft site may be insufficient to provide adequate antibacterial concentration levels. There is great interest in both orthopedics and implant dentistry for the local administration of antibiotics. Locally administered antibiotics may achieve a twenty-fold higher concentration in a contained area versus intravenous administration. This local administration is seen to offer promise in both prevention and treatment of localized osseous infections. Sanders et al. in 1983 reported that bone grafts containing antibiotics had greater predictability than those not containing antibiotics. Mabry et al. reported that the combination of gentamicin and tetracycline mixed with freeze-dried bone allografts increased osseous regeneration. Other studies have shown that the addition of antibiotics to graft material had a depressive effect on bone formation. These conflicting reports may be due in part to potential toxic effects of various antibiotics and their localized concentrations. Studies have demonstrated that some antibiotics are toxic to osteoblast-like cells, especially at higher concentrations. During the initial healing process of the bone graft, osteoblasts are present along with human mesenchymal stem cells (hMSCs), which migrate into the bone graft, where they proliferate and differentiate. If these progenitor cells should fail to mobilize, proliferate, or differentiate, a failure in bone development may occur. Knowing that high concentrations of antibiotics have influence on bone-forming cells and that anaerobes would most likely be the bacteria of concern, the clinician should consider these factors when making a choice of which antibiotic to add to the bone graft. Cavalier use of an inappropriate locally administered antibiotic may lead to toxic effects and result in the failure of the bone graft.
Duewelhenke et al. investigated the effect of 20 antibiotics from different classes and antibacterial mechanisms in cell cultures of primary human osteoblasts (PHO). The mean inhibitory concentrations (ICs) (IC 20 , IC 50 ) for proliferation and metabolic activity were compared ( Table 23-1 ). Cytotoxicity could not be observed 24 hours after treatment of PHO with inhibitors of bacterial cell wall synthesis, aminoglycosides, tetracycline, rifampin, and lincomycin at any concentration. Aminoglycosides and antibiotics that inhibit bacterial cell wall synthesis, with the exception of higher concentrations of cefazolin, did not have an effect on proliferation or metabolic activity. Cefazolin decreased the proliferation of PHO at higher concentrations. Clindamycin and erythromycin demonstrated cytotoxicity at higher concentrations, but not at lower concentrations. At ultralow concentrations, clindamycin and erythromycin did not affect proliferation or metabolic activity, but did have an effect at moderate to high concentrations. Azithromycin and roxithromycin demonstrated cytotoxicity, inhibited proliferation, and decreased metabolic activity even at lower doses.
Antibiotic | Mean IC (Proliferation, Metabolic Activity; m g/mL) | |
---|---|---|
IC 20 | IC 50 | |
Cefazolin | 380, >400 | >400, >400 |
Lincomycin | No effect | No effect |
Clindamycin | 30, 340 | 150, >400 |
Erythromycin | 30, 210 | 180, >400 |
Azithromycin | 20, 80 | 25, 160 |
Roxithromycin | 20, 110 | 70, 210 |
Ciprofloxacin | 70, 260 | 170, >400 |
Moxifloxacin | 80, 190 | 160 >400 |
Tetracycline | 40, * | 180, * |
* Could not be determined as a result of inference with assay.
The fluoroquinolones (ciprofloxacin and moxifloxacin) inhibited PHO proliferation and interfered with metabolic activity at all concentrations. Tetracycline and rifampin inhibited proliferation of PHO. However, both of these agents had a direct effect on the thiazolyl blue tetrazolium bromide (MTT) assay used to assess metabolic activity. Therefore, no conclusions could be drawn as to their effect on metabolic activity.
The observed cytotoxic and cytostatic effects of clindamycin, macrolides, fluoroquinolones, linezolid, chloramphenicol, rifampin, and tetracycline on PHO can be explained by an impairment of mitochondrial energetics. It is known that macrolides and clindamycin accumulate intracellularly. These agents at high localized concentrations could be cytotoxic or cytostatic for bone cells in vivo after local administration. Some of these agents might impair bone metabolism, even in accepted therapeutic serum concentrations. The inhibitors of bacterial cell wall synthesis (with the exception of cefazolin with an unknown eukaryotic target) and aminoglycosides had no effect on PHO cytotoxicity because they are unable to enter the cells in the absence of a specific receptor. Interestingly, lincomycin had no effect on cytotoxicity, proliferation, or metabolic activity, even at higher concentrations.
Aminoglycosides (gentamicin) are the most frequently used antibiotics for local treatment of bone infections and are known to affect mitochondrial protein synthesis. Despite this effect on protein synthesis, Duewelhenke et al. did not find any effect on PHO. This finding is in contrast to Isefuku et al., Pedersen et al., and Yuhan et al. who found that gentamicin at higher concentrations inhibits proliferation and differentiation of hMSCs, which could compromise the bone healing process. Gentamicin applied topically to localized bone infections has been reported to be effective and to circumvent the problems associated with systemic administration. However, gentamicin at 100 μg/mL (0.1 μg/μL) and higher was shown to have a substantial inhibitory effect on hMSCs in vitro and in vivo . Because hMSCs are instrumental for bone formation following bone grafting procedures, the use of gentamicin in concentrations higher than 100 μg/mL may compromise or delay new bone formation.
Kim et al. evaluated the effect of high local concentrations of gentamicin or tetracycline versus normal saline on bone repair, induced by demineralized bone in rats. Gentamicin was used at a concentration of 15 mg/0.5 mL (30 μg/μL) of demineralized freeze-dried bone (DFDB), and tetracycline was used at 30 mg/0.5 mL (60 μg/μL) of DFDB. In this study, antibiotic-treated groups demonstrated cellular necrosis or growth inhibition as opposed to the saline-treated group. This result suggests that saline mixed with bone grafts is an effective material for the regeneration of osseous defects; however, if an infection is present, then the use of gentamicin or tetracycline would be of value. Locally applied gentamicin is used in orthopedics for the treatment of osteomyelitis. It has also been used as gentamicin-containing cement for implantation and is one of the most recognized localized antibiotic treatments for skeletal infections. However, prophylactic addition of gentamicin and tetracycline to bone graft mixtures in an effort to facilitate the (re)generation of bone is questionable.
Lincomycin did not inhibit proliferation or affect metabolic activity at any concentration, and it displays good activity against anaerobes. Lincomycin, when used systemically, is known for causing pseudomembranous colitis and, as a result, has fallen into disuse. Local application at the time of graft placement should circumvent this systemic toxic effect. This is an area of implant therapy that warrants further investigation.
Penicillin and amoxicillin did not affect PHO proliferation or metabolic activity, even at high concentrations, but these two agents have minimal activity against anaerobes. Kuriyama et al. tested 800 bacterial isolates and found that 34% produced β-lactamase, therefore making penicillin or amoxicillin an inferior choice as a prophylactic antibiotic when mixed with bone grafting material. Cefazolin does have greater activity against anaerobes found in the mouth and is more resistant to hydrolysis by β-lactamases.
For cefazolin the mean IC that inhibited proliferation 20% (IC 20 ) is 380 μg/mL. The minimum bacterial inhibitory concentration (MIC 90 ) for 155 isolates for cefazolin is 64 μg/mL. To prepare a solution that is cefazolin 250 μg/mL, dilute 500 mg of cefazolin with 9.5 mL of 0.9% normal saline (final volume 10 mL), then take 250 μL (25 units) of this initial dilution and add to 50 mL of 0.9% normal saline. This final concentration would be approximately 4 times the MIC 90 for the various isolates. The 25 units can be measured by using an insulin U-100 syringe of which 100 units represent 1 mL, therefore 1 unit equals 10 μL. A reasonable final solution volume to add to each gram of grafting material would be 0.3 mL (300 μL) of diluted antibiotic.
For clindamycin the mean IC that inhibited proliferation 20% (IC 20 ) is 30 μg/mL. The minimum dental-related MIC 90 for more than 750 isolates is 0.03 to 0.25 μg/mL. To prepare a solution that is clindamycin 20 μg/mL, dilute 0.5 mL of a 150-mg/mL stock solution with 9.5 mL of 0.9% normal saline (1 : 20 dilution), then using an insulin U-100 syringe, take 133 μL (13.3 units) of this initial dilution and add to 50 mL of 0.9% normal saline. This final concentration would be approximately 140 times the MIC 90 for the various isolates. A reasonable final solution volume to add to each gram of grafting material would be 0.3 mL (300 μL) of diluted antibiotic. Clindamycin would be a preferred agent because of its activity against anaerobes, but it must be used at a subtoxic concentration ( Table 23-2 ).
Antibiotic | Dilution | Add to 50 mL of 0.9% Normal Saline * |
Cefazolin, 500 mg powder | Mix with 9.5 mL of 0.9% normal saline (final volume 10 mL) | 250 μL, 25 units |
Clindamycin, 150 mg/mL | Dilute 1 : 20 (e.g., 0.5 mL stock with 9.5 mL 0.9% normal saline) (final volume 10 mL) | 133 μL, 13.3 units |
* Use 0.3 cc of final diluted antibiotic for every 1 g of bone grafting material.
In summary, lincomycin may be the agent of choice because it does not interfere with proliferation or metabolic activity at any concentration, but there are no clinical studies to confirm in vivo efficacy. Tetracycline and gentamicin have extensive anecdotal clinical data, but are known to inhibit osteoblastic activity and therefore may not be suitable for the prophylactic addition to grafting material. Cefazolin does have documented efficacy and may be a suitable choice, but it does not have an extended anaerobic spectrum. Clindamycin does have a broader anaerobic spectrum of activity and documented efficacy with a large therapeutic index. Therefore, its use is evidence based and a rational choice.
It is obvious that more in vitro and in vivo studies are needed to offer complete guidance in this area. From what is known, it is obvious the final administered concentration of the commonly used anecdotal bone grafting antibiotics, including tetracycline or clindamycin, is very important.
▪
PERIIMPLANTITIS
Implant success is not defined simply by osseous integration or a technically correct prosthesis, but rather by long-term function. Implant dentistry has a remarkable long-term success rate for both complete and partially edentulous patients. Schwartz-Arad et al. in 2005 reported that the 10-year cumulative survival rate for maxillary and mandibular dental implants when restored by overdentures was 95.4% (maxilla, 83.5%, mandible, 99.5%). Another study reported a 12-year cumulative implant survival rate for nonsmokers of 97.7%. These long-term success rates support the concept that implant dentistry is a predictable modality and thus an excellent option for practitioners and their patients. Most failures occur during the initial healing phase or during the first year after prosthetic loading. Occasional complications do arise during maintenance and retention of implants. The hard and soft tissues supporting dental implants are susceptible to microbial disease that may lead to the loss of what had been a previously successful functioning implant. Chronic inflammation and infection of the tissues surrounding the dental implant can lead to the loss of supportive alveolar bone. This condition is known as periimplantitis (PI). PI has a confirmed infectious cause, with high levels of periodontal pathogens, including Actinobacillus actinomycetemcomitans (AA), Porphyromonas gingivalis, Porphyromonas intermedia, Tannerella forsythia , and Treponema denticola , reported. Even with the confirmed infectious cause, it must be recognized that PI is a multifaceted disease process, which may also include host immune response and susceptibility, host modifying factors, and local environment. The contribution of factors, such as implant surface type, occlusal loading scheme, smoking, and a history of chronic periodontitis, is still unknown.
PI is mainly a localized lesion, and therefore local treatment seems logical. The known bacterial cause supports the concept that PI is a site-specific inflammatory response to bacteria, rather than simply a patient-associated specific host response. Limited studies that have examined the outcome of PI treatment suggest that systemic antibiotics in combination with mechanical débridement are partially successful in controlling PI. There is a lack of studies reporting 1-year microbial profiles when systemic antibiotics are used as the primary form of treatment.
PI is not synonymous with “failing implant” or “ailing implant,” as defined by the first European workshop on periodontology in 1993. Dental implant loss as a result of PI is not inevitable, and the severity of it may be kept to a minimum if the lesion is detected at an early stage and the disease is intercepted by appropriate means. Logical and evidence-based criteria should be used in the development of an appropriate strategy.
The cumulative interceptive supportive therapy (CIST) protocol, developed at the University of Bern School of Dental Medicine (2000), has demonstrated that periimplant infections can be successfully controlled by a protocol that is cumulative in nature and includes four sequential steps. These steps begin with mechanical débridement and proceed sequentially through antiseptic therapy, antibiotic therapy, and regenerative or resective surgery. This section will focus on the various pharmacologic agents used in PI treatment. Table 23-3 provides a treatment protocol for varying degrees of PI.
Clinical Parameters | Maintenance Classification | Treatment | ||||
---|---|---|---|---|---|---|
Plaque | Bleeding on Probing | Suppuration | Periimplant Probing Depth (mm) | Radiographic Bone Loss | ||
± | − | − | <4 | − | 0 | (A) |
+ | + | − | <4 | − | 1 | A |
+ | + | ± | 4-5 | + | 2 | A + B |
+ | + | ± | >5 | ++ | 3 | A + B + C |
+ | + | ± | >5 | +++ | 4 | A + B + C + D |
Mechanical débridement (treatment A) with antiseptic treatment (treatment B) is indicated in situations where there is plaque present, bleeding on gentle probing, periimplant probing depth 4 to 5 mm, possible incipient radiographic bone loss, and suppuration. Antiseptic treatment includes the application of a potent agent, such as chlorhexidine digluconate, either in the form of a daily rinse (0.1%, 0.125%, or 0.2%), or as a gel applied to the PI site. Three to four weeks of regular application are necessary to achieve a positive result. Chlorhexidine is available with or without alcohol and with 0.055% sodium fluoride. All preparations have been shown to exhibit equal clinical efficacy with respect to gingival index, plaque index, discoloration index, and bleeding on probing. There are several oral antiseptic mouth rinses available, but unfortunately most have not been subject to controlled studies evaluating their efficacy in the treatment of PI; therefore, we are unable to address their use.
Localized antiseptic (treatment B) and systemic antibiotic therapy (treatment C) are indicated when periodontal probing depth values of the periimplant sulcus or pocket increase to greater than 5 mm or more, plaque deposits are present, bleeding occurs on probing, and evidence of bone loss on radiographs is present. Suppuration may or may not be present. This periimplant lesion and pocket represents an ecologic niche, which is conducive to colonization with Gramnegative anaerobic, periodontopathic bacteria. The antibacterial treatment must include antibiotics to eliminate or at least appreciably reduce the pathogens in the submucosal ecosystem. Mombelli and Lang in 1992 demonstrated that if the previous treatment is used, soft tissue healing can be achieved. Before the administration of antibiotics, both mechanical débridement and antiseptic treatments must be performed.
Systemic antibiotic therapy (treatment C) should be directed toward the elimination of Gram-negative anaerobic bacteria. Metronidazole (Flagyl) would be the preferred antibiotic. Systemic antibiotics are unlikely to penetrate the established biofilm on the dental implant, and therefore thorough local débridement is necessary. The clinical experimental study by Mombelli and Lang validated the use of débridement and oral antibiotics. They found that periimplant infections treated with this protocol were resolved and remained stable for a documented period of 1 year. Prophylactic procedures should be instituted to prevent reinfection. The protocol suggested by Mombelli and Lang is that metronidazole when used as a single agent should be prescribed as 250 mg t.i.d. for 10 days. They also suggest the use of metronidazole 250 mg b.i.d. plus amoxicillin 375 mg/day for 10 days. Metronidazole has also been used in combination with amoxicillin and clavulanic acid (Augmentin) or ciprofloxacin in the treatment of periodontitis involving AA and may therefore be of value in treating PI. It is important that systemic antibiotic therapy should be continued for 10 days. For those cases that are refractory in nature, it would be logical and prudent to prescribe metronidazole 250 to 500 mg t.i.d. in conjunction with amoxicillin 500 mg t.i.d.
Matrix metalloproteinases (MMPs) form a family of enzymes that mediate multiple functions involved in tissue destruction and immune responses related to periodontal inflammation. The expression and activity of MMPs in healthy periodontium surrounding teeth is low, but is drastically enhanced to pathologically elevated levels in the presence of dental plaque and infection-induced periodontal inflammation. Hard and soft tissue destruction during PI is thought to be the result of a cascade involving bacterial virulence factors and/or enzymes, proinflammatory cytokines, reactive oxygen species, and MMPs. Ironically, recent studies suggest that MMPs can also exert antiinflammatory effects in defense of the host by processing antiinflammatory cytokines and chemokines and by regulating apoptotic and immune responses. In spite of this antiinflammatory host defense mechanism for MMPs, they do induce the fragmentation of an epithelial-cell derived adhesion protein (laminin-5), which can induce the apical migration of epithelial cells during the development of periodontal pockets. Low-dose doxycycline (LDD) (Periostat), administered orally, is an MMP-inhibitor drug that reduces laminin-5 levels. Thus, LDD therapy may also be used as an adjunct to mechanical débridement in the treatment of periodontal lesions. Clinical studies are needed to further explore the possible efficacy of this treatment form.
The application of local antibiotics through the use of controlled delivery devices has emerged recently as a suitable treatment concept. It is important that only release devices that ensure adequate release-kinetics be used to ensure successful clinical results. The antibiotic must remain at the site of action for at least 7 to 10 days in a concentration high enough to penetrate the submucosal biofilm. Tetracycline periodontal fibers (Actisite), minocycline hydrochloride microspheres 1 mg (Arestin), and sustained-release doxycycline (Atridox) are three commercially available delivery systems.
Mombelli et al. found that tetracycline periodontal fibers significantly lowered the anaerobic colony-forming units (CFUs) from baseline at months 1, 3, and 6 ( P < 0.001). There was also a significant decrease in mean periimplant probing depth from 6.0 to 4.1 mm ( P < 0.001), which was sustained for more than 12 months. The bleeding tendency was significantly reduced after 1 month and thereafter ( P < 0.001).
Minocycline microspheres 1 mg have also been used with favorable results in treating PI, following mechanical débridement and chlorhexidine treatment. Minocycline is a tetracycline derivative that exhibits both antimicrobial activity on periodontal pathogens and inhibitory properties on MMPs associated with tissue destruction. Oringer et al. found that these microspheres, placed subgingivally, induced reductions in AA, P. gingivalis, T. forsythia , and T. denticola up to 180 days after treatment. This study contained only 25 subjects and therefore may not be statistically significant. Minocycline microspheres have demonstrated an effective short-term reduction in the gingival cervical fluid biomarker interleukin 1-beta (IL-1β). IL-1β, when chronically present, is a bone-resorptive cytokine that is associated with periodontal disease activity.
A clinical trial for the treatment of PI revealed that the treatment group receiving mechanical débridement with application of a locally applied biodegradable sustained-release doxycycline experienced significant benefits over mechanical débridement alone. There was a mean reduction in pocket probing depth of 1.15 mm with an improvement in probing attachment levels of 1.17 mm. The doxycycline-treated group demonstrated a significant reduction in pocket probing depths and mean probing attachment levels (0.6 mm), accompanied by a significant reduction in mean bleeding on probing ( P = 0.001). Sustained-release doxycycline in conjunction with mechanical débridement appears to be beneficial in the treatment of PI.
It is not fully known if bacteria and their by-products need to be completely removed from an implant surface to obtain a predictable and successful result. There have been numerous attempts to gain a “decontaminated” implant surface. These efforts include use of citric acid, chlorhexidine gluconate, hydrogen peroxide, tetracycline HCl, polymyxin B, and stannous fluoride. At this time, no one particular agent or combination has proven to be convincingly superior.
Conclusion:
PI is a significant risk factor that is preventable and treatable. Although it can, it does not have to lead to late implant failure. Mechanical débridement and surface decontamination are certainly key components for treatment of PI, but the addition of the proper pharmacologic agent, either locally or systemically, will improve the prognosis and success rate.
▪
NSAIDS AND BONE DEVELOPMENT
The process of normal bone healing or formation can be divided into four phases: immediate, early, regenerative, and remodeling. The immediate phase consists of localized tissue hypoxia and hematoma formation. Hypoxia is thought to initiate a signaling cascade that begins the healing process. Following the initiation of this signaling cascade, the early phase begins and is characterized by an inflammation response with the recruitment of cells to the surgical site through chemoattraction and increased proliferation. The regenerative phase involves the development of new woven bone through intramembranous ossification and requires angiogenesis. Remodeling follows, with the maturation of the newly formed woven bone into mature lamellar bone that will have mechanical integrity.
Even though all phases of the bone formation process are important, the early phase is critical for the ultimate success of bone formation. During the early phase, there are increased levels or increased gene expression of proinflammatory cytokines, mitogenic growth factors, and cell-differentiating growth factors. Inflammation, which occurs at the onset of early phase injury-induced bone formation, is hypothesized to provide the initial signaling molecules for continuation of the healing cascade. Mitogenic growth factors and celldifferentiation growth factors act on osteoblast’s precursor cells, which are provided primarily by the periosteum. Existing bone marrow (when present), small blood vessels, and fibroblasts are secondary sources of precursor cells.
The enzyme cyclooxygenase-2 (COX-2) is important to the course of normal inflammation. The rate-limiting step in the synthesis of prostaglandins (PGs) from arachidonic acid is catalyzed by COX-2. PGs are bioactive lipids and are of significance because they possess proinflammatory effects plus other varied physiologic effects. COX-2 is responsible for PGE 2 and PGF 2 formation. PGE and PGF have been detected at increased levels during the early phase of healing in rabbit osteotomy callus and surrounding muscle. This finding suggests that both PGE and PGF have a functional role in healing.
NSAIDs are often used at the time of dental implant placement or bone grafting procedures for their analgesic and antiinflammatory efficacy. The inhibition of COXs is the mechanism by which NSAIDs achieve their intended effects. Traditional NSAIDs (tNSAIDs), which inhibit COX-1 and COX-2, are drugs such as aspirin, indomethacin, ibuprofen, ketoprofen, flurbiprofen, naproxen, and diclofenac. There are also drugs that specifically inhibit COX-2, such as celecoxib (Celebrex), valdecoxib (Bextra), and meloxicam (Mobic). Following the hypothesis that inflammation is necessary for normal bone healing and formation, postoperative NSAID therapy may potentially delay bone healing. This phenomenon has been demonstrated in several animal models. Also, there is clinical evidence that nonselective and COX-2-specific NSAIDs exert inhibitory effects on new bone formation. This evidence has been challenged by others who found that the use of tNSAIDs did not significantly influence fracture consolidation or ectopic bone formation induced by demineralized bone matrix in rodent models. Three different research groups found that in male rats the COX-2 inhibitor celecoxib is metabolized so quickly by the liver that even high doses given once daily will yield too low a serum concentration to be relevant.
Simon in 2007 demonstrated that higher doses and longer periods of celecoxib treatment in female rodents (8 mg/kg/day; normal human dose is 2.67 to 5.34 mg/kg/day) were more detrimental to fracture healing than lower doses (2 mg/kg/day) and shorter periods of celecoxib treatment. Female rodents that were treated with a modest dose of celecoxib (4 mg/kg/day) for 5 days following a fracture had significantly worse outcomes after 8 weeks of healing than control rats. Therefore, one may suggest that NSAID therapy following a fracture could adversely affect healing in humans. Two recent human studies do offer support for this conclusion. The Giannoudis study may have the greatest correlation to dental implant surgery. They found a marked association between nonunion of femoral diaphysis fractures that were stabilized with implant fixation and the use of NSAIDs after injury (p = 0.000001). The test population that received NSAID therapy (mean therapy time 21.2 weeks) did not develop union of the fracture. Those that received NSAIDs for only 1 week did attain fracture union, but at a slower rate (7.5 months) than those that did not receive any NSAIDs (5.5 months). All NSAID therapy patients (both long term and short term) had an odds ratio for nonunion of 10.74 (95% confidence interval 3.55 to 33.23; p = 0.000001). The dosages of the two main NSAIDs used, ibuprofen and diclofenac, were not reported. Their conclusion was that, even though there are many factors involved in bone healing, the main association of nonunion or delayed union of implant-stabilized fractures with the use of NSAIDs was noteworthy, and therefore they recommend excluding these drugs from clinical use in patients with diaphyseal fracture. The dental literature offered an animal study that examined the effect of COX-2− / − (lacking the COX-2 gene in both alleles) knockout mice on osseointegration. The results suggested that COX-2 played an essential role in osseointegration and that COX-2-selective NSAIDs may interfere with osseointegration clinically.
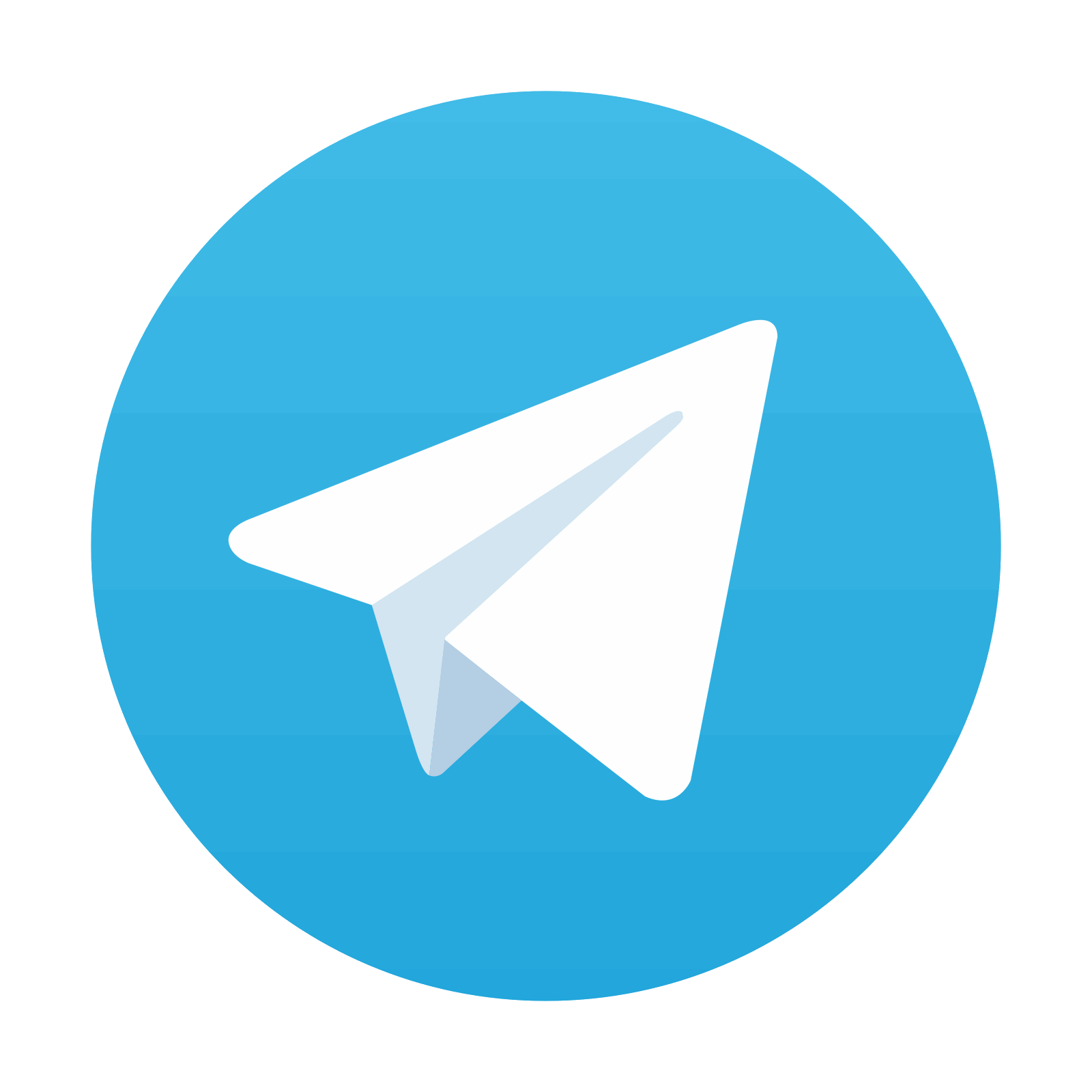
Stay updated, free dental videos. Join our Telegram channel

VIDEdental - Online dental courses
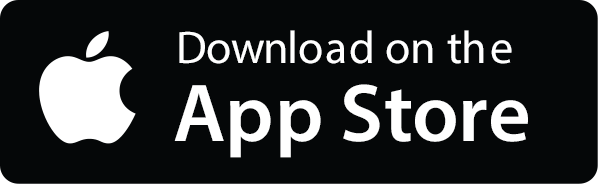
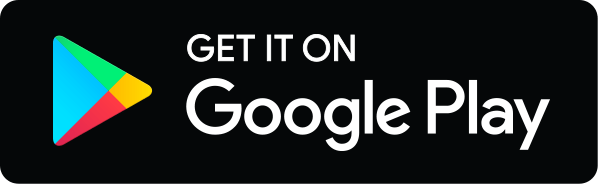