5 Orthodontic tooth movement
Biological basis of tooth movement
Physiology of bone
Bone remodelling
Regulation of bony deposition and resorption is important for normal maintenance of the skeleton and when these mechanisms break down, pathological change can occur. Numerous systemic and local factors have been implicated in bone remodelling (Table 5.1).
Table 5.1 Factors affecting bone remodelling and maintenance of the periodontal space
Systemic factors | Local factors |
---|---|
Parathyroid hormone | Cytokines and growth factors |
Vitamin D metabolites | Prostaglandins |
Calcitonin | Leukotrienes |
Biomechanics of tooth movement
Early research into tooth movement investigated the histological response of tissues using animal models, whilst more recent work has focused on cellular activity following mechanical stimulation (Box 5.1).
Pressure–tension theory
Histological studies carried out independently by Carl Sandstedt and Albin Oppenheim at the turn of the past century provided the foundation for current understanding of orthodontic tooth movement. When a force is placed on a tooth, bone is laid down on the tension side of the periodontal ligament and resorbed on the pressure side (Fig. 5.1). On the pressure side, when the force is light, multinucleate cells resorb bone directly. However, if the forces are higher and exceed capillary blood pressure, cell death can occur and a cell-free area forms. This is described as hyalinization, due to the glass-like appearance of these regions when viewed with light microscopy resembling hyaline cartilage. Resorption of these areas proceeds at a much-reduced rate. This process is described as undermining resorption and will result in slower tooth movement and greater pain and discomfort for the patient. Later work showed that even forces as light as 30g will produce some areas of hyalinization, and this tends to occur more with tipping than bodily movement of teeth, presumably because the force is dissipated more evenly through the periodontal ligament during bodily movement (Reitan, 1964).
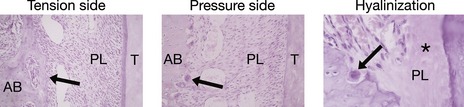
Figure 5.1 Histological appearance of the periodontium during orthodontic tooth movement.
Courtesy of Professor Jonathan Sandy.
From histological and clinical studies there appears to be a range of force effective for tooth movement (Storey & Smith, 1952) although the optimum force magnitude for orthodontic tooth movement has yet to be described (Ren et al, 2003). Light continuous forces are thought to be more effective than heavy forces as these will increase the risk of hyalinization, with no increase in the desired tooth movement but with greater potential anchorage loss (Fig. 5.2). However, large variation does exist between individuals and more important than the absolute force is the stress generated in the periodontal ligament. Stress is force per unit area and depending on the type of tooth movement, stress distribution within the periodontium will vary. Therefore, different force levels are recommended for different types of tooth movement (Table 5.2). Tipping teeth requires less force than bodily movement, whilst intrusive forces need to be light as these are dissipated through the apices of the teeth, increasing the risk of root resorption.
Tipping | 30–60 g |
Bodily movements | 100–150 g |
Rotational movements | 50–75 g |
Extrusion | 50–75 g |
Intrusion | 15–25 g |
Bone deflection and piezoelectricity
Orthodontic forces are transmitted within the periodontal ligament of the tooth and traditionally this has been thought to be via principle fibres of the periodontal ligament itself. However, when cross-linking of collagen is disrupted, the histological response of bone to orthodontic tooth movement appears normal (Heller & Nanda, 1979). Therefore, it has been proposed that the periodontal ligament represents a continuous hydrostatic system that distributes forces equally to all its regions (Baumrind, 1969). However, this would mean differential forces could not be distributed within the ligament, which is clearly not the case.
Teeth will displace a greater amount than the width of the periodontal ligament when force is initially applied to them, which implies that some deflection of alveolar bone also occurs. This might explain why differential forces can develop at the bone surfaces of the periodontal ligament. Bone deflection also produces stress-generated electrical potentials at the bone surface, which at one time were thought to be involved in bone remodelling. However, these are short-lived and very small and as such, unlikely to play an active role (McDonald, 1993).
Cellular shape change and signal transduction
A relationship appears to exist between cell shape and metabolic activity. Cell shape is controlled by the cytoskeleton, which terminates in specialized sites at the cell membrane, which form junctional complexes with the extracellular matrix. At these focal adhesion integrins, a family of proteins that span the cell membrane link the cytoskeleton to the extracellular matrix. They act as intracellular signalling receptors and are involved in numerous signalling pathways. Mechanical stress on cells within the periodontal ligament initiates both the cyclic AMP and phosphoinositide pathways, which results in an increase in production of secondary messengers. This produces an increase in intracellular calcium levels, which mediates further cellular events, including a stimulation of DNA synthesis (Harell et al, 1977).
Production of arachidonic acid metabolites
Arachidonic acid is an unsaturated fatty acid produced from membrane phospholipids, which is metabolized into prostaglandins, leukotrienes and thromboxanes (Fig. 5.3). These molecules are potent mediators of inflammation and numerous in vitro and in vivo experiments have shown a relationship between mechanical stimulation and prostaglandin production in bone. Based on this work, prostaglandins have been used clinically via local administration in the gingivae to increase the efficiency of orthodontic tooth movement (Yamasaki et al, 1984). The other principle products of arachidonic acid metabolism, leukotrienes, have also been shown to increase around teeth moved orthodontically. This may explain the observation that on administration of a non-steroidal anti-inflammatory drug in an animal model, there is a decrease in osteoclast numbers, but not tooth movement (Sandy & Harris, 1984). This suggests some overlap between the pathways and a degree of redundancy within the system. Inhibition of leukotriene production itself results in inhibition of tooth movement (Mohammed et al, 1989).
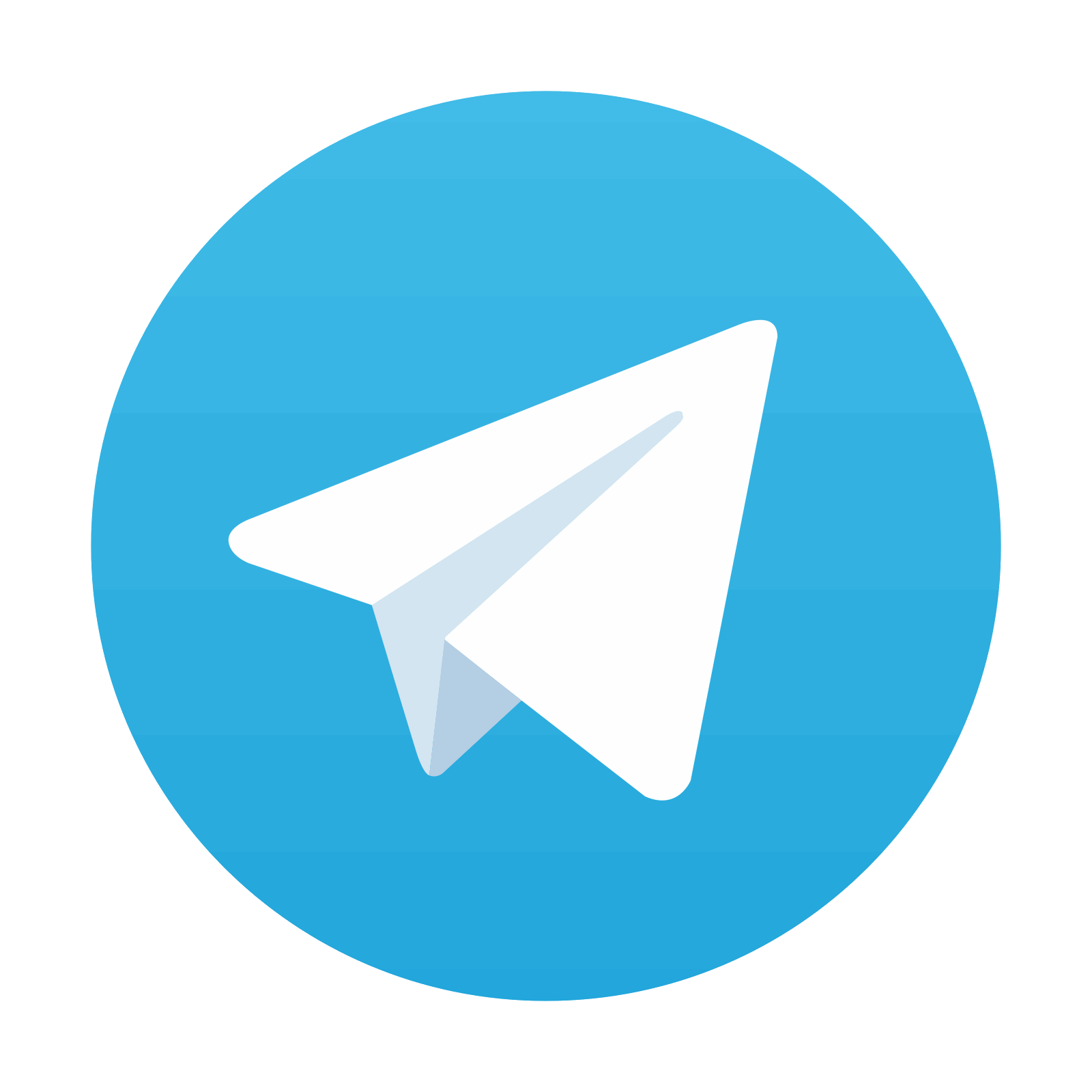
Stay updated, free dental videos. Join our Telegram channel

VIDEdental - Online dental courses
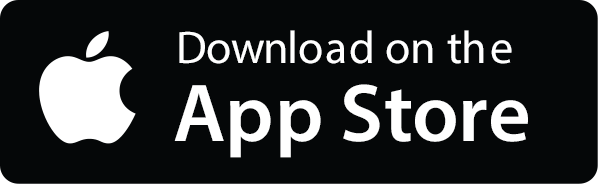
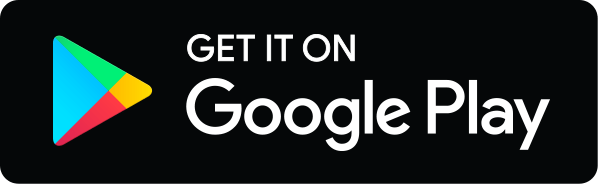