Abstract
Objective
A great benefit of FRC technology is that, in case of minor failure events, restorations can be repaired or reinserted. However, various FRC materials are available, that differ in matrix composition and fiber pre-treatment. The aim of this investigation was, therefore, to evaluate original and repair bond strength of FRC materials.
Methods
Five fully pre-impregnated, unidirectional FRCs were selected, one semi-interpenetrating polymer network FRC and four cross-linked-polymer FRCs. The primary endpoint was the evaluation of shear bond strength (SBS) between FRC and composite resin, which was performed by a universal testing machine. For each FRC specimens were divided into control (original SBS, resin to fresh FRC with oxygen inhibition layer (OIL), n = 30) and test groups (repair SBS, resin to FRC after removal of OIL and adhesive infiltration, n = 30).
Results
The cross-linked-polymer FRC GrandTec ® (12.4 ± 5.4 MPa) yielded the highest control SBS, followed by the semi-interpenetrating polymer network FRC (everStick ® , 9.2 ± 3.5 MPa). With everStick ® , repair led to a significant increase in the test SBS (14.6 ± 5.8 MPa, p = 0.01).
Significance
Control SBS was best with GrandTec ® indicating that the material is superior in direct clinical application. Test SBS was significantly increased with everStick ® which points at potential reparability and advantages in semi-direct or indirect fabrication of fiber-reinforced fixed partial dentures.
1
Introduction
The introduction of fiber-reinforced composites (FRCs) in dentistry enabled the development of tooth substance saving, esthetic and cost-effective treatment approaches . Today FRCs are applied in prosthodontic treatments with fixed partial dentures , removable dentures , periodontal splints , root canal posts with core buildups and orthodontic treatments .
However, being a complex structure consisting of inorganic and organic compounds, FRC restorations are prone to aging processes induced by exposure to the oral environment. It was shown that incomplete wrapping and coverage of fibers with resin matrix entails microvoids, leading to increased water sorption, hydrolysis and degradation of the polysiloxane network . Mechanical properties of FRC restorations can deteriorate over time and unfavorable events, such as fracture of the veneering composite, discoloration, or complete debonding, might occur. One of the great benefits of FRC technology is the possibility to extend functional survival by repairing restorations in the oral cavity . However, composite repair on an aged FRC substrate generally offers only 20–70% of the cohesive strength of bulk materials . Thus, different mechanisms may contribute to the creation of an FRC-repair composite resin interface that can withstand mechanical load. There might be (i) direct chemical bonds between an aged FRC matrix and the repair composite; (ii) micro-mechanical interlocking of the repair resin into minimal voids and gaps between exposed fibers and the FRC matrix; (iii) interdiffusion of fresh monomers into a semi-Interpenetrating Polymer Network (semi-IPN) . Repair bond strength can additionally be improved by the application of silanes which establish a link between monomers of the adhesive resin and exposed fibers of aged FRC . The bonding mechanism of silanes is a synergistic reaction. The silanol group adheres to the inorganic substrates and the organofunctional group bonds to the methacrylate groups of the monomers in the adhesive resin .
Today, different types of FRC materials are available for clinical use. They differ mostly in their matrix composition, and less in the pre-treatment of fibers or in the types of fibers. To incorporate fibers into a resin matrix, the fibers need to be covered with silane. Most manufacturers use silane compounds to ensure the reliable incorporation of fibers ( Table 1 ) . One manufacturer applies a plasma enhanced chemical vapor deposition (PECV) coating to pre-treat fibers before embedding them into a cross-linked polymer (CLP) resin matrix . Resin matrices of CLP materials consist of dimethacrylate monomers, e.g. Bis-GMA or UDMA, whereas semi-IPN matrices are composed of Bis-GMA and PMMA, which polymerize into two nearly independent polymer networks ( Table 1 ) . Why is the use of two polymer networks of value? Linear polymers can be fully dissolved, whereas cross-linked polymers do not dissolve . A polymerized semi-IPN structure can be partly dissolved by fresh resin monomers. This might be of use in repair procedures on polymerized and aged FRC substrates . The benefit of a material with a semi-IPN matrix seems to be highest when restorations need reattachment or repair after polymerization and removal of the oxygen inhibition layer. In one of our previous investigations the interdiffusion depths of fresh monomers into different FRC materials were visualized by confocal laser scanning microscopy (CLSM). It was evident that fresh monomers were able to diffuse significantly deeper into the semi-IPN FRC than into CLP FRCs . These results lead to the question whether differences in interdiffusion depth would entail variations in shear bond strength of repaired FRC-composite resin interfaces.
Name | everStick ® PERIO | GrandTec ® | Tender fiber ® | Splint-It ® | Dentapreg ® PFU |
---|---|---|---|---|---|
Code | ES | GT | TF | SI | DP |
Manufacturer | StickTech Ltd., Turku, Finland | Voco, Cuxhaven, Germany | Micerium, Avegno, Italy | Pentron Clinical Technologies, Orange (CA), USA | ADM, Brno, Czech Republic |
Type of matrix | Semi-IPN | CLP | CLP | CLP | CLP |
Resin matrix | PMMA Bis-GMA | UDMA TEGDMA | Bis-GMA | Bis-GMA | Mixture of methacrylates |
Type of fibers | E-glass | R-glass | Glass fibers | Silicate glass fibers and polyethylene fibers | S2-glass |
Fiber volume fraction (vol%) | 60 wt% a | 35–40% | 54.8 wt% a | 38–40% | 35–45% |
Number of fibers | 2000 | 4000–4500 | 6400 | Not specified | 8300 |
Direction of fibers | Unidirectional | Unidirectional | Unidirectional | Unidirectional | Unidirectional |
Coating of fibers | Epoxy silane | Silane | Silane | Not specified | Plasma-enhanced chemical vapour deposition (PECVD) |
LOT | 20130322EP05 | 1114411 | 2011001424 | 166340, 4144566 | PFU_09_072011 |
a Exact fiber volume fraction was not calculated by the manufacturer.
In literature, there are several investigations and experimental studies evaluating the bond strength between FRC and light-curing composite resin . However, none of them provides data on the comparison of shear bond strength (SBS) of five different materials in a standardized setup focusing on original and repair bond strength.
The aim of this investigation was to evaluate the original SBS between fresh polymerized FRC and resin composite (control), and between polymerized FRC with removed oxygen inhibition layer (OIL) and resin composite (test), simulating a repair situation.
The null hypotheses were: (1) When comparing FRC materials against each other there would be no difference in control SBS and test SBS; and (2) when comparing control and test SBS of each FRC material there would be no difference.
2
Materials and methods
2.1
Specimen preparation
The FRC materials used are listed in Table 1 . Five fully pre-impregnated unidirectional FRCs were selected, one semi-IPN FRC (everStick PERIO ® , StickTech Turku, Finland) and four CLP FRCs (GrandTEC ® , VOCO, Cuxhaven, Germany; TenderFibre ® , Micerium Avegno, Italy; Splint-It ® , Pentron Clinical Technologies, Orange CA, USA; Dentapreg PFU ® , ADM, Brno, Czech Republic). Sixty samples were prepared with each material, thirty for the control and thirty for the test group. Fig. 1 shows the schematic illustration of sample preparation. In the control group resin composite was applied to fresh FRC strands immediately after light-polymerization to obtain the original shear bond strengths (SBS). This procedure simulates a direct intraoral fabrication process. For the assessment of the repair SBS the oxygen inhibition layer was gently removed after light-polymerization and fresh adhesive resin and resin composite were applied as described below.
Metallic preproduction models were fabricated and duplicate negative silicone impressions were obtained. Adhesive interface dimensions were standardized by using the silicone impressions to fabricate specimens. A nano-filled light-curing composite resin (Tetric Evo Ceram, Ivoclar Vivadent AG, Schaan, Liechtenstein) was used. In the test group a Bis-GMA-, HEMA- and TEGDMA-based filled adhesive resin (Optibond FL ® , KerrHawe, Bioggio, Switzerland) was used for intermediate resin coating (IMR) and interdiffusion of fresh monomers into the FRC materials according to a published protocol .
In the test group the fabrication procedure was the following: 10 mm of each FRC material was cut off. To obtain a well-defined and standardized bonding interface (3 mm × 3 mm) the strands were pressed flat between two microscope slides and light-polymerized (SmartLite PS, DENTSPLY DeTrey GmbH, Konstanz, Germany; wavelength 450–490 nm, 890 (SD 10) mW/cm 2 ) according to the manufacturer’s instructions. The oxygen inhibition layer (OIL) was removed using gentle and intermittent sandblasting (Al 2 O 3 powder, KaVo, Rondoflex 2013, Kaltenbach & Voigt GmbH & Co., Biberach, Germany; particle size 27 μm; for 5 s). To ensure complete removal of the OIL the surface was gently treated with an ethanol solution (50%) and a microbrush (for 20 s). Directly after removal of the OIL a Bis-GMA-, HEMA- and TEGDMA-based filled adhesive resin (Optibond FL ® Adhesive, KerrHawe) was applied to the surface of the pre-treated FRC materials, and specimens were stored for wetting with fresh monomers (5 min) under a light-protection shield (Viva Pad ® , Ivoclar Vivadent AG, Schaan, Liechtenstein) . Finally, the composite resin was bonded to the FRC strand using the silicon molds ( Fig. 1 ). Specimens of the control group were fabricated according to the procedure described above. In the control group the removal of the OIL was omitted. Flowable composite resin (Tetric Evo Flow, Ivoclar Vivadent AG) and composite resin (Tetric Evo Ceram, Ivoclar Vivadent AG) were applied consecutively on the freshly polymerized FRC strands and light-polymerized, respectively.
2.2
Evaluation of shear bond strength (SBS)
Specimens were embedded into auto-polymerizing acrylic resin (Paladur transparent ® , Kulzer, Weinheim, Germany) and fixed to the universal testing machine (Zwicki 1120, Zwick, Ulm, Germany). Evaluation of SBS was performed with a crosshead speed of 1 mm/min according to ISO/TS 11405 . The orientation of the fiber was orthogonal to the shearing force ( Fig. 1 ). Force was applied until the load dropped to 50% of the maximum force.
Failure analysis of specimens was done using magnification loupes (6-fold) and diagnostic optical light (Sirolux F, Sirona Dental Systems GmbH, Bensheim, Germany). The categorization of failures was determined to be adhesive (failure occurs at the FRC-resin composite interface), cohesive (failure occurs within the FRC strands) or mixed (failure occurs partly at the interfacial area and within the FRC strand).
2.3
Sample size and statistical analysis
For each of the five materials 60 specimens were fabricated ( n = 30 control, n = 30 test). Mean values and standard deviations were calculated. Student’s t -test was performed to compare control vs. test within the FRC material type. One-way Analysis of Variance (ANOVA) and post hoc testing (Scheffé procedure) was carried out to compare test and control between FRC materials . Failure analysis is presented descriptively. Statistical analysis was performed using SAS version 9.2 (SAS Institute Inc., Cary, NC, USA).
2
Materials and methods
2.1
Specimen preparation
The FRC materials used are listed in Table 1 . Five fully pre-impregnated unidirectional FRCs were selected, one semi-IPN FRC (everStick PERIO ® , StickTech Turku, Finland) and four CLP FRCs (GrandTEC ® , VOCO, Cuxhaven, Germany; TenderFibre ® , Micerium Avegno, Italy; Splint-It ® , Pentron Clinical Technologies, Orange CA, USA; Dentapreg PFU ® , ADM, Brno, Czech Republic). Sixty samples were prepared with each material, thirty for the control and thirty for the test group. Fig. 1 shows the schematic illustration of sample preparation. In the control group resin composite was applied to fresh FRC strands immediately after light-polymerization to obtain the original shear bond strengths (SBS). This procedure simulates a direct intraoral fabrication process. For the assessment of the repair SBS the oxygen inhibition layer was gently removed after light-polymerization and fresh adhesive resin and resin composite were applied as described below.
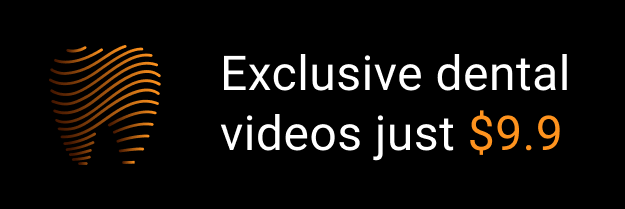