Optimal management of orbital fractures remains challenging and often enigmatic ( Fig. 39-1 ). Orbital anatomy is complex, and various vital structures and highly specialized organs are bundled in a small space. A number of approaches exist and numerous materials are available for reconstruction. No individual approach and no single material is best suited for all patients. Primary repair offers injured patients their best chance at functional recovery. Complications related to the injury itself or to repair, such as persistent enophthalmos or ocular dysmotility, are difficult to predict in the acute setting and, once clinically manifested, are challenging to repair because of intraconal or extraconal fibrosis.
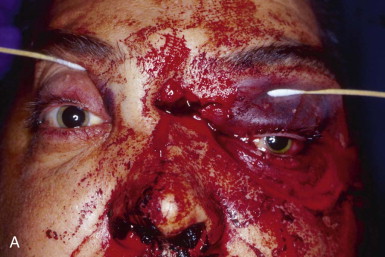
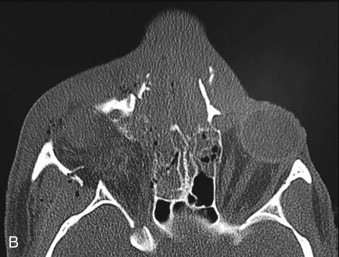
Several technologic advances have occurred in the last 3 decades that have had a significant impact on the management of patients with orbital injuries, including the following:
- •
Improved imaging modalities, such as high-resolution computed tomography (CT), which has resulted in a much greater ability to recognize, qualify, and quantify orbital injuries than was possible in the pre-CT scan era
- •
Refined alloplastic materials, which have largely replaced the once “gold standard” of calvarial bone grafts for use in the internal orbit
- •
Application of computer-aided design and computer-aided modeling (CAD/CAM) to maxillofacial surgery, which has provided a means to precisely analyze, segmentalize, and manipulate virtual images in advance of surgery to perform an ideal virtual reconstruction, as well as the construction of custom implants or guide stents
- •
The development of intraoperative navigation (frameless stereotaxy) and intraoperative CT scanners, which allow precise placement of implants or bone grafts, as well as confirmation of adequate reduction before leaving the operating room
- •
The application of minimally invasive, endoscopically assisted, transantral approaches to the orbits, which in selected cases obviates the need for a lower lid incision
This chapter is intended to provide readers with a rational approach to managing orbital injuries based on clinical and radiographic findings and to review the currently available adjuncts that can be used to optimize treatment outcomes.
Etiopathogenesis
Data from a level I trauma registry have shown that the orbit is involved in approximately 47% of severely injured patients admitted to a trauma service. The vast majority of these injuries occur as a result of blunt trauma, usually a motor vehicle collision or interpersonal violence, as well as sporting accidents, industrial accidents, and ground-level falls. Fractures involving the orbit may affect the internal orbit, the external orbital frame, or both ( Figs. 39-2 to 39-5 ). For purposes of clarity and discussion, these fractures will be defined and discussed as follows:
- •
Orbitozygomaticomaxillary complex (OZMC) fractures
- •
Naso-orbito-ethmoid (NOE) fractures
- •
Internal orbital fractures (blowout, blow-in)
- •
Combined orbital fractures (shattered orbit).
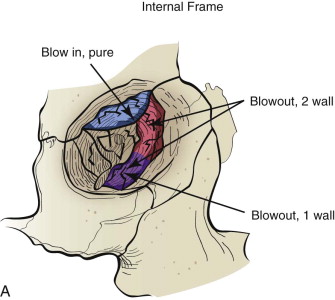
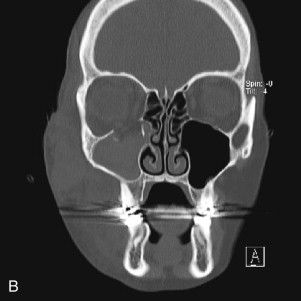
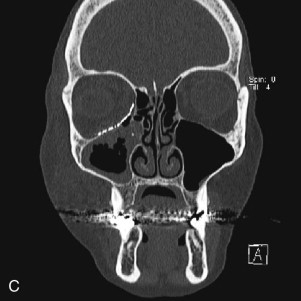
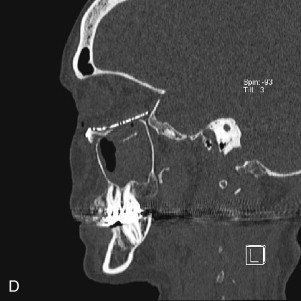
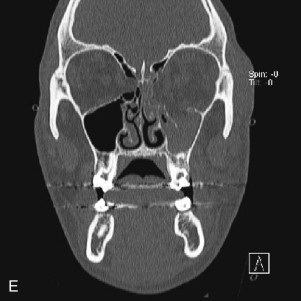
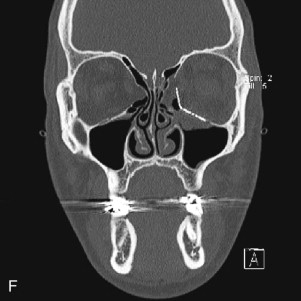
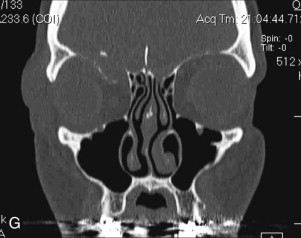
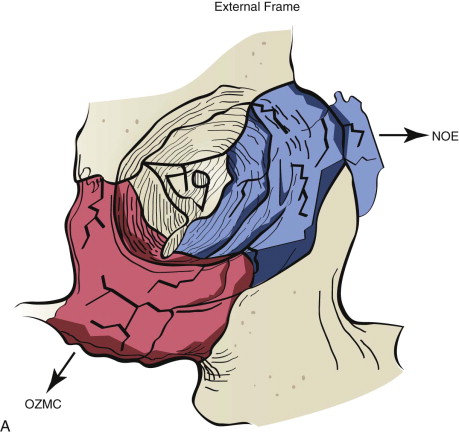
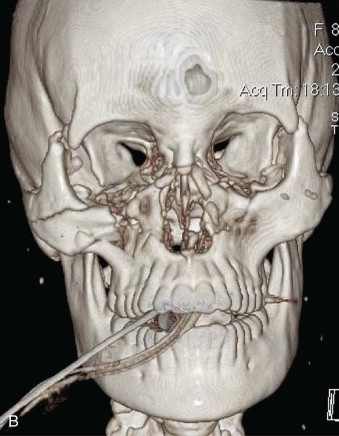
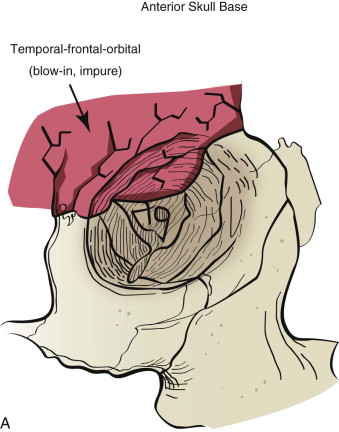
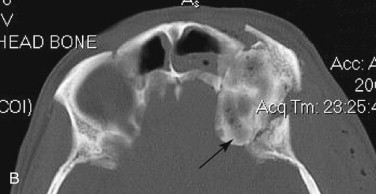
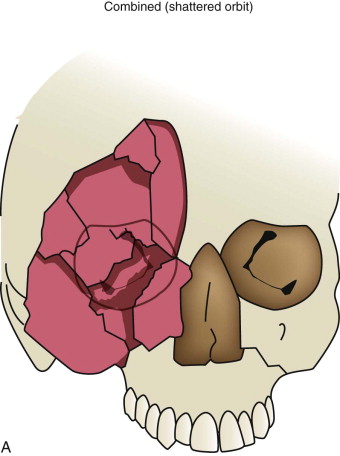
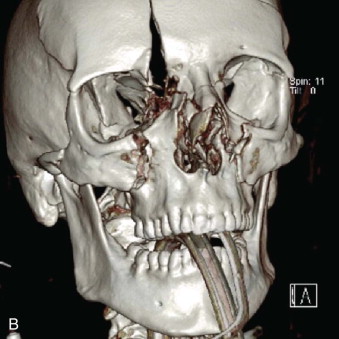
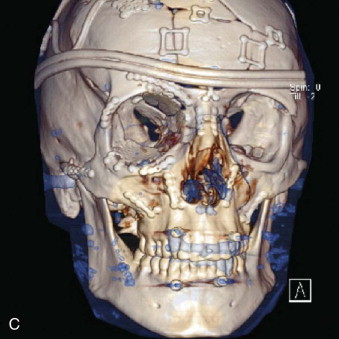
Fractures of the internal orbit typically occur by one of two mechanisms. The first is through a force applied to the globe itself, which results in a sudden increase in intraorbital pressure that exerts an outward force against the internal orbital walls, the weakest of which fractures. This “hydraulic theory,” popularized by Smith and Regan, provides an explanation why most orbital blowout fractures occur just medial to the infraorbital canal. In other instances, however, the force is applied directly to the bone, often the zygomatic bone or the infraorbital rim, or both, which produces an orbital floor fracture through direct transmission of energy from the orbital rim to the floor and results in a compression-type fracture. The orbital floor may fracture alone (termed pure blowout), or there may also be rim involvement (impure).
Once an internal orbital fracture occurs, the volume occupied by the soft tissue contents (the eye and adnexa) may expand or contract secondary to the direction of displacement of the orbital fracture (i.e., blow-in or blowout). Blow-in fractures typically occur on the orbital roof and are usually associated with high-velocity injuries involving the anterior skull base. Blow-in fractures result in contraction of orbital volume and downward and forward displacement of the globe. Most blowout fractures, in contrast, occur on the inferior or inferomedial aspect of the orbit and result in volumetric expansion with displacement of the globe posteromedially and inferiorly. Fracture displacement and orbital expansion or contraction may lead to extraocular muscle imbalance and subjective diplopia, enophthalmos, or proptosis.
Extraocular muscle imbalance and diplopia are generally the result of muscle contusion. Less commonly, however, they can be the result of incarceration of either the extraocular muscle (e.g., inferior rectus muscle) or the soft tissue adjacent to the muscles, cranial neuropathy (third, fourth, or fifth cranial nerves), or deviation of the visual axes. True entrapment in adult patients is very unusual. In children, however, blowout fractures may produce complete immobility of the ocular globe with enophthalmos ( Fig. 39-6 ). Such severe loss of motion implies actual muscle incarceration, which is an indication for immediate orbital exploration with release of the entrapped extraocular muscle system. It is typically accompanied by pain on attempted rotation of the globe, nausea, and vomiting, all of which are unusual in adult patients with blowout fractures.
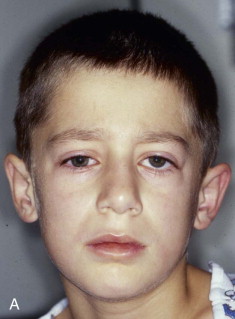
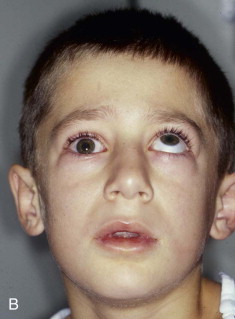
Enophthalmos is the second major potential complication of orbital injuries, the primary cause of which is an increase in orbital volume ( Fig. 39-7 ). Other mechanisms for enophthalmos are possible, including entrapment of extraocular muscles or periocular soft tissues, fat atrophy, or decrease in vitreous volume. For many years fat atrophy was thought to be a major etiologic factor in enophthalmos; however, studies by Manson and co-workers suggest that this is indeed not the case. It has been shown that much of the globe support and position within the orbit is due to intramuscular (intraconal) fat ( Fig. 39-8 ). Fat is located in a primarily extraconal position in the anterior orbit; however, posteriorly, where it is needed, most of the fat is intraconal. It is thought that this intraconal fat is extruded into an extraconal location and that when combined with post-traumatic scarring, clinically significant enophthalmos can result.
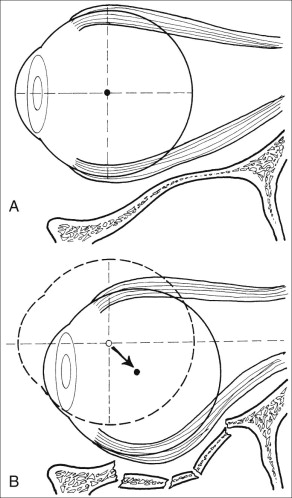
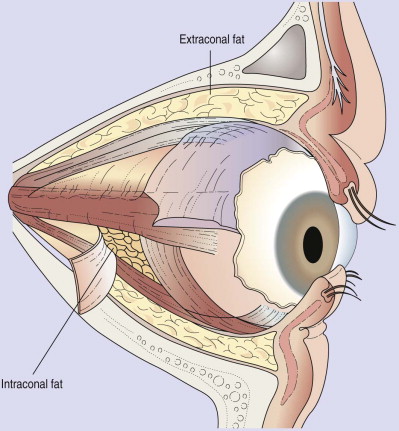
Pathologic Anatomy
In adult patients, orbital volume and external and internal morphology are remarkably consistent between individuals. The internal orbit is a cone-shaped pyramid with its apex oriented posteromedially and its base anterolaterally. The lateral orbital rim coincides with the mid-vertical axis of the globe. This relationship makes the lateral wall important in establishing correct anteroposterior position of the globe.
The internal orbit contains two critical bulges that are essential to restore when disrupted. The first “ethmoidal bulge,” also termed the “key area,” is located posteromedially within the orbit and results from medial extension of the ethmoidal air cells ( Fig. 39-9 ). The second critical area, the “antral bulge,” is located in the posteroinferior orbit and is the result of cranially oriented outpocketing of the maxillary antrum ( Fig. 39-10 ). These outpocketings serve to support the anterior position of the globe. Failure to restore these critical bulges will predictably result in increased orbital volume and risk for enophthalmos.
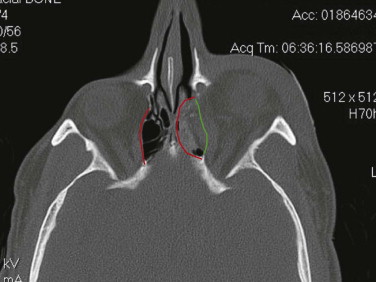
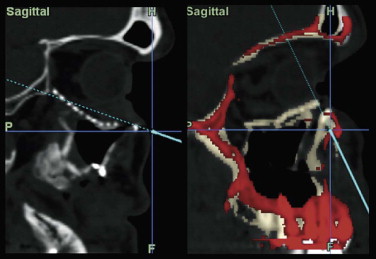
Soft tissue support of globe position within the orbit is provided by interrelated suspensory ligaments and a network of orbital fat ( Fig. 39-11 ). These ligaments include the medial and lateral canthal and check ligaments, the superior sling containing Whitnall’s ligament, the inferior sling containing Lockwood’s ligament, muscular sheaths and lacrimal ligaments, Tenon’s capsule, and the annulus of Zinn. Lockwood’s ligament is essentially a fascial thickening at the inferior aspect of the orbit that provides support to the globe, even in the absence of an intact orbital floor. The extrinsic muscles that penetrate this ligament are encased in “tubular reflections” of the same fascia that creates muscle compartments. Orbital fat is thus characterized by its position relative to the extraocular muscle compartments as being either extramuscular (extraconal) or intramuscular (intraconal). As mentioned previously, intraconal fat is thought to contribute to the vertical position and anterior projection of the globe. In a landmark anatomic study, Koornneef described a connective tissue framework in the midst of orbital fat consisting of septa that anchor the globe and extraocular muscles to the orbital wall. Thus, the globe receives its positional support from both structural soft tissue elements and the external and internal bony skeleton. Generally, a significant increase in orbital volume, caused by displacement or removal of one or more of the internal or external orbital walls, or a decrease in intraconal fat or globe volume will result in perceptible enophthalmos or ocular dysmotility.
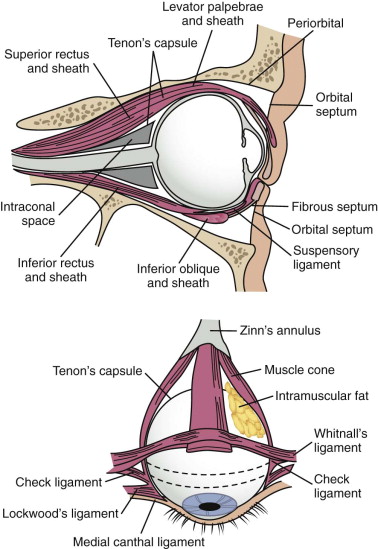
It is clear that increased orbital volume alone has the potential to cause enophthalmos or ocular dysmotility in some, but not all patients; enophthalmos may become apparent weeks or months after injury; diplopia or ocular dysmotility can take weeks to resolve; surgical repair is necessary in some, but not all patients; and if indicated, surgical repair is optimally performed within the first 2 weeks after injury because of secondary scarring intraorbitally or within Tenon’s capsule. Therefore, the questions that need to be answered by the clinician are (1) which patients with orbital fractures will benefit from surgical repair (i.e., is there a quantitative volumetric or linear threshold that predicts functional or esthetic complications?) and (2) which patients can be safely observed?
Pathologic Anatomy
In adult patients, orbital volume and external and internal morphology are remarkably consistent between individuals. The internal orbit is a cone-shaped pyramid with its apex oriented posteromedially and its base anterolaterally. The lateral orbital rim coincides with the mid-vertical axis of the globe. This relationship makes the lateral wall important in establishing correct anteroposterior position of the globe.
The internal orbit contains two critical bulges that are essential to restore when disrupted. The first “ethmoidal bulge,” also termed the “key area,” is located posteromedially within the orbit and results from medial extension of the ethmoidal air cells ( Fig. 39-9 ). The second critical area, the “antral bulge,” is located in the posteroinferior orbit and is the result of cranially oriented outpocketing of the maxillary antrum ( Fig. 39-10 ). These outpocketings serve to support the anterior position of the globe. Failure to restore these critical bulges will predictably result in increased orbital volume and risk for enophthalmos.
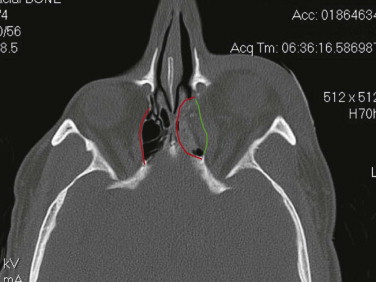
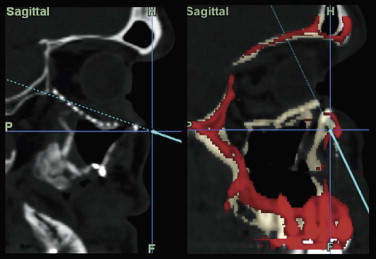
Soft tissue support of globe position within the orbit is provided by interrelated suspensory ligaments and a network of orbital fat ( Fig. 39-11 ). These ligaments include the medial and lateral canthal and check ligaments, the superior sling containing Whitnall’s ligament, the inferior sling containing Lockwood’s ligament, muscular sheaths and lacrimal ligaments, Tenon’s capsule, and the annulus of Zinn. Lockwood’s ligament is essentially a fascial thickening at the inferior aspect of the orbit that provides support to the globe, even in the absence of an intact orbital floor. The extrinsic muscles that penetrate this ligament are encased in “tubular reflections” of the same fascia that creates muscle compartments. Orbital fat is thus characterized by its position relative to the extraocular muscle compartments as being either extramuscular (extraconal) or intramuscular (intraconal). As mentioned previously, intraconal fat is thought to contribute to the vertical position and anterior projection of the globe. In a landmark anatomic study, Koornneef described a connective tissue framework in the midst of orbital fat consisting of septa that anchor the globe and extraocular muscles to the orbital wall. Thus, the globe receives its positional support from both structural soft tissue elements and the external and internal bony skeleton. Generally, a significant increase in orbital volume, caused by displacement or removal of one or more of the internal or external orbital walls, or a decrease in intraconal fat or globe volume will result in perceptible enophthalmos or ocular dysmotility.
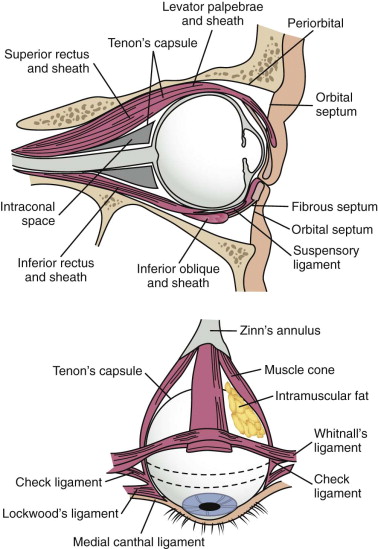
It is clear that increased orbital volume alone has the potential to cause enophthalmos or ocular dysmotility in some, but not all patients; enophthalmos may become apparent weeks or months after injury; diplopia or ocular dysmotility can take weeks to resolve; surgical repair is necessary in some, but not all patients; and if indicated, surgical repair is optimally performed within the first 2 weeks after injury because of secondary scarring intraorbitally or within Tenon’s capsule. Therefore, the questions that need to be answered by the clinician are (1) which patients with orbital fractures will benefit from surgical repair (i.e., is there a quantitative volumetric or linear threshold that predicts functional or esthetic complications?) and (2) which patients can be safely observed?
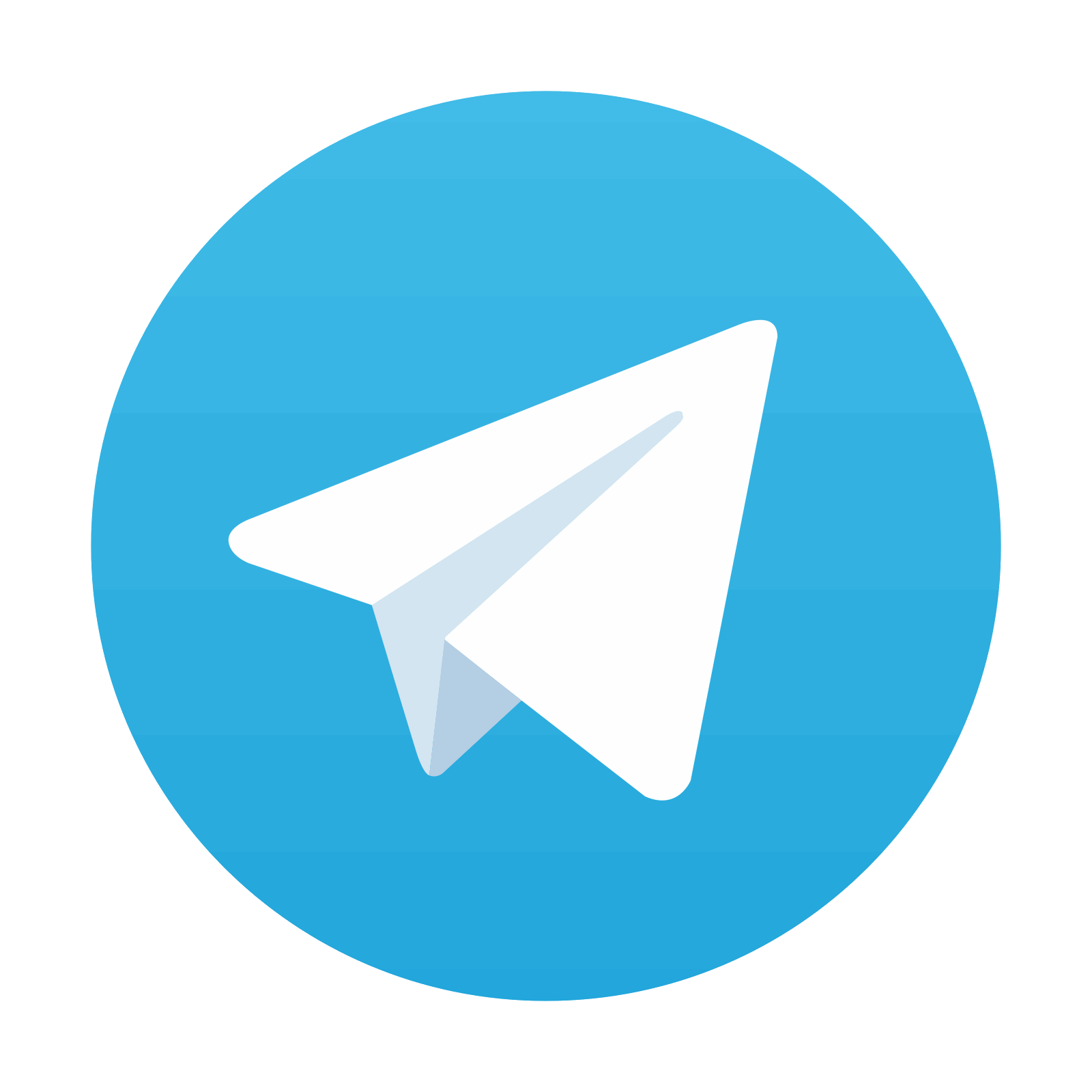
Stay updated, free dental videos. Join our Telegram channel

VIDEdental - Online dental courses
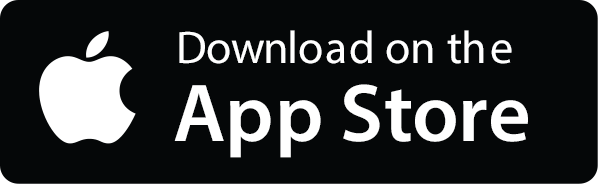
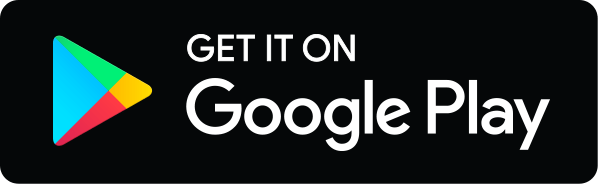