Graphical abstract

Highlights
- •
Cold Atmospheric Plasma (CAP) is an emerging technology with a great potential in implant dentistry.
- •
CAP is suitable for degrading oral biofilms formed in situ on Titanium (Ti) surfaces, without causing alterations to Ti surface topography.
- •
CAP cleaning efficacy is greater on machined than on moderately rough discs.
- •
CAP did not show any additive effect with air abrasion (AA) in the in vitro model used in the present study.
Abstract
Objectives
The aim of the present study was to assess the decontamination efficacy and titanium surface alterations of erythritol based air abrasion (AA) and cold atmospheric plasma (CAP) targeting a human complex biofilm.
Methods
Saliva collected from a peri-implantitis patient was used to develop in vitro human biofilm over titanium discs with machined (group A) and moderately rough (group B) surface. The discs were treated with AA, experimental CAP or a combination of both treatments (COM). The amount of biofilm on the discs was measured by crystal violet (CV). Surface features and roughness before and after treatment were assessed by SEM and laser profilometry, respectively. The data were statistically analyzed using Kruskal Wallis followed by Dunn’s multiple comparison test after being checked for normality by Shapiro-Wilk test.
Results
All the discs in group A performed better to treatments compared to group B. In both groups, CV data showed significantly lower amount of biofilm after AA treatment compared to CAP ( p < 0.05). Cleaning efficacy revealed relevant decontamination of both the surfaces following AA and COM treatments and almost complete biofilm removal after AA application on group A (99.92%). SEM analysis demonstrated no post-treatment alterations on the discs and laser profilometry did not show statistically significant changes in S a and S dr values.
Significance
Decontamination with AA delivering erythritol with or without CAP is highly effective in biofilm removal from different titanium surfaces. All the tested treatments, including CAP showed no noticeable alterations of the titanium discs surface features. Further in vivo studies are necessary to understand the potential of CAP technology in implant surface decontamination.
1
Introduction
The 2017 World Workshop on the Classification of Periodontal and Peri-implant Diseases and Conditions has newly introduced peri-implantitis as a plaque-associated pathological condition occurring in tissues around the dental implants, characterized by inflammation in the peri-implant mucosa and subsequent progressive loss of supporting bone . When a dental implant is inserted into the bone, its coronal portion might become exposed to the contaminated oral environment due to either crestal bone remodeling during post-operative healing or a supra-crestal positioning of the implant neck or an inefficient soft tissue seal. In these circumstances, microbial accumulation and development of a biofilm can occur within minutes, in which surface characteristics of implant may have crucial roles. Bermejo et al. recently demonstrated that implant surfaces with moderate-roughness have accumulated a significantly greater biomass and higher number of pathogenic bacteria, when compared to implant surfaces with minimal roughness, using an in-vitro biofilm structure. This is an important issue as roughness can be considered a double-edged sword: it is fundamental to achieve a better osseointegration with a modified implant surface, but at the same time the modified surface may promote greater microbial growth and biofilm formation leading to chronic inflammation in around the implant. Besides, the threaded design of the dental implant coupled with surface roughness is not easily accessible for infection control by oral hygiene practices, especially in non-surgical approach , makes the peri-implantitis as a much complex issue for researchers, clinicians and patients.
Hence, methods for decontamination of implant surfaces with varying roughness are of great technical and therapeutic interest for the treatment of peri-implantitis; particularly structure-preserving methods which pursue the ideal goal to obtain a complete cleaning of contaminated implant surface without destroying the surface topography but re-establishing surface characteristics promotive for re-osseointegration .
Despite implant features and roughness have been enhanced and increased over the years to optimize adequate osseointegration, it is a greater challenge to treat a rough implant surface compared to smooth implant surface . The currently available techniques for implant surface decontamination have advantages and disadvantages, and we are still in pursue of the optimal treatment protocol for the management of peri-implantitis.
Given the potentially limited effect of mechanical debridement, adjunctive therapies using air-abrasive (AA) units, laser devices, and chemotherapeutic anti-infective agents have been trialed. A recent systematic review on the effects of AA decontamination on in vitro titanium surfaces reported that AA is a safe and effective treatment method to clean implant surfaces. Furthermore, newer powders on the market, such as erythritol, were also found to be superior in cleaning efficacy and biocompatibility preservation than traditional powders .
Cold atmospheric plasma (CAP) treatment is an emerging technology, that could act as an adjunct to the conventional therapy for peri-implantitis. Indeed, its use in the medical context to decontaminate bacteria, promotion of wound healing and to target oncological lesions has been documented . Plasma is the fourth state of matter, naturally energetic as electrons are stripped from atoms. It is an electrically neutral, ionized gas composed of ions, electrons, neutral species, ultraviolet irradiation, free radicals and chemically reactive neutral species . It can be controlled by magnetic and electric fields and directed onto targeted areas . Low-temperature plasmas can be classified as “thermal” or “non-thermal”, while cold (typically less than 40 °C at the point of application) atmospheric plasma (CAP) sources provide the possibility to extend the plasma treatment to living tissue . Dental applications of CAP include dental caries, sterilization, elimination of biofilms, root canal disinfection, increases in bond strength at the dentin/composite interface, bleaching, implant surface preparation, wound healing promotion, blood coagulation and many others will be available in the near future. This has been possible due to the bactericidal activity of plasma and partly due to its easy access into narrow and confined spaces . The characteristics of CAP that interests oral health practitioners in the treatment of peri-implantitis include its safety; ability to decontaminate bacteria; minimal surface alteration and wound healing promotion. In addition, treatment protocols that predictably support re-osseointegration of implants with peri-implantitis, are also possible. It was shown that CAP was able to enhance the wettability of implant surface, leading to an improved osteoblasts adhesion, spreading and subsequent growth on dental implants of various surface topologies.
In the context of peri-implantitis treatment, two critical aspects that should be carefully considered are the intra-oral cleaning of the bacterially contaminated implant surface and the re-establishment of surface characteristics which promotes re-osseointegration. The aims of the present in vitro study are: (i) to compare the efficacy of bacterial decontamination of different treatment modalities incorporating AA and CAP alone and in combination on machined and moderately rough Ti surfaces; and (ii) to compare the effect of those decontamination treatments on titanium surface before and after treatments.
2
Materials and methods
2.1
Titanium materials
A total of 112 Ti discs (Grade 4 Titanium diameter 10 mm, thickness 1.5/2 mm) with two different surfaces were used:
- –
group A: 58 machined surface, S-SMOOTH-2;
- –
group B: 54 rough alumina particles – blasted surface, S-MROUGH-2.
All discs were kindly provided by Southern Implants, Irene, South Africa.
2.2
Study design
The protocol of the present study was approved by the Griffith University Human Research Ethics Committee (GU Ref No: 2018/135).
Both groups (A and B) were subdivided into 5 treatment groups ( Fig. 1 a and b) :
- –
-ve control: non-contaminated and treated titanium discs by AA and CAP;
- –
+ve control group: contaminated and untreated discs;
- –
AA: contaminated and treated by AA;
- –
CAP: contaminated and treated by CAP;
- –
COM: contaminated and treated by the combined AA and CAP treatment;

2.3
Biofilm growth
A systemic healthy volunteer with no evidence of active caries, no salivary gland disease but affected by peri-implantitis was selected to donate his saliva. The subject was asked to refrain from practicing oral hygiene routine for 12 h before saliva collection in the morning. The subject chewed on paraffin wax until 5 ml of saliva was collected.
To generate biofilm on the titanium discs in-vitro , each discs were incubated, in 1 mL of Brain Heart Infusion (BHI) media (OXOID CM1135, 37 g/L) supplemented with 5% defibrinated sheep blood and 10% human saliva from the donor , in sterile 24 well plates (Costar 3524). The culture plates were incubated for 96 h under anaerobic conditions at 37 °C, 80 rpm to allow bacterial growth. For negative controls, titanium discs were incubated at same conditions with 1.0 ml of BHI supplemented with 5% defibrinated sheep blood and 10% PBS solution, instead of human saliva.
2.4
Instrumentation modalities
2.4.1
Air abrasion
Groups A and B discs were treated with an AA device (Airflow, EL-308/C, EMS Nyon, Switzerland) at a static pressure of 7 bar (101.5 psi) using erythritol powder (particle size 14 mm) for 20 sec, perpendicular to the disc, at a distance of 10 mm and 60 mL water/min. The standard handpiece was mounted with a holder so as to keep the nozzle at a static position to each titanium discs treated. Each titanium disc then received treatment by being consistently rotated opposing the nozzle from the centre to the periphery in four circular motions ( Fig. 2 a and b) . All treatments were performed by the same experienced operator, a senior Periodontics Specialist in training (W.L.H.). After instrumentation all the discs were cleaned with water.

2.4.2
Cold Atmospheric Plasma (CAP)
Plasma treatment was performed with an atmospheric experimental plasma pen jet described below. The device mainly consists of four parts: a high voltage direct current (HVDC) power source, a resistance selector, a 0.33 μF storage capacitor (C) and a plasma reactor. The HVDC power source can transform the commercial 240 V AC signal to a DC high voltage signal with the magnitude of 0–10 kV. The HVDC voltage is output with positive polarity connected to the resistance selector and negative one grounded. The resistance selector consisted of a single-pole multi-throw switch and three resistors with different value of R 1 = 2 MΩ, R 2 = 1 MΩ and R 3 = 0.5 MΩ. Only one resistor is connected in the circuit when device is working. The capacitor and the plasma reactor are connected parallel between the resistance selector and the ground ( Fig. 3 ).

For safe handheld operation, the plasma reactor is wrapped with a black rubber sleeve and fixed in plasma pen. The reactor consists of three parts: a high-voltage electrode, a grounded electrode and an insulating sleeve. The high-voltage electrode is a 60 mm-in-length and 1.5 mm-in-diameter copper rod and is connected to the variable resistance switch. The copper rod head is grinded into a needle to decrease the breakdown voltage. The grounded electrode is made of stainless steel with the outer and inner diameter of 9 mm and 7 mm, respectively. It also has a 3 mm-in-diameter hole in the head. As marked in Fig. 4 the distance between the head of high-voltage and grounded electrodes, namely the spark gap, varies from 2 mm to 7 mm. The insulating sleeve, made of polytetrafluoroethylene, is used to fix and insulate the two electrodes. The spark discharge generated in the spark plasma pen is pulsed. When the device is on, the energy from HVDC power source is transferred through the variable resistance switch and is stored in the capacitor, thus increasing the voltage across the capacitor (C) and the spark gap. Once the voltage reaches the breakdown threshold of the spark gap, the stored energy is consumed to drive and maintain the discharge, and the voltage falls down immediately. As soon as the energy cannot maintain the discharge, another discharge cycle starts, and energy is again restored in the capacitor.

Plasma treatment was performed with the spark plasma pen on a standardized holder keeping a distance of 5 mm from the plasma nozzle tip (end of discharge capillary) to the titanium discs surface; it was operated with the pulse repetition rate of 1.4 Hz. A high RF-voltage (10 kV) was coupled to the needle electrode and temperature measurement yielded a maximum of 2 °C at the tip of the plasma jet. All titanium discs were submerged in PBS solution with the liquid medium just matching the discs surface at either 1.5 mm/2 mm according to the thickness of the discs.
2.4.3
Optical and electrical measurements
Optical emission spectroscopy (OES) was observed by the OES device (USB2000+, Ocean Optics, USA) in the range from 300 to 900 nm. The optical emission spectrums were detected 20 mm away from the exit of the glass tube and with 0.5 ms exposure time.
The voltage-current waveforms of spark discharge and the discharge current were measured using a Tektronix P6015A high-voltage probe and a Pearson Current Monitor 6595 current coil, respectively, and they are recorded by a Tektronix TDS3045c digital oscilloscope.
2.5
Biofilm quantification
Eight discs in each subgroup belonging to group A and 7 discs in each subgroup belonging to group B as well as 5 discs for the negative controls were assessed through crystal violet (CV) assay analysis for the evaluation of the effect of the different treatment modalities on biofilm removal; experiments were repeated in triplicates.
After the growth media was discarded, discs were first washed twice with 500 μL of sterile distilled water. The biofilm was then stained with 500 μL of 0.1% crystal violet (Sigma-Aldrich, Castle Hill, NSW, Australia) for 30 min at room temperature in a 24 well plate. The discs were then washed four times with 1 mL of sterile distilled water and removed maximum water possible by tapping onto paper towel. The dye bound to adherent biofilm was then solubilized using ethanol to acetone ratio of 80%:20% and the optical density (OD) of the solubilized dye was measured at 595 nm with a plate reader (POLARstar Omega plate reader, BMG Labtech, Adelaide, Australia) with 10 measurements taken for each well. Ethanol to acetone ratio was used as the blank to subtract any background reading. The percentage reduction in biofilm caused by the treatment was calculated as followed: ((OD +vecontrol − OD treatment )/OD +ve control ) × 100%.
2.6
Measurement of surface roughness
Two samples in each subgroup were used for laser confocal profilometry microscopic (LCPM) assessment after CV analysis. Surface roughness quantification of the titanium discs before and after the treatments was performed with an industrial LCPM (Olympus, LEXT OLS5000, Japan). LCPM images were analyzed using the OLS50-SW-Analysis software to obtain the mean roughness S a and S dr parameters.
2.7
Qualitative assessment of surface topography
Pristine discs were evaluated to provide a baseline surface assessment and description prior to group allocation; in addition, 4 titanium discs in each of the subgroups were allocated for subsequent scanning electron microscope (SEM) analysis. The samples were rinsed with 0.1 M of phosphate-buffered solution (PBS) at pH 7.1 and fixed overnight with a 4% PBS–paraformaldehyde solution at 4 °C. Samples were further washed with PBS buffer and dehydrated using an ascending alcohol series before mounting onto aluminum stubs and gold sputtering in an Emitech K 550 (Emitech Ltd., Ashford, Kent, UK).
A SEM with 10 kV and 3.3 A was used to capture images of the disc surface before and after the treatments. These images were used to examine any alteration on the titanium surface and were captured at 3, 6, 9, and 12 o’clock positions, at a distance of 300 mm from the center of the disc. Two magnifications of micrographs were used: 270×, 2700×.
2.8
Statistical analysis
Statistical analyses were carried out using a specific software (GraphPad, California, USA). Data distributions were checked for normality by Shapiro-Wilk test (Table S1a–b and Table S2a–d). Kruskal Wallis followed by Dunn’s multiple comparisons test was used to compare the differences among groups. Data were presented as medians and box plots showing upper and lower quartiles and confidence intervals. Statistically significant differences were set to P < 0.05.
3
Results
3.1
Plasma plume
Fig. 5 shows the photographs of discharge in the spark plasma pen under different spark gaps. The magnitude of voltage output from HVDC is fixed at 10 kV. A bright plume is observed to propagate for around 1 cm from the nozzle in the grounded electrode. The length of plume is sensitive to the spark gap. When the gap is increased from 2 mm to 5 mm, the length of plume rises from 13 mm to 19 mm, but it falls to 12 mm when the gap continues to increase to 7 mm. Since the length of the plasma jet is an important parameter which can influence its treatment effect, the spark gap is optimized to be around 5 mm for a longer plume.
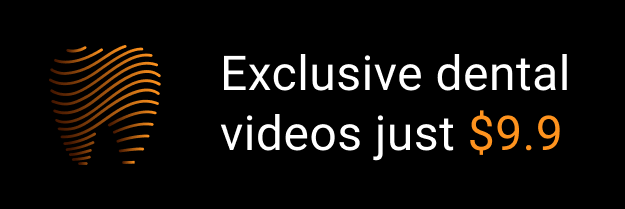