Highlights
- •
Dental Fiberglass posts are inadvertently damaged by the dentist during trimming.
- •
Imaging with synchrotron X-ray refraction radiography reveals nanoscale fiber flaws.
- •
Phase contrast-enhanced CT reveals μm cracks, splinters and fractures due to trimming.
- •
The outer layers of fibers in fiber posts are prone to fracture, shattering and delamination.
- •
Partial-coherence X-ray imaging facilitates quantification of structural damage in posts in 3D.
Abstract
Objectives
There is concern that the integrity of fiberglass dental posts may be affected by chairside trimming during treatment. We hypothesize that hard X-ray methods of phase contrast-enhanced micro-CT (PCE-CT) and synchrotron based X-ray refraction (SXRR) can reliably identify and help characterize the extent of damage.
Methods
Fiberglass posts were imaged both as manufactured and following trimming with a diamond bur. Each of the posts was imaged by SXRR and by PCE-CT. Datasets from PCE-CT were used to visualize and quantify 2D and 3D morphological characteristics of intact and of damage-affected regions caused by trimming.
Results
The SXRR images revealed fiber inhomogeneities from manufacturing with a significant increase in internal surfaces in sample regions corresponding to damage from trimming. PCE-CT volumes unveiled the micromorphology of single fibers in the posts and some damage in the trimmed area (e.g. fractures, splinters and cracks). Area, perimeter, circularity, roundness, volume and thickness of the glass fibers in the trimmed area were statistically different from the control (p < 0.01).
Significance
The integrity of single fibers in the post is critical for bending resistance and for long-term adhesion to the cement in the root canals. Damage to the fibers causes substantial structural weakening across the post diameter. Glass fragments produced due to contact with the dental bur may separate from the post and may significantly reduce bond capacity. The above mentioned synchrotron-based imaging techniques can further facilitate assessment of the structural integrity and the appearance of defects in posts (e.g. after mechanical load).
1
Introduction
Dental fiberglass posts are composites made of axially aligned glass fibers bound together with a reinforced polymer matrix. Outside the field of dentistry, fiber reinforced composites find extensive application [ ] due to the low density and enhanced mechanical performance. In dentistry, fiberglass dental posts are used as flexible and light reinforcements to support post-endodontic restorations, benefiting from the high strength of the millimetre (mm) long glass fibers with micrometer (μm) diameters. Dental posts are used for tooth restoration following root canal treatment and extensive tooth tissue destruction e.g. by caries [ ]. Posts are placed in and bonded to the cleared root canal where they contribute to mechanical retention of artificially restored dental crowns. Fiberglass posts have become widely used both due to their aesthetic benefits and due to a favorable elastic modulus that well matches that of the tooth substrate [ ]. Such modulus matching results in reduced stress differences between the natural tooth substrate and the artificial dental material, when cyclically loaded during tooth function.
Fiberglass posts are usually mass produced in standard lengths and thicknesses, though alternative chair-side manufactured options are increasingly attracting interest [ ]. Thus, to fit roots with biologically-varying anatomies, either the tooth or the post must be trimmed. Cutting away healthy dental tissues to receive a post weakens the tooth structure, which may endanger and worsen prognosis of treatment [ ]. A more conservative solution favored by dental practitioners is to prune and grind the post, primarily its length [ , ]. Most commonly, fiberglass posts are pruned using a high-speed handpiece with a diamond bur although manufacturers also recommend using a carborundum disk [ ]. However, to maintain the superior mechanical properties, the post must remain structurally intact [ , ]. The effects of trimming fiberglass posts are poorly understood [ ], especially when it comes to the long-term durability during cyclic loading (mastication), although, it can be assumed that flaws within the post structure are detrimental to tooth prognosis.
Different experimental methods have been used to evaluate the physical characteristics of dental posts, including strength, elastic modulus and fracture resistance [ ]. Nonetheless, assessing the internal integrity of the post is challenging especially when seeking causal links between structural defects and restoration failure [ , ]. Mapping flaws in dental posts has, to date, been mainly reported using serial slicing of samples and by imaging using different microscopes [ , ]. Consequently, it is difficult to assess how much of what was reported previously is due to sample preparation, specifically the emergence of diffuse damage.
For non-destructive evaluation of fiber-reinforced posts, there exist several well-established methods of investigation [ ]. Of those, absorption-based X-ray computed tomography is highly relevant, since it produces 3D images of the undisturbed structure, an essential step on the path to determine relationships between structural defects and potential failures [ ]. The matrix and glass fibers typically present low attenuation and they are usually transparent in X-ray images. Often, heavy-element filler particles included into the matrix surrounding the fibers, increase the visibility of the post when imaged radiographically by medical X-rays. Phase contrast-enhanced micro-computed tomography (PCE-CT), which exploits the “laser-like” attributes of partial-coherence X-ray sources (available e.g. in shared large-scale synchrotron research facilities), provides high-contrast images, well-suited to study defects in fiber reinforced composites [ ]. Small differences in density along the X-ray path can lead to strong contrast enhancement at interfaces between different material phases, e.g. matrix and glass fibers [ ]. Due to the μm-scale diameters of the fibers in dental posts, PCE-CT imaging at micron-scale resolution shows great promise to map the spatial distribution of the post components. PCE-CT is typically acquired using beamlines in synchrotron radiation facilities where CT setups exploit parallel beams. Such PCE-CT is limited to a resolution of ∼1 μm, which may be insufficient to detect small, submicron damage within the glass fibers themselves. X-ray refraction (XRR) techniques [ ] are a group of radiographic and tomographic methods that combine a capacity to image large fields of view (several mm in width and height) with exceptional sensitivity to small with sizes in the nanometre (nm) range [ , ]. Synchrotron XRR radiography uses analyser-based imaging, also known as ‘diffraction-enhanced imaging’ [ ]. Employing a Bragg-reflection configuration as the X-rays propagate towards the detector, and due to differences in the path of X-rays scattered from internal cracks and gaps, changes in interference effects can be recorded digitally. The refracted intensity patterns can be directly linked to specific kinds of investigated flaws, for example internal cracks and air voids [ ]. Moreover, XRR has been shown to successfully detect fiber-matrix debonding in lightweight materials e.g. carbon or fiberglass-reinforced plastics [ , ]. XRR may therefore help push the boundaries of sensitivity with regards to detection of internal defects in dental posts based on various observations with this method [ ].
The aim of the present study is to non-destructively explore possible damage to fiberglass dental posts following dental chairside diamond-bur trimming. We hypothesize that phase-sensitive highly-penetrating hard X-ray methods of PCE-CT and XRR can help characterize internal defects in dental fiber posts.
2
Materials and methods
2.1
Sample characteristics and preparation
Free-standing as well as canal-cemented fiberglass posts were imaged, intact and after pruning, to identify geometry and to optimize PCE-CT imaging conditions. Three isolated fiberglass posts were then selected for reference and treatment samples, followed by imaging with both XRR and PCE-CT methods. The dental fiberglass posts (Dentin Post, ER System, size 090, Komet, Lemgo, Germany) comprise an epoxy matrix, glass fibers and radiopaque fillers (e.g. Barium oxide) shaped into a tapered cone, 12 mm long (Lot number: 312850). One sample was used as control and was imaged as received in an intact state (‘Control’ sample). The two other samples were trimmed using a regular grain cylindrical diamond bur (diamond bur n. 835.314, Brasseler, Lemgo, Germany) using a high-speed handpiece creating (a) a single side-to-side lateral groove across the post shaft (‘Grooved’ sample) and (b) apical thinning employing ‘bevel shaping’ in the narrow region near the tip (‘Beveled’ sample). Air cooling was used, due to a need to avoid wetting of the post so as to minimize X-ray imaging artefacts e.g. due to evaporation or sample motion due to water diffusion. The used bur and the intact sample were also imaged in an SEM with the backscatter imaging mode (density contrast) of a PhenomXL scanning electron microscope (Thermofisher, Eindhoven, The Netherlands).
Each of the posts was imaged on BAMline [ , ], the imaging beamline at Bessy (Berliner Elektronenspeicherring-Gesellschaft für Synchrotronstrahlung) HZB — Helmholtz-Zentrum Berlin, Germany. For XRR measurements, the samples were mounted in a slide frame ( Fig. 1 a). For PCE-CT, each sample was attached to a metal stub and scanned with repeated rotation (spanning 180°) on a high-resolution imaging air-bearing sample stage [ ] ( Fig. 1 b).



2.2
Synchrotron X-ray refraction radiography (SXRR) measurements
Measurements were performed on the BAMline SXRR setup, where an analyzer crystal, mounted between the sample and an off-axis detector, can be tilted to measure rocking curves. Such curves were used to identify maximum scatter from inner surfaces within each sample ( Fig. 1 a). From the maximum and integral intensities ( I max and I int ) of each rocking curve the refraction value C of a sample of thickness d is calculated (for each pixel of the camera) according to:
where the index 0 stands for reference measurements performed without the sample in the beam. C is proportional to the internal specific surface, i.e. the surface per unit volume in the path of the beam. To eliminate the influence of sample thickness on C, it is custom to normalize Eq. (1) by the sample linear attenuation μ * d = ln( I int,0 / I int ), where I int and I int,0 are the transmitted intensities measured both with and without the sample in the beam. C /μ yields normalized refraction values (henceforth termed “relative specific surface”). For the experiments reported here, a photon energy of 30 keV and a pco.1600 camera system (1600 × 1200 pixel) with a nominal pixel size of 4.08 μm × 4.08 μm was used. Note however, that the setup is sensitive to flaws that are far smaller than the imaging pixel size. The direction of the incident beam onto the Si(111) analyzer crystal and the direction of the beam reflected by the analyzer crystal define the scattering plane ( Fig. 1 ). The analyzer crystal only discriminates the components of refracted X-rays propagating within the scattering plane, therefore, SXRR radiography is usually performed by mounting the samples in two orthogonal orientations to detect the location and orientation of the defects (pores, cracks, etc.). Accordingly, the fiber posts were imaged in two orthogonal orientations: fiber posts axis perpendicular (marked , Fig. 1 a left) and parallel (marked
, Fig. 1 a right) to the scattering plane of the analyzer crystal. This defines two sensitivity maps: the perpendicular orientation is sensitive to inner surfaces that are oriented parallel to the long axis of the glass fibers in the post (henceforth termed ‘perpendicular orientation’); the parallel orientation is sensitive to inner surfaces that are oriented perpendicular to the axis of the post (henceforth termed ‘parallel orientation’).
2.3
Phase contrast-enhanced micro-computed tomography (PCE-CT)
Each sample was positioned on the high-resolution BAMline rotation stage of the micro-CT setup, where it was scanned using inline propagation-based phase contrast-enhanced microtomography, as previously reported [ , ]. A sample-detector distance of 45 mm ( Fig. 1 b) was used with an X-ray photon energy of 34 keV, with detector binning to reach effective pixel sizes of 876 nm. By rotating 180° around the sample axis, 1800 radiographic projections were recorded using 500 ms exposure times.
2.3.1
3D reconstructions
All tomographic scans were normalized by empty-beam reference images and were then reconstructed by the back projection method (Octopus V8.5, Zwijnaarde, Belgium) [ ] following single-distance phase-retrieval for contrast enhancement, calculated using Fourier filtering by the ANKAphase Fiji plugin [ ] (delta/beta ratio of 50).
2.3.2
Image processing and segmentation
The reconstructed datasets were visualized using ImageJ (ImageJ 1.52d, National Institutes of Health, USA), and Amira (AmiraZIBEdition version 2020.48, Zuse Institute Berlin (ZIB), Germany, and Thermo Fisher Scientific, Bordeaux, France). The histograms of the gray-values from all samples were examined to verify the intensity range corresponding to the different materials comprising the fiberglass posts. The full dataset of the reference post (Control) and cropped volumes of the Grooved post (about 500 μm in all directions extracted near the surface affected by the bur) were segmented and analyzed in both 2D and 3D.
2D segmentation and quantification was accomplished using ImageJ (for further details see Appendix A and Supporting information Fig. S4). Following separation of fibers from the matrix, cross-sectional slices were analyzed with the “Particle Analysis” module to calculate area, perimeter, circularity and roundness of each of the fibers along a stack of images down the fiber axis. Voronoi tessellation was applied to quantify the distance between virtual cross sections of fibers in each slice in 2D. 3D segmentation was performed using the software package Amira following the procedures described previously by Baum et al. [ ] (for further details see Appendix B and Supporting information Fig. S5). Volume, tortuosity and thickness of each fiber were assessed in 3D. A custom module of Voronoi tessellation was implemented to generate maps of variations in distance along the fiber longitudinal axes.
3
Results
The SXRR images, normalized by the spatially-varying sample attenuation maps (see Supporting information Fig. S3) revealed local variations in interfaces and highlight specific regions containing sub-μm damage/internal surfaces. Fig. 2 shows gray-scale images of the relative specific surfaces (C/μ) of the Control, Grooved and Beveled posts. The complementary perpendicular ( ) and parallel (
) orientations generate images that reveal strong local differences in brightness, which is due to the distribution and orientation of internal surfaces. The stronger signal is depicted quantitatively in the gray-scale values, which, for the perpendicular orientation, shows significantly higher scattering originating from the dominant interfaces oriented parallel to the glass fibers in all 3 posts ( Fig. 2 a–c left) . The mean value of the relative specific surface in this post orientation is ∼4 times higher than the mean value measured perpendicular to the glass fiber orientation ( Fig. 2 a–c right). Substantial scattering thus takes place along the interfaces between the reinforcing glass fibers and the matrix. The mean C /μ-value of ‘regular’ post regions is about 0.38 and varies from 0.27 in the dark to 0.67 in the brighter stripes. Dark stripes indicate areas with better fiber-matrix bonding, light areas suggest local defects in bonding [ , ]. Since these are 2D images similar to radiography, little can be concluded about the spatial distribution of these defects. The SEM image (see Supporting information Fig. S2) shows that at least some of these defects are present on the external surface of the post due to manufacturing.



The Grooved and Beveled samples show regions where the post structure was disrupted by contact with the diamond bur. In regions that came into contact with the grinding drill, a very bright signal can be seen that arises from a high density of internal defects and cracks. The lateral extent of damage is visible in both parallel and perpendicular orientations and seemingly extends across several fibers. The fact that for the most part, posts in the parallel orientation ( Fig. 2 a–c right) show a very low C/μ ratio of about 0.05 suggests almost no internal scatter-inducing inhomogeneities oriented perpendicular to the main fiber axis. This means that both the matrix and the glass fibers are continuous with negligible pores and cracks on the order of ×10 to ×100 smaller than 4 μm nominal resolution of the SXRR images (i.e. deep into the nm range). It also means that the fibers are completely continuous along their long axis corresponding to the longitudinal direction of the post, making them ideal for sustaining, bending and tension resistance. This observation however no longer holds when examining the regions that came into contact with the diamond bur. Damage to the glass fibers and matrix is encoded in the huge local increase in C/ μ-values, exceeding 0.8.
The damage caused by the drill is clearly visible in both parallel and perpendicular orientations of the posts with respect to the analyzer scattering plane. The internal damage is therefore not limited to debonding along the fiber-matrix interface. In some regions, the local C/μ value increases up to 1.0 and the bright halos suggest that damage encompasses a volume extending at least 50 μm away from the post surface that came into contact with the dental drill. This should not be confused with the sporadic observations of higher, relative specific surface values seen on the outer rims of the posts. Such bright areas are due to fiber inhomogeneities from the manufacturing process, leading to micro-debonding at the periphery, accompanied by occasional glass-fiber damage. SEM close-ups of the fiber outer surface (Supporting information Fig. S2) show examples of delaminations of the matrix with localized exposure of fibers. SEM also shows that exposed glass fibers occasionally fracture or become locally shattered.
Due to limits of resolution and contrast, we were not able to resolve the fibers in the fiberglass posts scanned in a laboratory micro-CT (Supporting information, Fig. S6). PCE-CT revealed the architecture of the glassy fibers that can easily be followed in 3D within the reconstructed volumes (Supporting information: Videos V1–V3). Within the resolution limit (∼1 μm), fibers, matrix and voids could be distinguished. Occasionally, clusters of filler particles (various oxides) are observed in the matrix ( Fig. 3 c, white ellipse). Such filler particles have an appearance that is similar to the contrast of the fibers, but are much smaller in size. In 2D, they were identified (and filtered out) as being particles with diameters smaller than 6.5 μm, corresponding to 3D objects with volumes smaller than ∼300 μm 3 . Exemplary virtual cross-sectional slices from a final volume are shown in Fig. 3 .
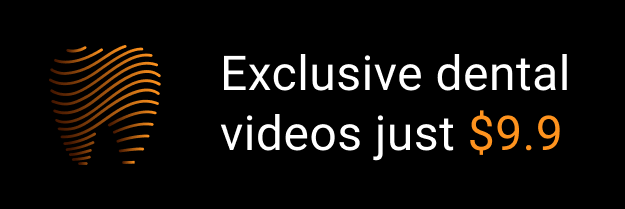