Highlights
- •
Adhesives with improved biological and physicochemical properties were formulated.
- •
Adhesives with higher long-term μ-TBS after aging for 1 year were achieved.
- •
Nanotubes with triazine compound provided antibacterial activity for the adhesives.
- •
All adhesives formulated presented no cytotoxicity against human pulp cells.
Abstract
Objective
Formulate experimental adhesives containing titanium dioxide nanotubes (nt-TiO 2 ) or titanium dioxide nanotubes with a triazine-methacrylate monomer (nt-TiO 2 :TAT) and evaluate the effect of these fillers on the physical, chemical, and biological properties of the adhesives.
Methods
First, nt-TiO 2 were synthesized via a hydrothermal method. The nt-TiO 2 were mixed with a triazine-methacrylate monomer (TAT) to formulate nt-TiO 2 :TAT, which were characterized by transmission electron microscopy (TEM). The nt-TiO 2 , TAT, and nt-TiO 2 :TAT were evaluated via Fourier Transform Infrared, Ultraviolet–visible, and micro-Raman spectroscopies. An experimental adhesive resin was formulated with bisphenol A glycerolate dimethacrylates, 2-hydroxyethyl methacrylate, and photoinitiator/co-initiator system. nt-TiO 2 or nt-TiO 2 :TAT were incorporated at 2.5 wt.% and 5 wt.% in the adhesive. The base resin without nt-TiO 2 or nt-TiO 2 :TAT was used as a control group. The adhesives were evaluated for antibacterial activity, cytotoxicity, polymerization kinetics, degree of conversion (DC), Knoop hardness, softening in solvent (ΔKHN%), ultimate tensile strength (UTS), 24 h- and 1 year- microtensile bond strength (μ-TBS).
Results
TEM confirmed the nanotubular morphology of TiO 2 . FTIR, UV–vis, and micro-Raman analyses showed the characteristic peaks of each material, indicating the impregnation of TAT in the nt-TiO 2 . Adhesives with nt-TiO 2 :TAT showed antimicrobial activity against biofilm formation compared to control (p < 0.05), without differences in the viability of planktonic bacteria (p > 0.05). All groups showed high percentages of pulp cell viability. The polymerization kinetics varied among groups, but all presented DC above 50%. The addition of 5 wt.% of nt-TiO 2 and both groups containing nt-TiO 2 :TAT showed higher values of Knoop hardness compared to the control (p < 0.05). The groups with nt-TiO 2 :TAT presented lower ΔKHN% (p < 0.05) and higher UTS (p < 0.05) than the control group. After one year, the group with 5 wt.% of nt-TiO 2 , as well as both groups containing nt-TiO 2 :TAT, showed higher μ-TBS than the control (p < 0.05).
Significance
The mixing of a triazine-methacrylate monomer with the nt-TiO 2 generated a filler that improved the physicochemical properties of the adhesive resins and provided antibacterial activity, which could assist in preventing carious lesions around tooth-resin interfaces. The set of physical, chemical, and biological properties of the formulated polymer, together with the greater stability of the bond strength over time, make nt-TiO 2 :TAT a promising filler for dental adhesive resins.
1
Introduction
The replacement of dental tissues with resin composites is a common procedure performed when patients are affected by caries [ ]. However, the lifespan of direct technique with resin composites remains about five years [ ], leading to materials replacement and a spiral sequence of restorative treatments [ , ]. A greater extent of dental tissues may be lost over time due to the progressive substitution of unsuccessful composite restorations, jeopardizing patients’ oral health conditions [ ]. Materials fracture and recurrent caries around dental-resin interfaces are the major reasons for the failure of composite restorations [ ].
The development of caries seems to be the most important factor related to the survival of composite restorations after the first five years since their placement [ ]. While the annual failure rate (AFR) of direct composite restorations was reported as 4.6% for high-caries-risk patients, this value was reported as 1.6% for low-caries-risk patients. This difference led to cumulative survival values of around 35% for those with high risk and 60% for low risk after 20 years [ ]. Furthermore, knowledge about caries prevention is an essential factor that positively affects the survival of direct restorations [ ]. Therefore, the quality of restorations depends on individual factors, and clinical patient-centered approaches must always be in focus [ ]. However, restorative materials with antibacterial properties could be adjuvants in this process, may assisting in decreasing the AFR in those patients who present greater difficulty in controlling biofilm.
Another essential factor for restoration survival is the quality of the bonding to the hard-dental structures, which is influenced by the mechanical and chemical properties of the adhesive and the hybrid layers formed [ ]. The hybrid layer is composed of a collagen network embedded by adhesive resin, and it represents the weakest site of the restoration set [ ]. Mechanical forces application, patient-related variables, and a deficit of appropriate marginal sealing make the restoration prone to loss its mechanical stability and increase its degradation [ ]. Marginal gaps caused by polymer degradation and polymerization shrinkage, together with increased roughness of composites over time [ ], influence the development of recurrent caries adjacent to the bonding interface. Within this framework, efforts should be addressed to enhance the quality of the hybrid layer formed, improving the mechanical properties of dental adhesives, and providing antibacterial activity for this class of material.
Inorganic filler addition has been a strategy investigated to improve the physicochemical and biological properties of adhesive resins [ ]. Researches on dental bonding have been progressively demanding inorganic nanoparticles due to their small size and high surface area, which may be an advantage to provide biointeractivity with dental tissues [ ]. Titanium dioxide (TiO 2 ) nanostructures were incorporated into dental materials to improve their physicochemical and biological properties. Hardness, flexural strength, degree of conversion, elastic modulus, shear bond strength, and antibacterial activity are among the superior features that TiO 2 can confer [ ]. Furthermore, TiO 2 presents bioactivity, which could provide remineralization ability for resin-based dental materials [ ]. Due to these properties, materials derived from TiO 2 have been highlighted as reasonable fillers to be added to dental materials. In this context, titanium dioxide nanotubes (nt-TiO 2 ) are nanocarriers that could provide TiO 2 properties to adhesives besides being a suitable method to decrease the release rate of therapeutic agents such as antibacterial molecules [ ]. nt-TiO 2 are biocompatible nanocarriers with elongated shape, tubular structure, and significant loading ability. These features make them attractive for drug delivery systems [ ].
Dental adhesives have been modified with antibacterial agents to prevent bacterial colonization at restoration margins [ , ]. Methacrylate monomers with antibacterial activity that are able to copolymerize with the resin may offer a long-term antibacterial effect because of their covalent bonding within the resin network [ ]. 1,3,5-triacryloylhexahydro-1,3,5-triazine (TAT) is a methacrylate monomer with three functional groups of carbon-carbon double bonds. This positively charged monomer was previously tested in dental formulations for orthodontic [ , ] and restorative [ ] purposes. TAT increased the resins’ stability against solvents and the bond strength to enamel, besides had demonstrated antibacterial effect against Streptococcus mutans [ , , ].
Based on the characteristics above mentioned of nt-TiO 2 and TAT, the incorporation of nt-TiO 2 into dental adhesives could be a strategy to confer superior mechanical properties besides providing antibacterial activity and bioactivity for the polymer. Moreover, nt-TiO 2 could serve as a drug carrier of other agents, such as TAT. Considering that TAT was already tested purely in methacrylate dental resins, inducing antibacterial effect, and improving the physical and chemical properties, it could be mixed with nt-TiO 2 . These properties led us to investigate nt-TiO 2 or nt-TiO 2 with a triazine-methacrylate monomer (nt-TiO 2 :TAT) as fillers. This study aimed to formulate experimental adhesive resins containing nt-TiO 2 or nt-TiO 2 :TAT and evaluate the effect of these fillers on resins’ physical, chemical, and biological properties. The null hypothesis to be tested is that the addition of nt-TiO 2 or nt-TiO 2 :TAT does not affect resins’ physical, chemical, or biological properties.
2
Materials and methods
2.1
Study design
In this in vitro study, five experimental adhesive resins were formulated and tested with different concentrations (2.5 or 5 wt.%) of two separated fillers: titanium dioxide nanotubes (nt-TiO 2 ) or titanium dioxide nanotubes mixed with a triazine-methacrylate monomer (nt-TiO 2 :TAT). The following dependent variables were evaluated: antibacterial activity against biofilm formation and planktonic bacteria, cytotoxicity against human pulp cells, polymerization kinetics, degree of conversion, Knoop hardness, softening in solvent, ultimate tensile strength, immediate and long-term microtensile bond strength (μ-TBS).
2.2
Preparation of fillers
The synthesis of nt-TiO 2 was the first step to prepare both fillers for the experimental adhesive resins. Fig. 1 A summarizes the process. A hydrothermal method was used with commercial TiO 2 P25 (1.0 g) and NaOH aqueous solution (10 M, 100 mL). The reagents were mixed in a hydrothermal autoclave reactor, and they were kept at 110 °C under autogenous pressure for 24 h. The product was washed with deionized water and filtered, followed by aqueous acidic solution wash (HCl at pH 1.6) and deionized water wash up to pH 7.0. The final product was dried under vacuum at 100 °C [ ].

To prepare the nt-TiO 2 :TAT, nt-TiO 2 were mixed with 1,3,5-triacryloylhexahydro-1,3,5-triazine (TAT) at a 1:1 ratio (by weight). Ethanol PA was added, and the mixture was kept under magnetic stirring until solvent evaporation. The produced powder was incubated in a desiccator at 25 °C for three days [ ]. The synthesized nt-TiO 2 :TAT material was evaluated by transmission electron microscopy (TEM, JEM 1200 EXII, JEOL, Tokyo, Japan). The produced powder was mixed in isopropanol, the solution was sonicated for 15 min, and it was dropwise (10 μL) onto a 200-mesh carbon-coated copper grid (SPI, West Chester, Pennsylvania, USA). The grid was incubated for 24 h in a desiccator, and the analysis was performed under an acceleration voltage of 80 kV. All reagents for filler preparation and analysis were purchased from Sigma-Aldrich Chemical Co. (St. Louis, Missouri, USA).
2.3
Chemical analysis of (TAT), nt-TiO 2 , and nt-TiO 2 :TAT
To evaluate a possible chemical interaction between TAT and nt-TiO 2 , the powders of TAT, nt-TiO 2 , and nt-TiO 2 :TAT were analyzed via Fourier transform infrared (FTIR), ultraviolet-visible (UV–vis), and micro-Raman spectroscopy. For FTIR analysis, the powders were placed on the attenuated total reflectance device (ATR) and analyzed with a spectrometer (Bruker model Alpha-P spectrometer) 32 scans, 4 cm −1 of resolution, in a range from 500 to 4000 cm −1 . UV–vis spectroscopy measurements were evaluated using a Shimadzu UV–vis Spectrometer UV-2450 in a diffuse reflectance mode, in a wavelength range from 200 to 800 nm. The band gap of nt-TiO 2 and nt-TiO 2 :TAT were calculated according to a previous study [ ]. For micro-Raman spectroscopy (SENTERRA, Bruker OptikGmbH, Ettlingen, Baden-Württemberg, Germany), the powders were analyzed using a 50x magnification, diode laser with 100 mW and 785 nm, ∼ 3–5 cm −1 resolution, in a range from 413 to 1775 cm −1 [ ].
2.4
Formulation of the experimental adhesive resins
Bisphenol A glycerolate dimethacrylate at 66.66 wt.% and 2-hydroxyethyl methacrylate at 33.33 wt.% were mixed to prepare the adhesive resins. Camphorquinone and ethyl 4-dimethylaminobenzoate at 1 mol% were added as photo- and co-initiators. Butylated hydroxytoluene was incorporated at 0.01 wt.% as polymerization inhibitor. All reagents for adhesives preparation were purchased from Sigma-Aldrich Chemical Co. (St. Louis, Missouri, USA). The reagents were hand-mixed for 5 min, following by sonicated during 180 s and hand-mixed for more 5 min. The base resin without fillers was used as a control group. The prepared nt-TiO 2 and nt-TiO 2 :TAT were incorporated in the adhesive resins at 2.5 or 5 wt.% separately, totalizing five experimental groups.
All samples, except for ultimate tensile strength and microtensile bond strength, were prepared using a polyvinylsiloxane mold measuring 1 mm thickness and 4 mm diameter. The light source used to photoactivate the adhesives was a light-emitting diode (Radii cal, SDI, Bayswater, Australia) with 1200 mW/cm 2 for 30 s on each side except for the polymerization kinetics test in which the samples were evaluated along 50 s of photoactivation.
2.5
Antibacterial activity evaluation
The antibacterial activity of the adhesives was evaluated for biofilm formation and planktonic bacteria against Streptococcus mutans (NCTC 10449, INCQS 00446). For the antibacterial activity test by direct contact inhibition, six samples from each group were attached to the cover of a 48-well plate that was submitted to hydrogen peroxide plasma sterilization [ , ]. Three samples from each group [ , , ] were used for 24 h assay and the other three samples for 48 h assay. Sucrose-riched brain-heart infusion (BHI) broth (900 μL, 1 wt.% sucrose, Sigma-Aldrich Chemical, St. Louis, Missouri, USA) was added in each well with 100 μL of an overnight culture suspension of Streptococcus mutans . The bacterium was subcultured as previously described [ ]. The set of cover with samples was placed on the 48-well plate with the samples’ surfaces exposed to the BHI containing the bacterium inoculum at 10 6 CFU/mL. The set was incubated for 37 °C for 24 h. The samples of 24 h assay were removed from the cover and vortexed in 1 mL of saline solution (0.9%) to be serially diluted until 10 −6 . The samples of 48 h assay were stored with fresh BHI broth containing sucrose and 10 6 CFU/mL of bacterium inoculum for 24 h. For both analyses, two drops with 25 μL from each dilution were plated on BHI agar on Petri dishes, and the plates were incubated at 37 °C for 48 h. The number of colonies was visually counted, and they were transformed in colony-forming units per milliliter (CFU/mL) using: (average number of colonies × dilution factor)/volume of the culture plate. These procedures were repeated with the samples from the 48 h assay. To analyze the viability of the planktonic bacteria in the BHI broth in contact with the polymerized samples, 100 μL from the BHI containing Streptococcus mutans were serially diluted in saline solutions until 10 −6 . Two drops of 25 μL from each dilution were plated on BHI agar on Petri dishes, and the plates were incubated for 48 h at 37 °C. The colonies were counted and transformed into CFU/mL as cited above. These procedures were performed for the 24 and 48 h assays.
2.6
Cytotoxicity
Sulforhodamine B (SRB) assay was performed against human pulp cells to analyze the possible cytotoxic effect of the formulated adhesives. Human pulp cells were collected from a third molar extracted after approval of the Research Ethics Committee of this local institution (nº 1.739.340). Eluates were prepared using three samples from each group [ , , ], which were sterilized with hydrogen peroxide plasma. Each sample was immersed in 1 mL of Dulbecco’s Modified Eagle’s Medium (DMEM) for 24 h to prepare the eluates. On this same day, 100 μL of DMEM containing the pulp cells (concentration of 5 × 10 3 ) were seeded in triplicate on a 96-well plate. After 24 h, 100 μL of eluate from each sample was inoculated in the wells containing pulp cells. A group of cells was treated with DMEM without eluates to be used as a negative control for this test. The 96-well plate was incubated at 37 °C for 72 h.
After this period, the pulp cells were treated with 50 μL of trichloroacetic acid solution at 50 vol.%, and they were incubated at 4 °C for 1 h and washed six times with running water. The plates were dried at room temperature. SRB (50 μL, Sigma-Aldrich, St. Louis, USA) at 0.4 vol.% was added in each well, and the plates were incubated for 30 min at room temperature. The plates were washed four times with acetic acid solution at 1 vol.%, and they were kept at room temperature to dry again. Trizma solution at 10 mM (100 μL) was added in each well to solubilize the dye, and the plates were incubated for 1 h at room temperature. The absorbance of the solution in each well was analyzed at a wavelength of 560 nm, and the cell viability was normalized against the cell viability of untreated cells (negative control). The results were expressed as a percentage of viability (100 % = viability of negative control).
2.7
Polymerization kinetics and degree of conversion
The polymerization kinetics and the degree of conversion were evaluated via Fourier Transform Infrared Spectroscopy (FTIR, Bruker Optics, Ettinger, Germany) with a spectrometer equipped with an ATR device. The spectrum of the background was run, and three uncured samples from each group [ , , ] were inserted in the molds on the ATR device. By subtracting the saved background spectrum, the polymerization kinetics was carried out for 50 s applying the tip of the light-curing unit device 1 mm distant from the top of each sample. Data processing was carried out with Opus 6.5 software (Bruker Optics, Ettlingen, Germany), from 800 to 4000 cm −1 , with 2 scans per second, the velocity of 160 kHz, and resolution of 4 cm −1 . The degree of conversion was calculated according to a previous study [ ] at 30 s, and the graphs of the degree of conversion versus time and polymerization rate (Rp) versus time were plotted using SigmaPlot software (12.0 version, Systat Software, San Jose, CA, USA).
2.8
Knoop hardness and softening in solvent
To analyze the Knoop hardness and softening in solvent of the formulated adhesives, three polymerized samples from each group [ , ] were embedded in self-curing acrylic resin. The samples were polished (Model 3v, Arotec, Cotia, SP, Brazil) with a sequence of silicon carbide sandpapers (600-grit, 1200-grit, and 2000-grit) under running water followed by the use of a felt disc saturated with alumina suspension (Alumina, 0.5 μm, Arotec, Cotia, SP, Brazil). The measurement of Knoop hardness was performed using a digital tester (HMV 2, Shimadzu, Tokyo, Japan). Five indentations with 10 g/5 s were made in each sample to access the initial (KHN1) and final (KHN2) Knoop hardness after immersion in an ethanolic solution (70%) for 2 h. The difference between KHN1 and KHN2 was expressed as percentages (ΔKHN%).
2.9
Ultimate tensile strength (UTS)
The mechanical property of the formulated adhesives was evaluated via UTS assay using ten samples from each group [ ]. The samples were prepared using a metallic mold with an hourglass shape (8 mm long, 2 mm wide, 1 mm thick, and a cross-sectional area of 1 mm²). After 24 h, the constriction area of each sample was measured using a digital caliper (Mitutoyo, Kawasaki, Kanagawa, Japan), and they were fixed in jigs with a cyanoacrylate resin. The samples were loaded under tension in a universal testing machine (EZ-SX Series, Shimadzu, Kyoto, Japan) at 1 mm/min. The values were acquired in newton (N), and they were expressed in megapascal (MPa).
2.10
Microtensile bond strength (μ-TBS)
The immediate μ-TBS was evaluated using one hundred bovine mandibular incisors (20 per group [ ]), who had their buccal surface removed and the dentin surface exposed. A 600-grit silicon carbide sandpaper was used with water for 30 s per tooth to create a simulated smear layer on the dentine surface. The dentine surface was etched with 37 % phosphoric acid for 15 s, washed for 30 s and gently dried with filter paper. A commercial primer (Primer Scotch Bond MultiPurpose; 3 M ESPE) was actively applied for 20 s, and the solvent was evaporated. The experimental adhesive resins were applied and photoactivated for 20 s. A composite build-up (Filtek Z350 XT, 3M ESPE, St Paul, USA) was performed in two increments of 2 mm each one, and they were light-cured for 40 s according to manufacturer instructions. The teeth were stored in distilled water at 37 °C for 24 h and sectioned into four to six sticks with 0.7 mm versus 0.7 mm in the bonding area. The bonding area of each stick was measured, and they were fixed in jigs with cyanoacrylate resin and subjected to tensile strength in a universal testing machine (EZ-SX Series, Shimadzu, Kyoto, Japan) at 1 mm/min until failure.
For the long-term analysis, one hundred bovine mandibular incisors (20 per group) were treated as cited above, except for the time of storage of the sticks. The sticks were stored in distilled water for one year in an incubator at 37 °C. After this period, their bonding area was measured, and they were tested under tensile strength. The pattern of fracture after the μ-TBS assays was analyzed and classified as adhesive (A), cohesive in dentin (CD), cohesive in composite resin (CR), or mixed (M). The results were expressed in percentages.
2.11
Statistical analysis
Data were analyzed with SigmaPlot software (version 12.0, Systat Software, San Jose, CA, USA). The distribution of data was evaluated via the Shapiro–Wilk test. Two-way ANOVA and Tukey post-hoc test were used to compare the groups for the antibacterial activity assay and μ-TBS. One-way ANOVA and Tukey post-hoc tests were used to compare groups for the degree of conversion, KHN1, ΔKHN%, UTS, and cytotoxicity. Paired t -test was used to compare the results from KHN1 and KHN2 within each group. All tests were performed at a significance level of 0.05.
3
Results
The nt-TiO 2 applied as filler for the adhesives was synthesized according to a previously reported method [ ], and the nt-TiO 2 :TAT was characterized via TEM, showing mean size of 45.8 (± 7.4) nm, which is similar to the size already described in the literature [ ]. The nt-TiO 2 :TAT presented a typical morphology of nanotubes ( Fig. 1 B–C). The particles were arranged in aggregates, and they presented clearer areas suggestive of the lumen presence.
The nt-TiO 2 , TAT, and nt-TiO 2 :TAT were evaluated via FTIR, UV–vis, and micro-Raman spectroscopies. Fig. 2 shows the spectra for each material from these analyses. In the nt-TiO 2 spectrum via FTIR, the absorption band at 3300 cm –1 is related to the asymmetric and symmetric stretching vibrations of O H group, whereas the band around 1637 cm −1 is the HOH bending vibration of the coordinated water [ ]. The broad band below 1000 cm −1 is indicative of Ti OT i anatase and rutile phases [ ]. In TAT FTIR spectrum, the band at 1648 cm –1 was attributed to the CO stretching; the peaks at 1611 and 1595 cm –1 were attributed to the C N vibrations of triazine ring and the band at 1430 cm –1 was due to CHC H 2 vibration of acrylamide [ ].

In the UV–vis, the absorbance band of nt-TiO 2 was larger than the band of TAT. The UV–vis provided the absorbance values to calculate the band gap of nt-TiO 2 and nt-TiO 2 :TAT. The nt-TiO 2 showed band gap of 3.4 eV and the nt-TiO 2 :TAT indicated a value of 3.5 eV.
In micro-Raman, the peaks at 639 cm −1 [ , ] and 514 cm −1 [ ] are related to the anatase phase, while the peak at 453 cm −1 is related to rutile phase [ , ]. A previous study showed that the nanotubes present 52:48 (%) anatase:rutile phases determined by X-ray diffraction [ ]. TAT showed its classical peak at 835 cm −1 related to C 2 N 3 ring [ ]. In the spectrum of nt-TiO 2 :TAT, it is possible to observe the peaks related to TAT and nt-TiO 2 , although the peaks from 450 cm −1 to 640 cm −1 of nt-TiO 2 are not totally distinguished in the spectrum.
Fig. 3 displays the results of the antibacterial activity ( Fig. 3 A) and cytotoxicity ( Fig. 3 B) analyses. Fig. 3 A shows that the groups with the triazine-methacrylate monomer had lower bacterial viability in biofilm in comparison to the control group and groups containing nt-TiO 2 (p < 0.05). The lowest bacterial viability in biofilm was observed for 5 wt.% of nt-TiO 2 :TAT (3.64 ± 0.08 log CFU/mL) followed by the group with 2.5 wt.% of nt-TiO 2 :TAT (4.10 ± 0.08 log CFU/mL). The values did not statistically differ from 24 h to 48 h (p > 0.05). There was no difference among groups against the planktonic bacteria in contact with the polymerized samples (p > 0.05). The results of cytotoxicity analysis against pulp cells ( Fig. 3 B) showed that the addition of 5 wt.% of nt-TiO 2 (97.84 ± 2.20 %) and 2.5 wt.% of nt-TiO 2 :TAT (93.83 ± 3.97 %) presented significantly higher values when compared to the control group (82.06 ± 3.45 %) (p < 0.05). The lowest value was observed for the control group, without statistical difference for 2.5 wt.% of nt-TiO 2 and 5 wt.% of nt-TiO 2 :TAT (p > 0.05).
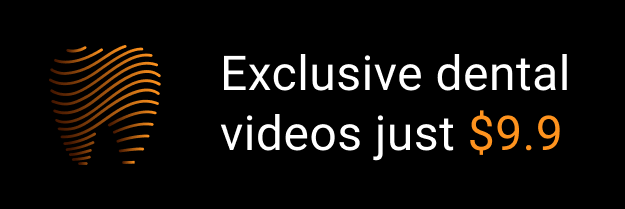