Highlights
- •
Optical Coherence Tomography shows real-time 3D internal defects in composites.
- •
Tested light-cured bulk-filled materials mainly showed inner voids and cracks.
- •
New flowable bulk-fill material achieved sound restoration with high irradiance.
- •
Flowable bulk-fill composites showed lower defect and better degree of conversion.
- •
Light settings affected defects formation but not degree of conversion.
Abstract
Objective
The study used optical coherence tomography (OCT) and micro Raman microscopy (mRM) to investigate internal defect (ID) and degree of conversion (DC) of two bulk-fill composite systems with high-irradiance or conventional light polymerization settings.
Methods
The ID and DC of the new bulk-fill composites; Tetric PowerFill (PwrPst) and Tetric PowerFlow (PwrFlw) were compared with the predecessor bulk-fill composites; Tetric EvoCeram Bulkfill (EvoPst) and Tetric EvoFlow Bulkfill (EvoFlw), using LED light curing unit in two settings; normal (LED-Nrm: 1000 mW/cm 2 , 10-s) or PowerCure (LED-Pwr: 3000 mW/cm 2 , 3-s). ID formation was observed in bonded cylindrical composite cavities (4-mm depth, 3-mm diameter) using Yoshida Dental OCT. From the vertically projected 2D image, ID area percentage (ID-A%) was calculated. DC at the top and bottom and bottom/top DC ratio (DC-R%) of 4-mm thick discs was calculated using mRM. All data were statistically analyzed at significance level of α = 0.05.
Results
Flowable composites in LED-Pwr showed smaller ID frequencies than other groups (PwrFlw: 0/8, EvoFlw: 2/8) (p < 0.05). Composite type significantly affected ID-A%, DC and DC-R% (p < 0.001), while light settings significantly affected ID-A% but not DC and DC-R% (p > 0.05). In both light settings, EvoPst showed the lowest DC-R% (<80%).
Significance
Internal defect and degree of conversion of bulk-fill composites were affected by their composition and light settings. There was a tendency for less defects and better polymerization for the flowable bulk-fills. The new flowable bulk-fill composite with 3-seconds high irradiance light curing showed no defect formation.
1
Introduction
Resin composites have become reliable materials for direct restorations, thanks to the development of the mechanical properties and adhesive technology [ ]. In order to meet the demands for application in various clinical situations particularly extensive restorations, incremental layering techniques were introduced [ ]. The rationale behind the technique was to guarantee the penetration of the curing light deeply enough to initiate and complete curing, besides the minimization of the shrinkage and shrinkage-induced stress associated with the polymerization [ ]. The time consuming nature of the placement and light irradiation in multiple increments along with the risks of void formation and oral fluid contamination [ ] have promoted the development of bulk-fill resin composites, which have been commercially introduced for placement as a single increment up to 4- or 5-mm depth [ ].
Polymerization shrinkage of methacrylate-based dental resin composites is unavoidable, due to the fact that monomer molecules are converted into a polymer network and, therefore, exchanging van der Waals spaces in covalent bond spaces [ ]. Contraction stress is the result of polymerization shrinkage taking place under confinement caused by bonding to cavity walls [ ]. The stress magnitude is affected by the volume of each increment and cavity configuration (C-factor) [ ], which are relatively larger in bulk-fill materials applications. Therefore, the stress control has been one of the main subjects in the material development. Various approaches have been employed in the formulation of bulk-fill composites to reduce the stress, adjust the stress generation kinetics and improve depth of cure. Those include changes in filler content and shape [ ], modified monomer molecular weight and structure [ ], addition of stress relievers and polymerization modulators [ , ], increase of polymerization inhibitors [ ], new combinations of photoinitiators [ ], enhanced material translucency and dual-cured polymerization mechanisms [ ].
In addition to the resin composite material deveropments, the dental light curing units (LCUs) have been evolving in the paradigm shift. Light emitting diodes (LEDs) have intensively been used as light source for LCUs due to the advantages over quartz–tungsten–halogen units (QHTs) in terms of lower heat emission, maintenance cost, and the higher durability [ ]. Compared to the wide range wavelength output of QHTs, LEDs have a narrower maximum peak of light emission [ ]. In order to achieve the maximum polymerization efficiency of each composite, various LED-LCUs have been developed with adjustment the emission peaks and combination of different LEDs that match the absorption spectrum of the targeted photoinitiators [ ]. However, these technological developments have set some restrictions on the selection of LCUs to match the photo-polymerization requirements of the composites [ ].
Manufacturers have introduced high irradiance LED-LCUs, anticipating an improved polymerization efficiency and shortened clinical procedure time [ ]. Recently, a bulk-fill composite system including high and low viscosity light-cured bulk-fill composites and a high irradiance light curing unit has been developed (3 s PowerCure, Ivoclar Vivadent AG, Schaan, Liechtenstein). The system is compositionally mainly similar to its predecessors, but features a 3-seconds curing setting at 3000 mW/cm 2 .
According to the exposure reciprocity law, the total radiant exposure (J/cm 2 ) is the product of irradiance (W/cm 2 ) and exposure time (s). However, studies have reported that the degree of conversion (DC) and the kinetics of the composite polymerization depended on the many parameters and did not necessarily conform to this law and total radiant exposure [ , ]. Furthermore, the high irradiance might be associated with negative effects on stress generation [ , ]. In spite of these concerns, clinical evaluations with up to 10 years follow-up suggested that bulk-fill composites were comparable in performance with incremental composite restorations [ ]. The adverse events of bulk-fill composite restorations were mainly associated with physical defects, loss of marginal integrity and tooth/restoration fractures [ , ]. Given the importance of the material integrity, a meaningful investigation of a newly introduced bulk-fill materials should consider internal defect (ID) formation [ ].
Optical coherence tomography (OCT) has been introduced for non-invasive real-time observation of ID formation and 3D quantification in resin composites. The infrared beam of high-speed swept-source OCT is far in wavelength from the absorption spectrums of any photo-initiators. In addition, fast digital to analogue (DAC) technology and powerful image processing [ , ] have facilitated 3D real-time observation of the polymerization phenomenon.
The objective of this study was to observe and quantify ID formation during light polymerization of the new fast-curing bulk-fill composite by OCT followed by DC analysis using micro Raman microscophy. The null hypothesis was that there was no significant difference in ID and DC among the new and the predecessor bulk-fill materials and in combination with different light curing settings.
2
Materials and methods
2.1
Specimen preparation
Procedures of specimen preparation and OCT real-time monitoring followed a previous study [ ]. Cylindrical cavities (3 mm in diameter, 4 mm in depth, C-factor = 4.98) were prepared in resin composite blocks using a diamond bur (SF101CR, Shofu, Kyoto, Japan) with a high-speed handpiece under water coolant. A silicone impression of the preparation was made (EXA’lence extra light body; GC America, IL, USA), form which 64 standardized molds were fabricated with a light-curing flowable resin composite (Estelite Flow Quick shade A2; Tokuyama Dental, Tokyo, Japan). The flowable resin composite was inserted and polymerized in 4 increments with 40 s using a halogen light curing unit with 500 mW/cm 2 light irradiance (Coltolux 75; Coltene/Whaledent Inc., Cuyahoga Falls, OH, USA) for each increment. Each resulting mold was stored in 37 °C for 1 week to complete polymerization. In order to provide sufficient bonding, the inner surfaces of the molds were sandblasted with 50 μm alumina particles (MicroEtcher II; Danville Materials, Carlsbad, CA, USA) at 0.4 MPa for 15 s, followed by ultrasonic cleaning for 10 min (Model US 102; SND, Nagano, Japan), phosphoric acid cleaning (K-etchant gel; Kuraray Noritake Dental, Tokyo, Japan), silanization (Clearfil Ceramic Primer Plus; Kuraray Noritake Dental, Tokyo, Japan), and adhesive (Adhese Universal; Ivoclar Vivadent AG, Schaan, Liechtenstein). All surface conditioning steps were performed in accordance with the manufacturer’s instructions as listed in Table 1 .
Tested composites | |||
---|---|---|---|
Material/shade (code/lot no). manufacturer | Recommended light activation time and irradiance | Viscosity | Composition (filler wt% & vol%) |
Tetric EvoCeram Bulk fill /IVA ( EvoPst /W33026) Ivoclar Vivadent AG, Schaan, Liechtenstein | 20 s (≥500 mW/cm 2 ), 10 s (≥1000 mW/cm 2 ) | High | Monomers; Bis-GMA, Bis-EMA, UDMA Fillers; Barium aluminium silicate glass, Ytterbium, Trifluoride, Prepolymer, Mixed Oxide (77 wt%, 55 vol%) |
Tetric EvoFlow Bulk fill /IVA ( EvoFlw /V30889) Ivoclar Vivadent AG, Schaan, Liechtenstein | 20 s (≥500 mW/cm 2 ), 10 s (≥1000 mW/cm 2 ) | Low | Monomers; Bis-GMA, Bis-EMA, UDMA Fillers; Barium aluminium silicate glass, Ytterbium, Trifluoride, Prepolymer, Mixed Oxide (68.2 wt%, 46.4 vol%) |
Tetric PowerFill /IVA ( PwrPst /W11515) Ivoclar Vivadent AG, Schaan, Liechtenstein | 20 s (500–900 mW/cm 2 ), 10 s (900–1400 mW/cm 2 ) 5 s (1800–2200 mW/cm 2 ), 3 s (2700–3300 mW/cm 2 ) | High | Monomers; Bis-GMA, Bis-EMA, UDMA, Bis-PMA, DCP, D3MA. Fillers; Barium glass, Ytterbium, Trifluoride, Copolymer, Mixed Oxide (SiO 2 /ZrO 2 ) (79 wt%, 53–54 vol%) |
Tetric PowerFlow /IVA ( PwrFlw /WM1175) Ivoclar Vivadent AG, Schaan, Liechtenstein | 20 s (500–900 mW/cm 2 ), 10 s (900–1400 mW/cm 2 ) 5 s (1800–2200 mW/cm 2 ), 3 s (2700–3300 mW/cm 2 ) | Low | Monomers; Bis-GMA, Bis-EMA, UDMA, CMP-1E, DCP, D3MA. Fillers; Barium glass, Ytterbium, Trifluoride, Copolymer, Mixed Oxide (SiO 2 /ZrO 2 ) (71 wt%, 46–47 vol%) |
Specimen preparation and surface treatment materials | |||
---|---|---|---|
Materials lot no. | Manufacturer | Composition | Application |
Estelite Flow Quick shade A2 JO651 | Tokuyama Dental, Tokyo, Japan | Monomers; Bis-MPEPP, TEGDMA, UDMA, Fillers; Silica-zirconia filler, silica-titania fillers (71 wt%, 53 vol%) | Dispense in layers up to 2 mm in thickness; light cure for 20 s. |
K-etchant gel 3D0042 | Kuraray Noritake Dental, Tokyo, Japan | 35–45% phosphoric acid, colloidal silica, water | Apply onto the adherent surface and leave for 5 s; Thoroughly wash and dry. |
Clearfil Ceramic Primer Plus A50030 | Kuraray Noritake Dental, Tokyo, Japan | Methacryloxypropil trimethoxy silane, MDP, Ethanol | Apply to the adherent surface; dry the entire adherent surface sufficiently using mild, oil-free air blow. |
Adhese Universal W83530 | Ivoclar Vivadent AG, Schaan, Liechtenstein | MDP, Bis-GMA, HEMA, CQ, MCAP, D3MA, ethanol, water, highly dispersed silicon dioxide | Apply onto the adherent surface and mild air blow; Light cure for 10 s. |
Bis-EMA, bisphenol-A polyethylene glycol diether dimethacrylate; Bis-GMA, bisphenol-A diglycidyl ether dimethacrylate; UDMA, urethane dimethacrylate; Bis-PMA, Propoxylated bisphenol A-dimethacrylate; DCP, tricyclodecane dimethanol dimethacrylate; D3MA, decandiol dimethacrylate; CMP-1E, p-cumylphenoxyethylene glycol methacrylate; Bis-MPEPP, bisphenol A polyethoxy methacrylate; TEGDMA, triethylene glycol dimethacrylate; MDP, 10-methacryloyloxydecyl dihydrogen phosphate; CQ, camphorquinone; HEMA, 2-hydroxyethyl methacrylate; MCAP, Methacrylated carboxylic acid polymer |
The light curing unit | ||
---|---|---|
Equipment, manufacturer | Light source and wavelength | Curing setting (code), irradiance & time (total exposure) |
Bluephase PowerCure Experimental #8 Ivoclar Vivadent AG, Schaan, Liechtenstein | 3 blue and 1 violet light LEDs Spectral peaks; 410 nm and 460 nm (>385 nm, <515 nm) | Normal setting ( LED-Nrm ); 1200 mW/cm 2 , 10 s (=12 J/cm 2 ) Power setting ( LED-Pwr ); 3400 mW/cm 2 , 3 s (=10.2 J/cm 2 ) |
Four types of light-cured resin composites (two high-viscous and two low-viscous resin composites) recommended for bulk placement in 4 mm depth were evaluated ( Table 1 ); two new bulk-fill composites; Tetric PowerFill (PwrPst, paste-type) and Tetric PowerFlow (PwrFlw, flowable) and their two predecessor bulk-fill composites; Tetric EvoCeram Bulkfill (EvoPst, paste-type) and Tetric EvoFlow Bulkfill (EvoFlw, flowable) (Ivoclar Vivadent AG, Schaan, Liechtenstein).
The resin composites were placed in the mold in bulk, and the free surface was covered by a plastic matrix (Hawe Transparent Striproll, Kerr, Orange, CA, USA) to flatten the surface and isolation without disturbing the light delivery. High viscous composites (EvoPst and PwrPst) were placed using a hand instrument as a single increment. Low viscous composites (EvoFlw and PwrFlw) were directly dispensed from the syringe using the provided tips.
The tested composites were polymerized by a LED light curing unit (Bluephase PowerCure premarket prototype test sample #8, Ivoclar Vivadent AG, Schaan, Liechtenstein) with two light settings similar in nominal total exposure; normal setting (LED-Nrm; 1000 mW/cm 2 , 10 s) and power setting (LED-Pwr; 3000 mW/cm 2 , 3 s). The radiant exitance of each mode was confirmed prior to the experiment using a handheld radiometer (Bluephase Meter II, Ivoclar Vivadent AG, Schaan, Liechtenstein). The values recorded from 3 measurements were consistently 1200 mW/cm 2 and 3400 mW/cm 2 , respectively. During photo polymerization, the LCU guide tip was set perpendicularly as close as possible over the plastic matrix on the top surface of the resin composite.
2.2
Optical observation of internal defect formation and quantitative 3D analysis
A prototype OCT system (Yoshida Dental OCT, Yoshida Mfg., Tokyo, Japan) with 12.13 mW output power at sample, a center wavelength was 1310 nm (bandwidth 140 nm) and 50-kHz sweep rate was used for evaluation of the internal defect formation in the placements of composites. The optical resolution is 40 μm transversally and 11 μm axially in air (7–8 μm in tissues with a refractive index around 1.5) [ ]. During polymerization of the resin composites, the OCT probe was placed beneath the mold specimen so that formation of the internal defect at deeper regions of the restoration adjacent to the cavity floor could be imaged through the 0.5-mm thick mold. The XZ cross-sectional views (B-scans) at the center of the mold and en-face views were recorded continuously in real time as a windows media video file at a resolution of 800 × 600 pixels and 20 frames per second. 3D scans were obtained immediately after light-curing at 400 × 400 × 1024 pixels over an XYZ volume of 6 × 6 × 8 mm 3 . Immediately after the scanning, OCT was applied from top of the cavities so that the integrity at the middle-top regions of the placements of all specimens was confirmed.
ID frequency was recorded as the specimens with any defect formation during polymerization in each group. Amira software (version 5.5.0, FEI Visualization Sciences Group, Oregon, USA) was then used for quantitative analysis of OCT data. For ID quantification, a volume of interest (VOI) of 3 × 3 × 1 mm 3 , covering the whole cavity floor area was selected on each scan. A binary image was created by averaging XY slices over 1000-μm thick region at the floor using the maximum intensity projection (MIP) in the VOI, according to a previously established method [ , ]. Internal defect area percentage (ID-A%) values were calculated considering the area proportion of the binarized pixel clusters over total floor area in each MIP image.
2.3
Degree of conversion (DC)
Discs from each the 4 experimental composites were placed and polymerized using each of the two light curing settings as described earlier in a silicone mold (3 mm in diameter, 5 mm in height). After 24 h storage in deionized water at 37 °C, the discs were axially sectioned at the center with low-speed diamond saw (Isomet, Buehler, Lake Bluff, IL, USA) in a dark room. Raman microscopy (LabRAM HR Evolution, Horiba, Kyoto, Japan) with power output of 5 mW was used to collect 633 nm wavelength laser spectrum along a Z-axis line at the center of the cross-section at 500 μm intervals (n = 3). Unpolymerized resin composite specimens (3 mm in diameter, 1 mm in height) were also measured under the same Raman settings at the center as a reference. From the obtained spectrums, the degree of conversion (DC) of the polymerized specimen was calculated using the aliphatic CC peak at 1640 cm −1 as an immutable reference and the aromatic CC peak at 1610 cm −1 as a reaction peak as follows:
DC=1-aliphaticC=CaromaticC=CpolymerisedaliphaticC=CaromaticC=Cunpolymerised
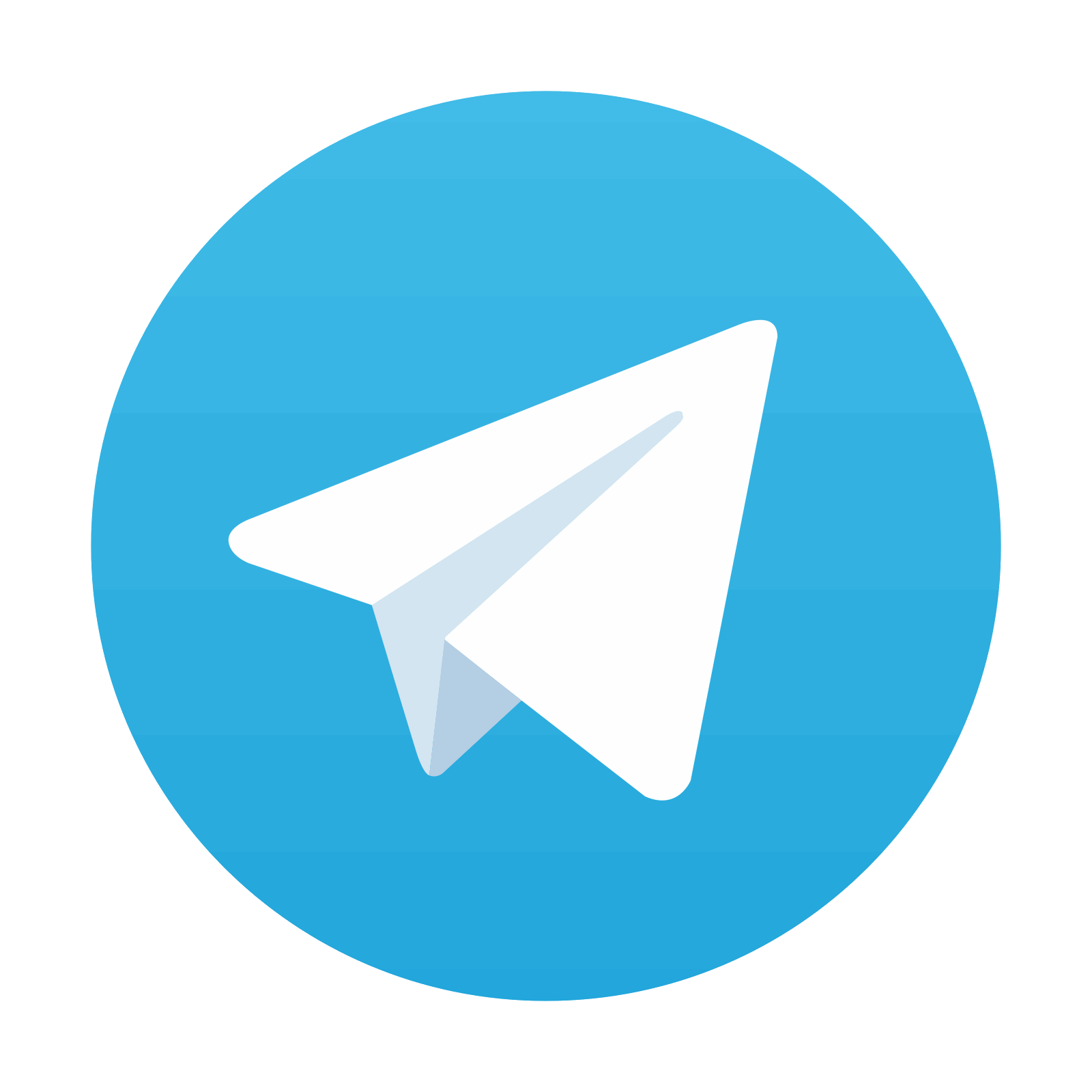
Stay updated, free dental videos. Join our Telegram channel

VIDEdental - Online dental courses
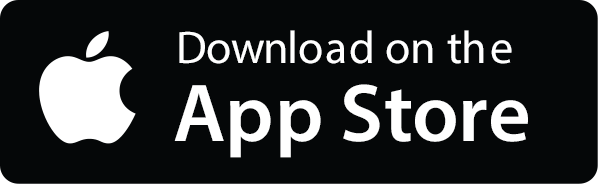
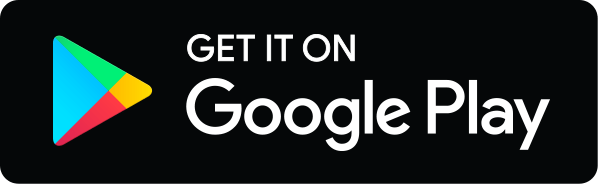
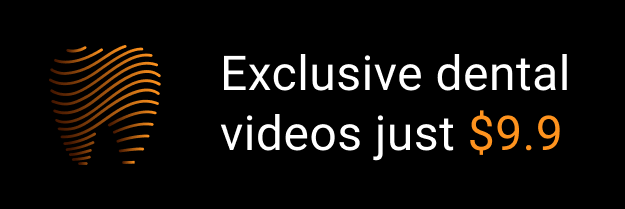