Highlights
- •
Development of a dynamic mechanical analysis method to assess bulk viscoelastic behavior of dentin
- •
Protein destabilization accelerates H-bond breakage in biomodified dentin matrix
- •
Key PAC structural features achieve stable biomodification via covalent bonds
Abstract
Objectives
To develop a protocol for assessment of the bulk viscoelastic behavior of dentin extracellular matrix (ECM), and to assess relationships between induced collagen cross-linking and viscoelasticity of the dentin ECM.
Methods
Dentin ECM was treated with agents to induce exogenous collagen cross-linking: proanthocyanidins (PACs) from Vitis vinifera – VVe, PACs from Pinus massoniana – PMe, glutaraldehyde – (GA), or kept untreated (control). A dynamic mechanical strain sweep method was carried out in a 3-point bending submersion clamp at treatment; after protein destabilization with 4 M urea and after 7-day, 6-month, and 12-month incubation in simulated body fluid. Tan δ, storage (E’), loss (E”), and complex moduli (E*) were calculated and data were statistically analyzed using two-way ANOVA and post-hoc tests (α = 0.05). Chemical analysis of dentin ECM before and after protein destabilization was assessed with ATR-FTIR spectroscopy.
Results
Significant interactions between study factors (treatment vs . time points, p < 0.001) were found for all viscoelastic parameters. Despite a significant decrease in all moduli after destabilization, PAC-treated dentin remained statistically higher than control (p < 0.001), indicating permanent mechanical enhancement after biomodification. Covalently crosslinked, GA-treated dentin was unaffected by destabilization (p = 0.873) and showed the lowest damping capacity (tan δ) at all time points (p < 0.001). After 12 months, the damping capacity of PMe and VVe groups decreased significantly. Changes in all amide IR resonances revealed a partial chemical reversal of PAC-mediated biomodification.
Significance
Viscoelastic measurements and IR spectroscopy aid in elucidating the role of inter-molecular collagen cross-linking in the mechanical behavior of dentin ECM.
1
Introduction
The viscoelasticity of dentin is attributed to the extracellular matrix (ECM) enabling the combined behavior of an elastic solid and a viscous liquid [ ]. Under uniaxial static forces, viscoelastic tissues commonly exhibit lack of linearity in the stress-strain curve [ , ]. In contrast to static methods, dynamic mechanical analysis (DMA) uses harmonic vibrations simulating the cyclic/dynamic loading of the dentin and, thus, enhancing mechanical characterization of viscoelastic materials.
Previous efforts showed that, at the nano-length scale, the viscoelasticity of the dentin (nanoDMA, small angle X-ray scattering, AFM) is attributed to the sliding and unfolding of collagen molecules and fibrils [ ], while at a higher hierarchical level, the viscoelasticity is associated with the crosslinking and density of the collagen fibrils [ ]. Consequently, dentin’s mechanical behavior results from its complex hierarchical composition, and the analysis of its bulk viscoelastic properties leads to a better mechanical understanding. The storage modulus (E’) represents the elastic properties of a material (capacity to store energy, solid-like response) while the loss modulus (E”) refers to the viscous properties of a material (capacity to lose energy, fluid-like response). The ratio between both moduli (tan δ), also called damping capacity, describes the ability of the material to dissipate energy and is considered an important functional feature [ , ].
The determination of collagen crosslinking effects on the bulk viscoelasticity of dentin is possible through the analysis of the viscoelastic properties. Collagen crosslinking is a post-translational modification of proteins that directly impacts the viscoelastic properties of the ECM [ , , ]. Plant-derived proanthocyanidins (PACs) and synthetic glutaraldehyde (GA) can induce non-enzymatic crosslinking that biomodifies the dentin matrix and promote the enhancement of their mechanical and biochemical properties [ , ]. Such dentin biomodification takes place at different hierarchical levels, and these chemical interactions are the basis for the biological stability, tensile strength, and viscoelasticity of the resulting dentin matrix [ , , , ].
A two-fold aim was pursued in this study: (1) to develop a method for the investigation of the bulk dynamic mechanical behavior of dentin matrices; and (2) to determine the mechanical and chemical stability of dentin ECM treated with biomodification agents using an accelerated method of protein destabilization. The null hypothesis tested was that the chemical and viscoelastic properties of the bulk dentin matrix, induced by different collagen cross-linkers, would not be affected by an accelerated protein destabilization protocol.
2
Methods
2.1
Preparation of dentin extracellular matrix and biomodification strategies
Mid-coronal dentin specimens of 1.5 × 7 × 0.5 mm (width × length × thickness) were prepared from extracted human sound molars (IRB no. 2018-0346) and demineralized in 10% phosphoric acid (Ricca Chemical Company, Arlington, TX, USA) for 5 h. A dimple created with a diamond bur (835.31.014 F G, Brasseler USA Dental, Savannah, GA, USA) in one edge of the specimen allowed constant specimen positioning throughout the entire experiment.
Demineralized specimens were assigned into groups of synthetic and natural dentin biomodification agents (n = 5). Biomodification strategies included 0.65% w/v of enriched extract from Vitis vinifera (VVe) [ , ] or Pinus massoniana (PMe) [ ], in 20 mM HEPES; and 5% v/v glutaraldehyde – GA (Lot 894368, Fisher Scientific, Fair Lawn, NJ, USA) in distilled water (DW) [ ]. A control group was exposed to HEPES buffer only. Specimens were immersed in 100 μL of solution (pH 7) for 1 h at room temperature and rinsed with DW. To leach any loosely bound biomodification agent, specimens were kept for 24 h in DW prior to testing.
2.2
Chemical protein destabilization
The protein destabilization protocol consisted of the incubation of specimens in 4 M urea (Lot. R25482, MP Biomedicals, Solon, OH, USA) diluted in DW (pH 6.8) for 24 h at 37 °C [ , ]. Then, specimens were rinsed and stored in simulated body fluid (SBF: 50 mM HEPES, 5 mM CaCl 2 ⋅2 H 2 O, 0.001 mM ZnCl 2 , 150 mM NaCl, and 3 mM NaN 3 , pH 7.4) [ ] for 12 months. SBF was changed every two weeks.
2.3
Dynamic mechanical analysis
A strain sweep was conducted to assess viscoelastic properties of the dentin ECM using a dynamic mechanical analyzer (Q800 DMA, TA Instruments, New Castle, DE, USA). A 3-point bending submersion clamp with a drive shaft that oscillates vertically under controlled stress forces was used with a 5-mm span ( Fig. 1 ). The measurements correspond to the response of a viscoelastic material as it is deformed over a range of strain (deformation amplitude) monitored at a constant frequency and temperature. The viscoelastic mechanical components were calculated (modulus precision: ± 1%) using instrument signals (force, displacement, and stiffness) and recorded specimen dimensions (thickness and width).

Based on pilot studies, the adopted test parameters were as follows: preload force of 0.01 N, amplitude sweep gradually ascending from 1 to 100 μm (in 10 steps), frequency of 1 Hz at room temperature. All properties were calculated within the range of linear viscoelasticity, where the modulus is independent of the strain. The storage modulus or elastic component is calculated as E’= (τ 0 /ε 0 ) cos δ, the loss modulus or viscous component as E”= (τ 0 /ε 0 ) sin δ, and the complex modulus as E* = (E’ 2 + E” 2 ) 0.5 , where τ 0 is the peak stress, ε 0 is the peak strain, and δ is the phase lag between stress and strain ( Fig. 1 ) [ ]. The damping capacity (tan δ) was calculated as the ratio of the loss modulus to the storage modulus (E”/E’) and reflects a fluid-like (viscous, Newtonian) behavior when value is high and a solid-like (elastic, Hookean) behavior when tan δ is low. Thus, a purely elastic material has a tan δ = zero.
All demineralized dentin specimens were pre-screened to include specimens with complex modulus in the range of 6−10 MPa baseline values. These specimens were then distributed into the experimental groups. DMA measurements were carried out at the following time points: biomodification (T1), protein destabilization (T2); and at 7-days (T3), 6-months (T4), and 12-months (T5) incubation in SBF.
2.4
Physicochemical analysis of the dentin extracellular matrix
The structural and biochemical variation of the dentin collagen before and after protein destabilization was assessed using ATR-FTIR spectroscopy (Nicolet 6700 and Smart iTR, Thermo Fisher Scientific, Waltham, MA, USA). Dentin specimens (2 × 6 × 0.5 mm, width × length × thickness, n = 3) were demineralized and treated as described in sections 2 .1 and 2.2. Absorbance spectra were collected in the range of 650 to 4000 cm −1 , using 128 acquired scans and a resolution of 4.0 cm −1 . The peak area of specific bands were determined following a two-point baseline correction, normalization, and band area integration using OMNIC Spectra Software (Thermo Fisher Scientific, USA). Variation in the secondary structure of the collagen triple helix was calculated by the ratio of the peak area of amide III (1240 cm −1 ) to the CH 2 scissoring (1450 cm −1 ) bands [ , ].
2.5
Statistical analysis
Levene’s intragroup variability test met the assumption of homogeneous distribution for the variable storage modulus (p = 0.071) but not for loss modulus (p = 0.002), complex modulus (p = 0.04) and tan δ (p < 0.001). Accordingly, data were analyzed using two-way ANOVA followed by Tukey or Games-Howell for multiple comparisons. Ratios showing structural variations of the dentin collagen were compared using one-way ANOVA and Tukey’s post hoc test. The level of significance for all tests was set at 5%.
3
Results
3.1
Dynamic mechanical analysis
Fig. 2 shows the range of linear viscoelasticity of the dentin ECM. Significant interactions between study factors (treatment groups vs . time points, p < 0.001) were found for E*, E’, E” and tan δ ( Fig. 3 ). While all treatments (dentin biomodification) significantly increased E’ and E” moduli, dentin ECM treated with PMe and VVe showed the highest values after biomodification (p < 0.001). The damping capacity (tan δ) among groups from highest to lowest was as follows: VVe > control = PMe > GA (p < 0.001).
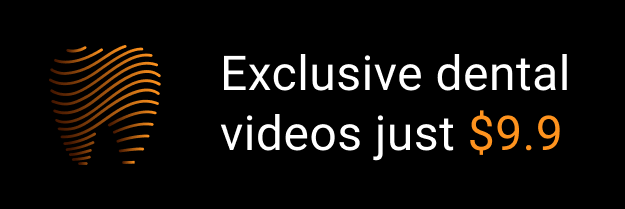