Fig. 9.1
Pictures of the biofilm model used in this study. (a) Custom-made stainless steel lid on which 24 clamps are fixed. Substrata glass cover slips or HA disks are shown. (b) Position of the substrata (HA disks) in the 24-well plate at the time of biofilm growth [25]
9.1.2.3 Open (Continuous Culture) System Microbial Models
Flow Cell Biofilm Model and Modified Robbins Device
Flow cell biofilm model and modified Robbins device [28] (MRD, Fig. 9.2) shared similar principles for the operation, whereby culture fluid is passed through a tube or cell and biofilms may be monitored microscopically (in flow cells) or formed on coupons (in some flat plate flow cells) or pegs (Robbins devices) [29]. Flow cells are commercially available devices with glass chambers that are particularly well suited for real-time nondestructive microscopic analyses of biofilms [14]. This model is not inexpensive, and the design can be versatile in the selection of material for the substratum [30]. They still have some disadvantages: Firstly, sterilization can be difficult because many of the common materials used for flow cell construction do not respond well to autoclaving, especially to repeated autoclaving. Secondly, peristaltic pumps can produce some pulsation in liquid delivery. Finally, in cases of high biomass within the flow cell [30], it is conceivable that a gradient in nutrients could be established over the length of the flow cell. As commonly used biofilm models, flow cells can be used for single-species biofilm or multispecies biofilm. The co-adhesion of Streptococcus gordonii with Streptococcus oralis was studied in a two-species in vitro oral biofilm flow cell system, in which green fluorescent protein was used as a species-specific marker [31]. In MRD, the tube can be plastic or metal, into which pegs can be inserted so that when in place, the end of the peg forms part of the wall of the tube [13]. In a typical experiment, the MRD is filled with a suspension of microorganisms and is flipped over to improve the adhesion of the planktonic cells to the disks [14]. After the adhesion phase, the pump is started to allow a continuous flow of the growth medium and biofilm development on the disks. The advantage of this system is that the biofilms can be sampled by removing plugs at any time, once they are replaced to maintain a closed system. This model was established as an appropriate biofilm model for susceptibility testing of oral microorganisms [32].
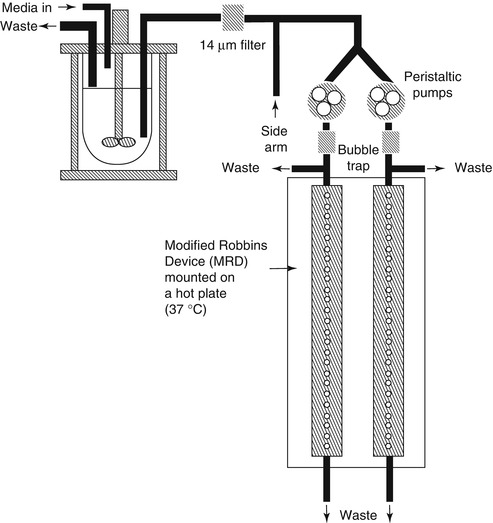
Fig. 9.2
Modified Robbins device [28]
Drip-Fed Biofilm Model
Among drip-fed biofilm models, drip flow biofilm reactors are a simple sort, in which biofilms are grown on angled slides continuously irrigated with small volumes of (inoculated) media, thereby providing a low-shear environment with dispersive mixing (Fig. 9.3) [14, 33, 34]. This system has been developed as an interproximal laboratory model to compare the potential effectiveness of powered brushing to remove biofilm plaque from interproximal spaces beyond the reach of bristles [35]. Different substrata can be used such as hydroxyapatite or teeth tissues. Care is required in experimental design and in sampling because medium flow over the surface of the slide may not be uniform and hence there may be significant aerial heterogeneity over the surface of the substratum [13]. In constant depth film fermenter (CDFF), biofilms are fed by the drip-wise addition of growth medium onto the turntable, and excess and/or spent medium flows downward through a waste outlet [13]. The biofilm developed on a surface is limited to a predetermined depth by mechanically removing excess biofilm, a situation mimicking the movement of the tongue over the teeth [14]. In this system, 200 or 300 μm is most commonly used for maintenance of dental plaques [13]. This system can develop single-species biofilm, defined consortium biofilm, or microcosm biofilm to evaluate antibacterial agent and explore the etiology of dental caries [36, 37].
Perfused Biofilm Fermenters
Perfused biofilm fermenters (PBF) are constructed such that nutrients are supplied by continuous perfusion of growth medium, which is pumped through the substratum (a permeable membrane) and hence through the biofilm [13]. The media flow can be accurately controlled, the growth rate of the biofilm can also be well modulated, and dynamic steady states can be achieved. The multiple Sorbarod device (MSD) is one kind of PBF which is proved to be an ideal system to grow oral biofilms by McBain and co-workers. It uses a simple two-piece stainless steel housing, yields relatively large amounts of biomass, and enables continuous monitoring of population dynamics through the analysis of perfusates (spent culture fluid) [38]. It has been validated for the maintenance of complex salivary microbial ecosystems and for the in vitro reproduction of interindividual variation within oral microbiotas. Disadvantages of MSD are related to the development of heterogeneous biofilms [13].
Artificial Mouth
Artificial mouth models mimic the in vivo oral niches and habitats to act as a laboratory “microcosm” [39]. It consists of a vessel in which a surface (or surfaces) is inoculated and supplied with a continuous or intermittent nutrient supply; during such experimental procedures, real-time growth and development of dental plaque/biofilm can be investigated (Fig. 9.4) [3, 39, 40]. This system has progressed from simple and basic apparatus to the currently available, highly sophisticated, computer-controlled, multi-station artificial mouth systems. The advanced multiple artificial mouth (MAM) system which was developed by Sissons and his co-workers could be employed for the long-term growth of multiple plaque samples within a standardized, simulated oral environment generated by computer-controlled facilities [40]. The environment and the biofilm pH range can be controlled and manipulated, and oral fluids and periodic pulses of sucrose to model meals were simulated. A chemically defined saliva-like oral fluid analogue is named defined medium with mucin (DMM), which can work well as a “saliva substitute” [41]. Plaque samples grown over several weeks in this system exhibited metabolic behavior and pH profiles typical of natural plaque. It was possible to analyze aliquots during plaque development without contaminating the mature samples [40]. These current applications contain evaluating microbial interactions in simulated dental plaque and similar biofilms and monitor their physical, chemical, biological, and molecular features to a very high degree of accuracy, evaluating potential antimicrobial agent [40, 42]. Besides, several artificial mouth models have been established to study factors influencing the development of primary carious lesions and evaluate caries-preventive agents, which will be represented in “microbial-based mineralization model” part in detail.
Chemostat
Chemostat can provide a homogeneous liquid environment for microbial growth, under highly defined and controllable conditions [3]. Bacteria can be grown at fixed growth rates, enabling single parameters to be varied independently so that true cause-and-effect relationships can be established; the culture can also be sampled repeatedly, facilitating statistical analysis. However, if excessive bacteria grew in chemostat, operational problems may arise from blocked tubing [3]. Prevention of wall growth (change of cells from planktonic to biofilm forms adhering to the chemostat vessel) is also very important, which will cause deviations from “steady-state” growth [43]. Pure culture and mixed culture can both grow in chemostat systems. Marsh developed a consortium, composed of nine bacteria to investigate carbohydrate pulses and pH on population shifts within oral microbial communities [44] and later focus on pH-driven disruption [17]; both of the two studies are very important to his “ecological plaque hypothesis.” The control of pH at neutral values during sugar pulsing was possible only with this type of laboratory model; this experiment could not have been performed in animal or human model studies because of the inevitable pH change when carbohydrates are metabolized [3]. The applications of chemostat for the usual microbiology laboratory are costly and have space considerations. A lot of medium are needed, especially when multiple parallel cultures are needed. Nowadays, some modifications are developed to alleviate the hurdles [43]. For classic chemostat, one of the shortcomings is that the organisms are not in a biofilm; however, recently, there are some modifications for chemostat to involve relevant surfaces to form biofilms. Sterile hydroxyapatite rods or disk can be inserted into chemostat head and immersed in steady-state planktonic cultures for certain periods [45]. Chemostat has also been linked to flow cells to form biofilm. S. Herles and co-worker developed a chemostat flow cell system (Fig. 9.5), in which the chemostat was provided with a continuous source of five species of oral bacteria grown in an artificial “saliva-like” medium. This mixture was pumped through six flow cells, each containing two types of surfaces in which plaque formed, and was subsequently used to compare the antiplaque agents [46]. Furthermore, chemostat can also be used to inoculate a novel biofilm generating model system, the thin film fermenter, in which biofilms of a predetermined depth can be generated, of which a key feature is that biofilms of a predetermined depth can be generated [3].
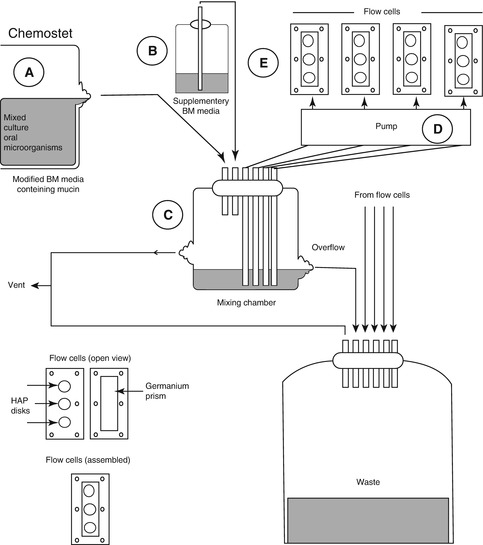
Fig. 9.5
Chemostat flow cell system [46]. A Chemostat containing a mixed culture of 5 oral bacteria, B Vessel containing a supply of supplementary BM medium (without glucose), C The mixing chamber containing the flow from A (1 ml/min) plus 5 ml/min from B, D The pump supplying flow (1 ml/min) from the chemostat to 6 low cells (1ml/min), E Flow cells containing the HAP disks and germanium ATR prisms
9.1.3 Microbial-Based De- and Remineralization Model
The use of microbial-based de- and remineralization model permits more clinical relevant in vitro investigations of dental caries etiology and the properties of caries-preventive agent. In this system, primary caries, secondary caries, pit and fissure caries, root caries, and so on can be developed. Batch culture techniques can be used, and continuous culture techniques were also applied in this system, during which “artificial mouth” was generally involved.
In an in vitro secondary caries model, the occlusal surface of the tooth was sealed with a conventional resin-based fissure sealant; during the process, certain part of the tooth was slightly moistened (contaminated) with saliva to produce marginal gaps, the teeth were infected with Streptococcus mutans in artificial mouth, and the secondary caries can be developed in contaminated regions [47]. This model can also be used to study the relationship of gap size and secondary caries, in which gaps were created by inserting shim stocks with different thickness at the tooth/resin interfaces and Streptococcus mutans was also used as inoculum [48].
Katz and his colleagues developed a pit and fissure caries in artificial mouth. They selected extracted human premolars and molars and inoculated pit and fissures with a concentrated Streptococcus mutans inoculum, which was overlaid with a nutrient layer of 15 % agar, 15 % glycerin, and 5 % sucrose. A filter paper and a thin layer of collodion were placed over the artificially created plaque. These specimens were incubated at 37 °C and continuously washed with artificial saliva (pH neutral) at a regular rate. The acrylic blocks with the tooth specimens were removed daily, and 0.24 % sodium fluoride dentifrice was applied for 3 min with a toothbrush. The latter procedure was repeated 5 days a week, and the whole experiment was conducted for 8 weeks. Subsequently, stereomicroscopic evaluations revealed that the artificial lesions were very similar to those of natural pit and fissure caries. This in vitro caries model contributed somewhat to the study of pit and fissure caries including the study of remineralization due to agents incorporated in toothpaste [40].
When root tissue (disks) was used and putative root-caries pathogens were used, root caries can be modeled in the bacterial system. M. Shu and colleagues developed such root caries model using four putative root-caries pathogens, Streptococcus mutans, Streptococcus sobrinus, Actinomyces naeslundii, and Lactobacillus rhamnosus [20]. Also, saliva can also be used as inoculum to develop the root caries [49].
9.2 In Situ Model in Caries Research
In situ caries models involve the use of appliances or devices which create defined conditions in human mouth to simulate the natural process of dental caries. These models attempt to provide clinically relevant information in a relatively short period without causing irreversible tissue changes in the natural dentition [50]. The advantages of in situ caries model systems compared with clinical trials include [1, 51]:
1.
They have fewer ethical and logistical problems.
2.
They are less costly.
3.
Experimental design can be more flexible, allowing hypotheses to be tested.
4.
The results are acquired in much shorter time.
5.
Better control with the study subjects and better compliance.
Compared with in vitro caries models, in situ caries model is complicated by dietary eating habits, the presence of physiologically secreted saliva, plaque of varying composition and thickness, and a pellicle-coated tooth surface [50], which make it more close to oral cavity than in vitro caries models.
Compared with animal caries model, in situ caries models are conducted in human beings, while animal caries models are conducted in animals. Due to so many differences between animals and human beings, some of the results from animal caries models may not extrapolate to human beings.
The disadvantages of in situ caries model are still obvious:
1.
The number of subjects in in situ caries model is generally limited. Whether the small study population can represent the general population is raised as a question.
2.
The validation of the studies is generally heavily dependent on compliance of the test subjects.
By using in situ caries models, we have the potential to study both fundamental aspects of the caries process, such as to form artificial carious lesions [52], to study de- and remineralization, and to obtain dental biofilm directly in the human mouth [53], as well as more applied research problems such as the effect of food on dental caries and the role of caries-preventive agent in caries prevention [54, 55] in human subjects without actually causing caries in the natural dentition [50]. In situ study designs are highly variable, with models using different hard tissue substrates, a variety of intraoral sites, different exposure periods, the inclusion or exclusion of participants’ diet, the use of different depth recesses and gauze to encourage plaque retention, the use of mesh to protect surface from mechanical disturbance and allow plaque accumulation, and the use of various mineral quantification methods [52, 56].
Both enamel and dentin can be used as hard tissue substrates. For enamel, both the natural enamel surface, which is more suitable for formation of dental plaque biofilm [57], and enamel slab (pieces of extracted teeth), which is suitable for mineralization study [54] to make similar baseline, can be used.
When the in situ caries model was used to assess de- and remineralization, enamel specimens were more frequently used than dentin; however, dentin is useful to simulate root caries. In remineralization study, it is better to produce standardized demineralized lesions in laboratory firstly. These lesions can be sectioned to provide one half lesion for the intraoral appliance and the other half used as the baseline lesion control [56].
However, the outcome of in situ caries models may differ substantially depending on their design, and therefore, the choice of model may significantly influence the conclusions drawn from such studies [58]. For example, the microbial composition of plaque will vary among different teeth in the same mouth, on the same tooth in different people, and even on different surfaces on the same tooth. So the selection of patients for in situ caries model should be carefully done. It makes sense to recruit subjects with similar salivary flow and buffer capacity, with desired microbiological pattern, and even with a preferred immunological profile [3]. Also, plaque that forms with the aid of gauze does not fully resemble “natural” plaque, in either structure or microbial composition [59].
9.2.1 Classification of In Situ Models
In 1990, Wefel grouped in situ model systems into three general types: removable appliances, single-section models, and banding models [60]. Nowadays, some new models were developed, and some models were less used; in situ caries models can be roughly divided into two categories: removable appliances and fixed appliances, in terms of the mobility characteristic.
9.2.1.1 Removable Appliances
Removable appliance is the widely used in situ caries model which can be constituted with acrylic appliances or denture and hard tissue substrates. The model can be exposed extraorally to the challenge, or to a therapeutic regime, to decrease variability in individual mouths. This kind of treatment method can permit the testing of agents or procedures which might be harmful to the natural dentition or ethically unacceptable [61]. If dietary challenges were not considered or oral hygiene is not the influence factor, the model can be taken out of the mouth when subjects are eating or carrying out oral hygiene, to give a high degree of control and to diminish compliance. In an in situ artificial dentin carious lesion study, acrylic palatal appliances containing two bovine dentin specimens, protected with a plastic mesh to allow biofilm development, were involved. The volunteers dripped a 20 % sucrose solution on each specimen four times a day for 14 days; finally, the in situ model produced a deep lesion with a high R value but with a thin surface layer [52]. A removable appliance with three 200-μm-wide grooves cut into bovine dentin disks was used to accumulate plaque, which was later treated with chlorhexidine. Then the in situ plaque with and without chlorhexidine treatment can be well investigated to extrapolate the similar conditions in oral cavity [53].
9.2.1.2 Fixed Appliances
Fixed appliances in in situ caries model are the appliances that can only be removed in the end of the study. They have various forms: banding model such as orthodontic band model which can develop orthodontic non-cavitated (white spot) lesion and can produce a plaque accumulation niche for demineralization. The crown single-section model relies mainly on placement of the sections in plaque-retentive areas below contact points [60]. The enamel can even be bonded to the teeth directly to collect natural plaques on the natural enamel surface [57]. Orthodontic non-cavitated (white spot) lesion models have been used regularly for testing the efficacy of novel remineralization agents; however, this approach cannot be extrapolated to model other types of non-cavitated lesions, for after removing the band, the lesion will not continue to develop [62]. A special in situ caries model is that non-cavitated lesions formed under plaque retention sites on the teeth that are destined for extraction, and the effects of remineralization agents can be tested [56].
9.3 Animal Model in Caries Research
In 1995, the US Food and Drug Administration (FDA) issued the Anticaries Drug Products for Over-the-Counter (OTC) Human Use final rule, establishing the requirement that “all OTC anticaries dentifrice drug product formulations be tested in the animal caries reduction test” [12].
Animal caries models have a long history of successful use in caries research. They are invaluable tools to simulate the natural progression of caries under true biological conditions. Unlike in situ and in vitro systems, which measure isolated components of caries process, animal caries models truly measure caries [4
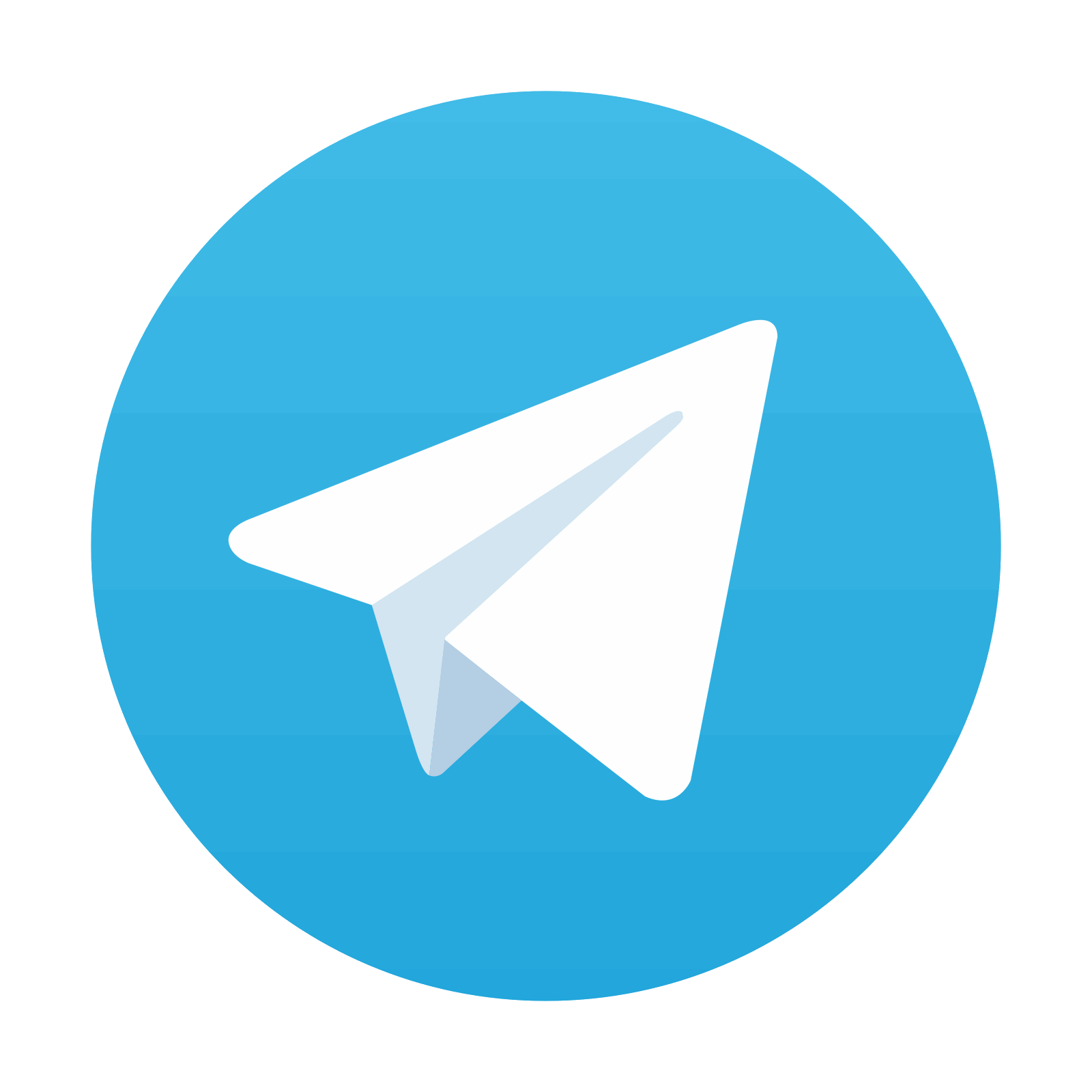
Stay updated, free dental videos. Join our Telegram channel

VIDEdental - Online dental courses
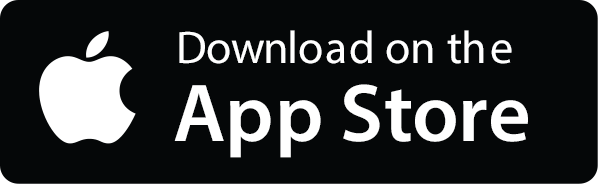
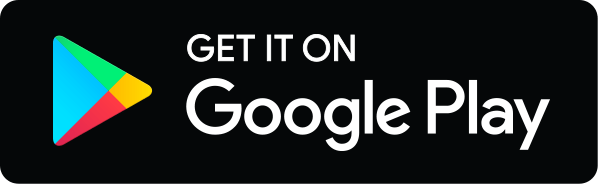