Composition
Concentration
Function
Inorganic
Ca2+
1–2 mM
Modulate demineralization and remineralization
Mg2+
0.2–0.5 mM
Na+
6–26 mM
K2+
14–32 mM
NH4 +
1–7 mM
H2PO4 − & HPO4 2−
2–23 mM
Modulate pH, buffering, modulate demineralization and remineralization
Cl−
17–29 mM
HCO3 −
2–30 mM
Modulate pH, buffering
F−
0.5–5 μM
Modulate demineralization and remineralization, antibacterial action
SN−
0.1–2 mM
Antibacterial action
Organic
Urea
2–6 mM
Modulate pH, buffering
Uric acid
0.2 mM
Indication for kidney function
Amino acids (free)
1–2 mM
Glucose (free)
50 μM
Lactate
0.1 mM
Fatty acids
10 mg/L
Macromolecules
Proteins
1.4–2 g/L
Lubricant, cleanse, aggregate, attach to microorganism, dental plaque metabolism, modulate demineralization and remineralization, antibacterial action
Glycoprotein sugars
0.11–0.3 g/L
Cleanse, aggregate, attach to microorganism, dental plaque metabolism
Amylase
0.38 g/L
Digestion, antibacterial action
Lysozyme
0.11 g/L
Antibacterial action
Peroxidase
3 mg/L
Antibacterial action
IgA
0.2 g/L
Antibacterial action
IgG
14 mg/L
Antibacterial action
IgM
2 mg/L
Antibacterial action
Lipid
20–30 mg/L
3.1.3 Salivary Flow Rate and Influence Factors
There is a broad normal range in individual salivary flow rate. The acceptable flow rate is no less than 0.1 mL/min for unstimulated saliva and 0.2 mL/min for stimulated saliva. In normal condition, the unstimulated whole saliva flow rate is 0.3–0.5 ml/min, while stimulated whole saliva flow rate is 1.5–2.0 ml/min and both of them have wide ranges.
The secretion of salivary glands is mainly controlled by parasympathetic impulses from the salivary nuclei. Several factors influence the salivary flow rate, including the nature and duration of stimulus, the emotional state, water balance of the body, and medication (which will be discussed later). The salivary flow and composition of saliva display large variations during the day [4]. The salivary flow rate usually increases during the day and reaches the acrophase in the late afternoon, around 15.00–17.00 h [2]. It’s almost zero during sleep. This rhythm could be related to change of hormone level during the day and indicates the importance of taking oral hygiene procedures before going to sleep. With the absence of protective effects of saliva, if there is much remaining food and dental plaque in the oral cavity, there would be a great chance for dental caries to happen.
Sympathetic impulses are more likely to influence salivary composition by increasing exocytosis from certain cells. Salivary composition may also be influenced by hormones such as androgens, estrogens, glucocorticoids, and peptide hormones.
3.2 Salivary Influences on Plaque PH and Oral Microflora
3.2.1 Salivary Influences on Plaque PH
Acid-producing bacteria, like Streptococcus mutans and Lactobacillus sp., existed in normal oral flora. These bacteria metabolize fermentable carbohydrates in food and produce variety of organic acids, leading to the reduction of pH value in dental plaque and causing demineralization of hard tissue of teeth.
However, because of the powerful salivary buffering capacity, dental caries is not likely to happen all the time. There are three major buffer systems in saliva: the carbonic acid/bicarbonate, the phosphate, and the protein buffer. The protein buffer mainly takes place in buffering below pH 5, while the optimal buffering range for the carbonic acid/bicarbonate and the phosphate buffer occurs at pH 6.3 and pH 7.2, respectively [5]. The phosphate buffer plays an important role in unstimulated saliva. The secondary phosphate ion, HPO4 2−, could bind a hydrogen ion and form a primary phosphate ion H2PO4 −. During food intake, its effectiveness is limited due to insufficient concentrations of phosphate in saliva.
The carbonic acid/bicarbonate buffer is the most important buffering system in saliva during food intake and mastication. Bicarbonate is secreted within the ducts and. In resting saliva, HCO3 − concentration is as low as 1–2 mM, but it increases with flow rate and reaches 60 mM in stimulated saliva. So during intake, two important events happen: (1) bacteria ferment carbohydrates and produce organic acrid, causing a drop in pH, and (2) the increased salivary flow rate leads to an increased HCO3 − concentration. The carbonic acid/bicarbonate equilibrium (Fig. 3.1) shifts to the left to produce more carbonic acid. With the presence of carbonic anhydrase VI secreted by the serous acinar cells in parotid and submandibular glands, carbonic acid is further driven to be converted into carbon dioxide and water.

Fig. 3.1
The carbonic acid/bicarbonate equilibrium in saliva
Concerning about caries, the pH of saliva may not be as important as the pH of dental plaque. Both fermentable carbohydrate remainders and salivary buffering capacity affected plaque pH. The resting plaque pH refers to the ph of plaque 2–2.5 h after the last intake of dietary carbohydrates and is usually between 6 and 7. Following exposure to fermentable carbohydrates, the plaque pH decreases rapidly during the first 5 min and reaches a minimum after approximately 5–20 min. Unless there is additional ingestion of fermentable carbohydrates, the plaque pH returns slowly to the starting level over 30–60 min. This plaque pH change over time is known as the Stephan curve.
The buffering capacity of saliva affects not only the rate at which the plaque pH decreases but also the minimum value of plaque pH and how long the pH stays at that minimum. The pH value of 5.6 is called a “critical value” which is the pH at which the tissues start to dissolve and cause demineralization. Low buffering capacity of saliva leads to increased rate of plaque pH fall after carbohydrate intake and prolonged time below critical pH, finally leading to an increased risk of dental caries.
Salivary stimulation after food intake also affects plaque pH. When gum is chewed, the flow of saliva increases from a resting value of 0.4–0.5 ml/min to approximately 5–6 ml/min. A series of studies confirmed an obvious and sustained rise in plaque pH when gum was chewed after sugar consumption. Both sugar-containing and sugar-free gum have such effect, but sugar-free gum displays a more powerful effect [6]. Chewing other material that could stimulate salivary secretion such as parafilm also works.
3.2.2 Salivary Influences on Oral Microflora
Besides affecting plaque pH, saliva has profound influence on oral microflora. Saliva provides a nutritious source for oral microflora. Salivary proteins, glycoproteins, peptides, and minerals can stimulate the growth of oral microflora and enhance biofilm formation. On the other hand, saliva displays antimicrobial activities with the presence of a series of immunologic (secretory IgA, IgG, IgM) and non-immunologic factors (enzymes, mucins, proteins, peptides). Lysozyme, lactoferrin, and histatins exhibit bacteriostatic, bacteriocidic activity, while immunoglobulins, mucins, and salivary agglutinin play a role in the oral clearance of bacteria by interfering with receptors on the microbial cell wall.
The immunoglobulins including IgG, IgM, IgA, and secretory IgA (SIgA) form the specific defense system in saliva against bacteria. SIgA is the most abundant immunologic component in saliva, mainly produced by plasma cells located in minor mucous glands. SIgA is non-detectable in neonates but become readily detectable 1 week after birth. It can neutralize pathogenic viruses, toxins, and enzymes produced by bacteria. SIgA can prevent bacteria forming colonies or attaching or penetrating host tissues, kill them directly, or activate complements or provide synergism with innate defense mechanisms. It’s also able to aggregate or clump bacteria, promoting oral clearance. SIgA against streptococcus mutans can be detected in children at the age of 3 years old, and the quantity increases with the length of exposure. Other immunologic components occur in less quantity in saliva. IgG is the only detectable Ig in saliva of neonates and is mainly maternal origin. The concentration of IgG decreases to non-detectable after a few months after birth and appears again after tooth eruption. At this time, IgG mainly comes from gingival crevicular fluid, originating from sera.
Lysozyme is a cationic protein with low molecular weight from the basal cells of striated ducts in parotid glands as well as monocytes and macrophages. It is one of the most important nonspecific defense substances in saliva. Lysozyme catalyzes cleavage of beta-1,4 glycosidic bonds between muramic acid and N-acetylglucosamine residues in peptidoglycan; hence it hydrolyzes bacterial cell wall. Due to the external lipopolysaccharide layer, Gram-negative bacteria are more resistant to lysozyme. Furthermore, nonenzymatic bactericidic effect of lysozyme related to activation of bacterial autolysins is reported as well. It is also documented that this enzyme functions to aggregate bacteria and inhibit bacterial adherence.
There are two categories of salivary peroxidases: human salivary lactoperoxidase (HS-LPO), also termed sialoperoxidase, synthesized in salivary glands, and myeloperoxidase (MPO) formed in polymorphonuclear leucocytes. Salivary peroxidases have enzymatic activity and are able to catalyze the oxidation of salivary thiocyanate and hypothiocyanous acid, inhibiting bacterial metabolism [7].
Salivary chitinase secreted by salivary glands catalyzes the hydrolytic cleavage of chitin. Chitin is a cellular wall compound of yeast cells; hence, chitin may play a role in the protection against yeast.
Mucins are glycoproteins secreted from submandibular and sublingual glands that selectively modulate the microorganism adhesion. There are two genetically distinct mucin groups: highly glycosylated, high-molecular-weight MG1 (>1000 kDa) and single-glycosylated peptide chain, low-molecular-weight MG2 (200–300 kDa). The two groups of mucins display similar carbohydrate chain makeup but have different bacterial adherence ability. MG1 binds tightly to tooth surface and takes part in enamel pellicle formation. MG2 promotes the aggregation and clearance of oral bacteria, including Streptococcus mutans. It’s reported that MG2 predominates in saliva of caries-resistant individuals, while the level of MG1 is higher in caries-susceptible individuals [8].
Salivary agglutinin (SAG) is a high-molecular-weight (approximately 340 kDa), mucin-like glycoprotein aggregate cells in suspension that can be found in parotid and submandibular saliva. SAG is highly glycosylated and extremely sticky. It could bind to hydroxyapatite and is a component of the enamel pellicle and may be involved in the initial adherence of bacteria to the enamel surface.
SAG binds to streptococci surface receptor antigen I/II in a calcium-dependent manner. Furthermore, SAG also binds to SIgA, resulting in synergistic effect of bacterial aggregation which promotes clearance of microorganisms from the oral cavity [9, 10].
Lactoferrin is a glycoprotein with a molecular weight of about 80 kDa. It is a member of a transferring family and able to link to ferric iron in saliva and lead to bactericidal or bacteriostatic effects on bacteria requiring iron for metabolism including streptococcus mutans by iron-depriving effect. This process of starving bacteria of vital nutrients is termed nutritional immunity. It’s reported that lactoferrin is a multifunctional protein having bacteriostatic, bactericidic, fungicidal, antiviral, anti-inflammatory, and immunomodulatory properties [11, 12].
Histatins are a family of peptides rich in histidine, arginine, and lysine residues. At least 12 histatin-like peptides are identified in human saliva. Histatins have antimicrobial activity against some strains of Streptococcus mutans and yeasts. They are implicated in pellicle formation, neutralization of lipopolysaccharides of the external membranes of Gram-negative bacteria, chelation of metal ions, and inhibition of proteinases including metalloproteins and cysteine proteinases.
Saliva provides nutrients to oral microorganisms and supports their growth. On the other hand, saliva has a protective function in maintaining oral health and microbial ecological balance by inhibiting pathogens and regulating pH in oral environment.
3.3 Xerostomia and Its Management
3.3.1 Etiology of Xerostomia
Xerostomia, also termed dry mouth or dry mouth syndrome, is referred to the subjective symptom of oral dryness, which is frequently, but not always, associated with salivary gland hypofunction and a significant reduction of unstimulated salivary secretion. As mentioned above, the normal flow rate of unstimulated saliva is about 0.3–0.5 ml/min, while that of stimulated saliva is about 1.5–2.0 ml/min. It would be considered as hypofunction of salivary glands if unstimulated flow rate is below 0.1 ml/min or stimulated flow rate is below 0.5 ml/min. As there is a wide range of salivary flow rate, a 50 % reduction of individual salivary flow rate would also be considered hypofunction [13, 14].
Saliva is secreted by three pairs of major salivary glands and the minor salivary glands. Any factors that could influence salivary gland function, including diseases of salivary glands, Sjögren’s syndrome, radiotherapy in the head and neck area, the use of certain drugs, etc., might lead to the reduced output of salivary secretion, causing xerostomia. Besides iatrogenic factors, aging could be another important cause for xerostomia. As life expectancy in developed countries keeps increasing, xerostomia is becoming increasingly common in the result of an increased incidence of systemic diseases and a more extensive intake of medication and/or the degeneration and reduced volume of acini. Table 3.2 shows the common causes of xerostomia.
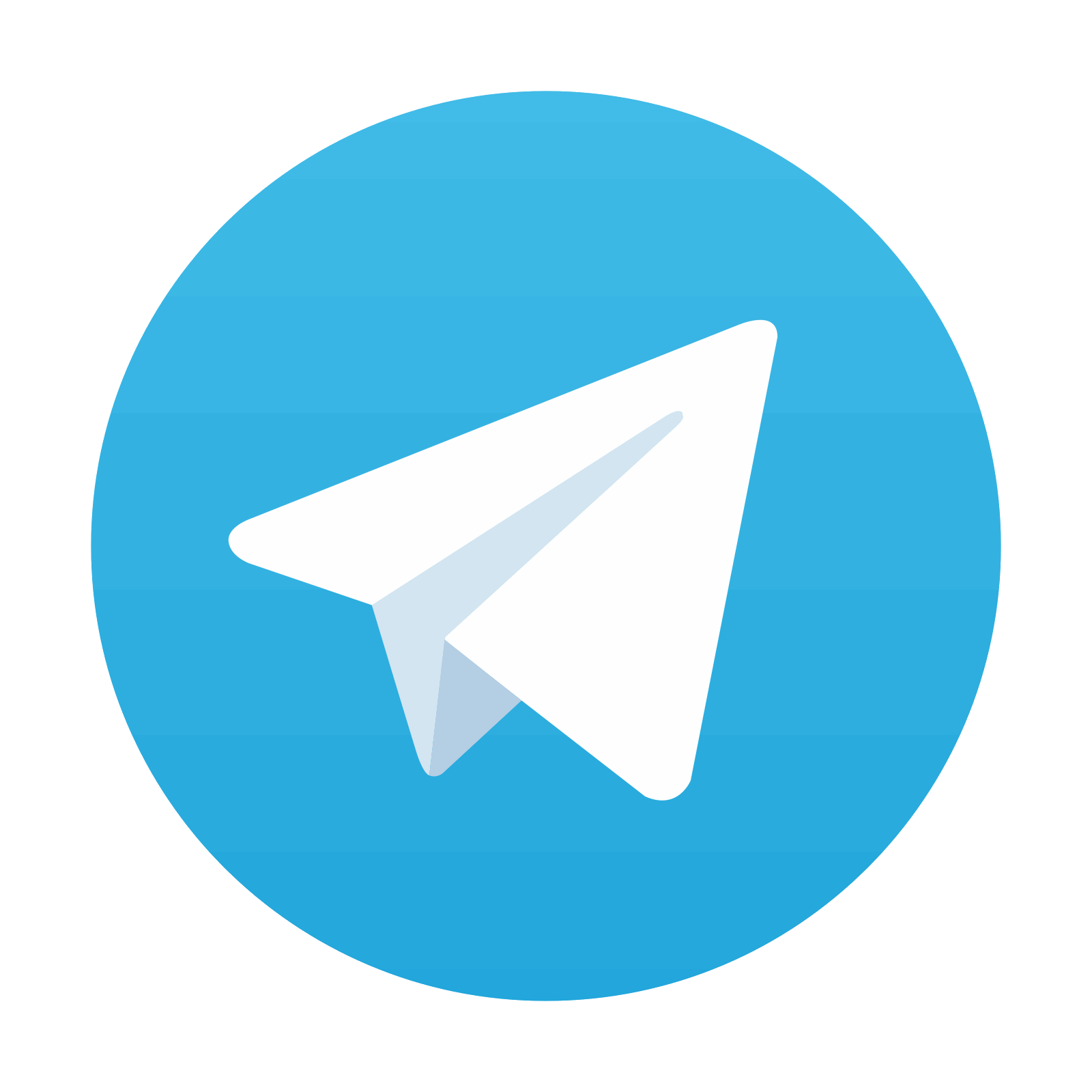
Stay updated, free dental videos. Join our Telegram channel

VIDEdental - Online dental courses
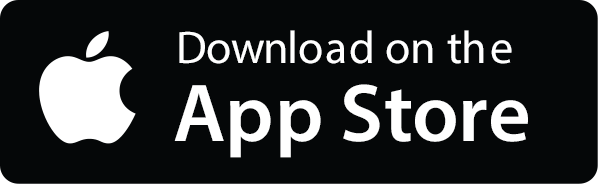
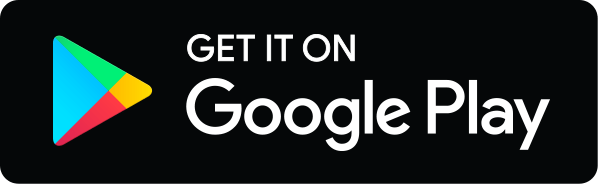