Introduction
In this study, we experimentally evaluated whether complex, mature sutures can be separated using skeletal anchorage and light, continuous forces.
Methods
Twelve adult, 8- to 9-month-old female New Zealand white rabbits were randomly assigned to 1 control group and 2 experimental groups. Open-coil nickel-titanium springs delivered constant forces of 100 g across the sagittal suture to miniscrew implants placed bilaterally in the frontal bone. Sutural separation was measured biweekly. Separation was also measured with microcomputed tomography. Bone formation (mineral apposition) was measured with fluorescent labels. Qualitative histologic analyses of the suture tissues were performed using hematoxylin and eosin staining; osteoclasts were evaluated with tartrate resistant acid phosphatase staining.
Results
All 24 miniscrew implants remained stable throughout the experiment. There was no statistically significant sutural separation in the control group. In the experimental groups, sutural separation was significant ( P <0.05) at all time points after the initial records were taken. The rate of separation was linear during the first 42 days. There were moderate correlations ( R = 0.59-0.89; P <0.05) between miniscrew implant separation and bone marker separation. Mineral apposition rate, which was not measureable in the control group, was significant in the experimental group. The mineral apposition rate was greater between 14 and 28 days than between 28 and 38 days, and it was greater on the ectocranial than on the endocranial surface. Based on the microcomputed tomography analysis, 3-dimensional sutural volume of the experimental group increased significantly ( P = 0.02), but surface area did not ( P = 0.26).
Conclusions
It is possible to separate the sagittal suture of mature rabbits. Sutural separation is limited, indicating involvement of other articulations.
Highlights
- •
Effect of force on mature, nonexpanding sutures was studied in rabbits.
- •
Sagittal sutures were separated with light continuous forces and skeletal anchorage.
- •
New bone formed, and sutural volume increased.
- •
The amount of sutural separation decreases over time.
- •
Bony articulations limit the amount of separation that occurs at this force level.
Orthodontists have expanded midpalatal sutures for over a century. Although it was initially introduced in the United States during the 1860s, palatal expansion was not commonly performed until decades later. Its popularization has been based on the specialty’s understanding of tissue reactions at the sutural level. Maxillary expansion has predominately been used in juveniles and young adolescents, whose sutures are less complex and easily separated. For older adolescents, whose sutures are more complex, expansion requires higher forces.
Expansion in adults has been limited by the beliefs that the midpalatal suture will not split and that the expansion forces may negatively affect the periodontium. There have been many attempts to separate the sutures of adults, but only sporadic cases have been successful. The majority of the expansion in adults has been shown to be due to bending of the alveolus, which is associated with significant morbidities. For adults who need maxillary expansion, surgically assisted rapid palatal expansion or orthognathic surgery is commonly prescribed. Both procedures are costly and associated with a host of morbidities.
Currently, there is no effective, nonsurgical method to separate the mature midpalatal suture in humans. Importantly, sutural expansion in older adolescents and young adults may be possible because the facial sutures maintain their patency until much later in life than commonly thought. Growth of the midpalatal suture is complete by about 14 years of age in girls and 16 years in boys, and the suture increases in interdigitation up to adolescence. Sutural complexity is highly variable and does not appear to change in adults, although sutural bone density increases. Most importantly, facial sutures do not fuse until much later in life and retain the cells necessary for bony remodeling. The sutures of the bones affected by expansion remain patent until later in life.
The purpose of our study was to evaluate whether a complex, mature suture can be modeled and separated using skeletal anchorage and light continuous forces. Theoretically, skeletal anchorage makes it possible to maximize the skeletal and minimize the dental changes. Lighter forces may be necessary for modeling to take place under the biologic constraints of mature sutures.
Material and methods
The sample consisted of 12 adult female New Zealand white rabbits (8-9 months old). The housing, care, and experimental protocol were in accordance with the guidelines of the Institutional Animal Care and Use Committee, Texas A&M University Baylor College of Dentistry (approval BCD 2012-12) in Dallas. The animals were initially quarantined for 3 days, maintained under standard laboratory conditions, and given a stock diet and water ad libitum.
The rabbits were randomly divided into 3 groups: a 42-day loaded group (n = 7), a 42-day control group (n = 3), and a 105-day loaded group (n = 2). The 42-day period was chosen based on a similar study in immature rabbits. The 105-day group made it possible to preliminarily evaluate longer-term expansion effects; the 105-day animals were not used for histology or microcomputed tomography. The sutures of both the 42-day and the 105-day groups were expanded using the same 100-g force level.
All animals were anesthetized intramuscularly with ketamine (35 mg/kg) and xylazine (1 mg/kg). The surgical sites were shaved and disinfected. All procedures were performed under sterile conditions. Local anesthesia was obtained with 2% lidocaine with epinephrine, 1:100,00. Two regions of skin were removed from the skull using a 3-mm punch biopsy instrument, approximately 5 mm lateral to the midsagittal suture, at a point midway between the anterior and posterior orbital rims ( Fig 1 , A ). The punch sites were displaced 3 to 4 mm anteriorly, and 2 sterile stainless steel bone markers (1.0 × 0.3 mm) were tapped into the skull with a custom-made stainless steel appliance. Two custom-made miniscrew implants (MSIs, 3 × 1.7 mm) were placed through the initial punch sites with a manual driver. Postoperative analgesia was controlled with nalbuphine (2 mg/kg, subcutaneously, twice a day, as required).
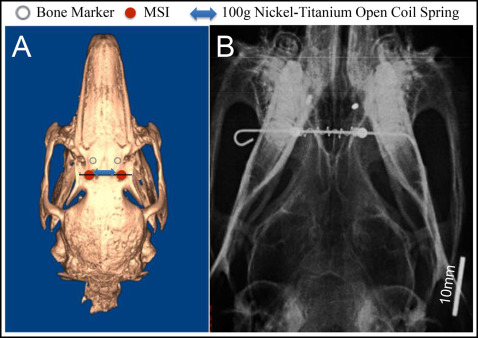
A 0.20-mm stainless steel wire was inserted passively through the holes in the heads of the MSIs and loaded with a 15-mm Sentalloy nickel-titanium open-coil spring clipped to remain passive (0 g) for the control group (GAC, Bohemia, NY). To keep the wire from sliding, the ends were bent with a stop loop and bonded with composite ( Fig 1 , B ). The 100-g force levels were maintained by nickel-titanium coil springs, which remained compressed between 8 and 12 mm.
Sutural expansion was measured at the time of the initial surgery and every 14 days for the first 42 days, as well as at 91 and 105 days for the 105-day group. Measurements were taken under the same anesthesia protocol used for the initial surgery. At each occasion, the animals were weighed, ventrodorsal radiographs were taken, and inter-MSI distances were measured with digital calipers. The distances between the MSIs were measured by 2 operators (R.J.P. and another) and averaged. The radiographs were exposed on phosphor plates at 68 kVp and 10 mA for 12 ms. The source was maintained at 30 cm from the phosphor plate; the animals were secured in the supine position ( Fig 1 , B ) with the bone markers located approximately 3 mm from the plate. A ruler was included in the radiograph so that after the digital images were transferred to the software (version 11.5; Dolphin Imaging, Chatsworth, Calif), they could be corrected. Interbone marker distances were measured by a blinded examiner (R.J.P.). After 4 weeks, 24 radiographs were measured twice to establish intraexaminer method error (0.049 mm) using Dahlberg’s formula (√Σd2/2n) ( Σ d 2 / 2 n ) .
To identify bone-forming regions and quantify mineral apposition rates (MARs), the fluorescent labels calcein (10 mg/kg; Sigma-Aldrich, St Louis, Mo) and alizarin complexone (20 mg/kg intramuscularly; Sigma-Aldrich) were administered to all animals. Calcein was administered at 14 days and again 4 to 5 days before the animals were killed (days 37-38). Alizarin complexone was administered 14 days before the rabbits were killed at day 28.
After day 42, the animals were anesthetized with ketamine and xylazine, and killed by exsanguination by perfusion of saline solution. Fixation was performed with 4% paraformaldehyde. A 1 × 0.5 × 2-cm section of the sagittal suture and adjacent bone was dissected between the MSIs and scanned using a desktop microcomputed tomography system (model 35; Scanco Medical, Brüttisellen, Switzerland) at 55 kVp and with an isotropic resolution of 10 μm. The suture was oriented perpendicular to the image plane of the microcomputed tomography system.
The 3-dimensional (3D) volume was segmented and analyzed using software (version 11; AnalyzeDirect, Overland Park, Kan) by a blinded examiner (R.J.P.). Three regions (anterior, middle, and posterior) of the suture were analyzed in all specimens. Each region contained 100 sequential coronal slices (100*10 μm = 1 mm total), with the middle region located where the wire crossed the suture, and the other regions located approximately 3 mm anteriorly and posteriorly to the middle region ( Fig 2 ). The suture was segmented slice by slice using a gray-scale threshold of 0 to 72. Limits were manually drawn at the superior and inferior edges of the suture and where the suture communicated with the medullary space. After the 3D rendering ( Fig 3 ), the volume and surface area of the space between the sutural margins were automatically calculated for each region. Replicate analyses performed 4 weeks later showed a method error for the 3D volume (0.018 mm 3 ).
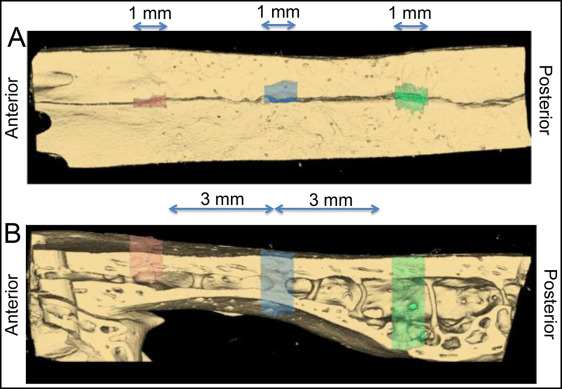
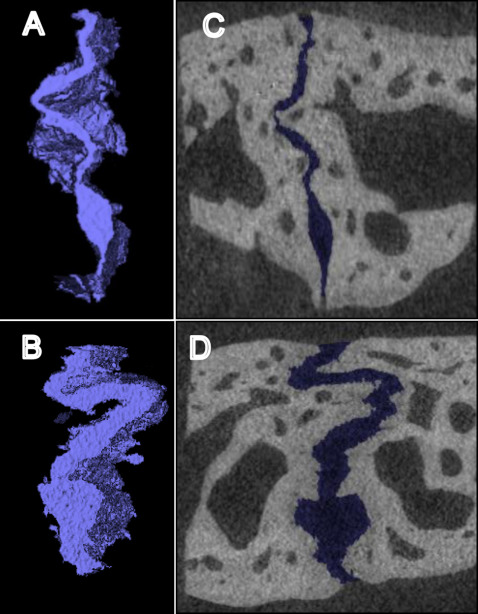
Three slices from each region (first, middle, and last) were exported as TIFF files to OSTEO software (version 13.2; Bioquant Image Analysis, Nashville, Tenn). The suture was then traced, and its length and height were measured. To evaluate the level of interdigitation, the complexity index was calculated:
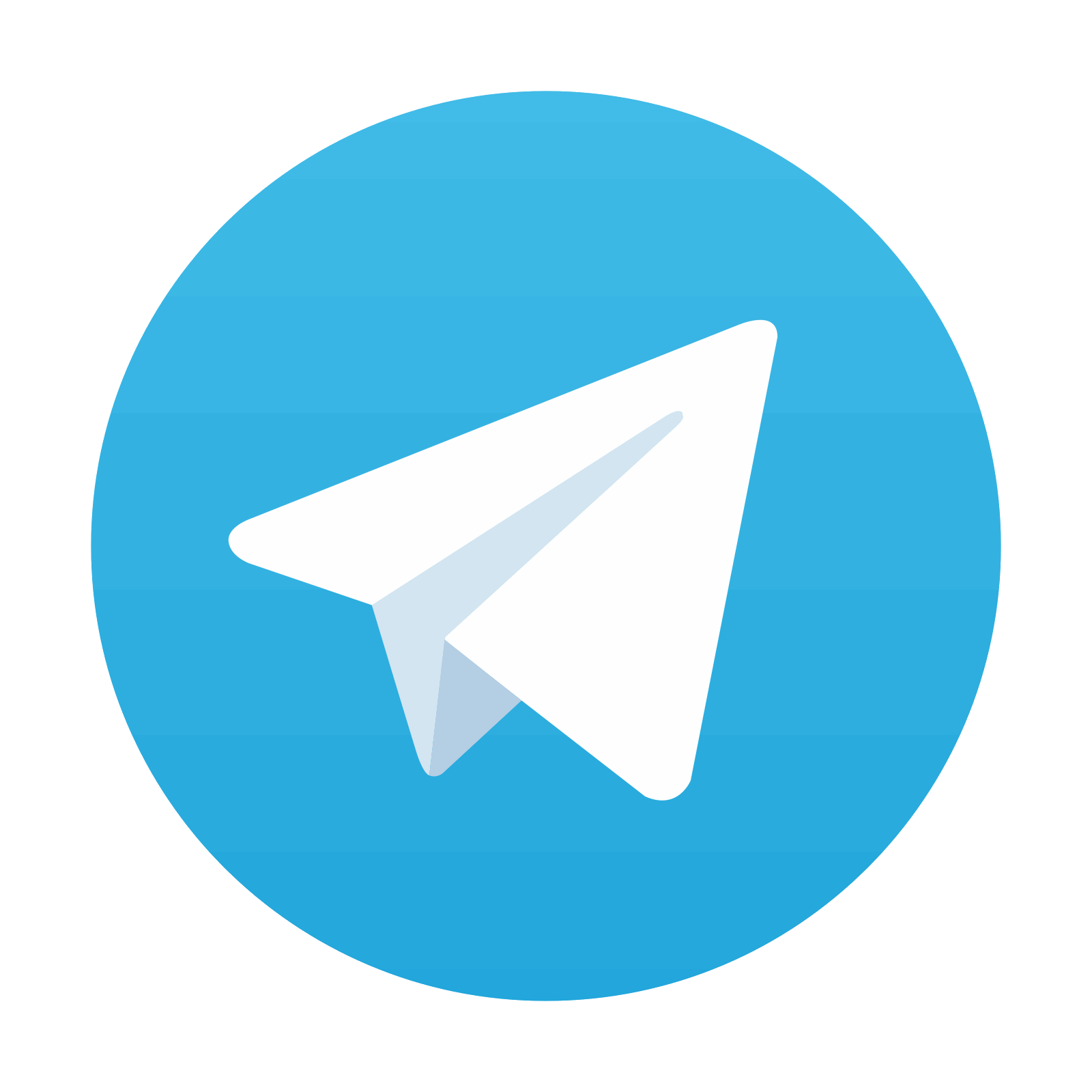
Stay updated, free dental videos. Join our Telegram channel

VIDEdental - Online dental courses
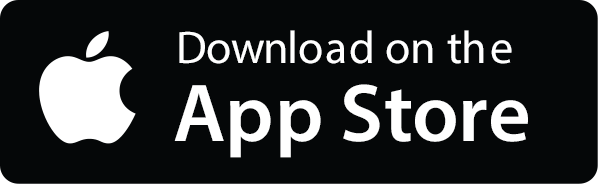
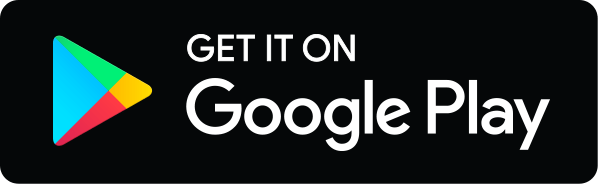