Fig. 8.1
Scanning electron microscopy (SEM) micrograph of dentin etched with 30 % (in a) and 40 % (in b) phosphoric acid for 15 s. Observe the area of demineralized dentin (red)
However, although most dental adhesive manufacturers recommend protocols for solvent evaporation, complete solvent elimination is difficult to accomplish, mainly when the solvent is added to highly hydrophilic adhesive blends (Pashley et al. 1998; Carvalho et al. 2003; Yiu et al. 2005b). As a consequence, the presence of residual water and solvents may further prevent the reactive pendant species to approximate, making cross-linking reaction inside the HL more difficult (Figs. 8.2 and 8.3) (Paul et al. 1999; Ye et al. 2007; Loguercio et al. 2009).
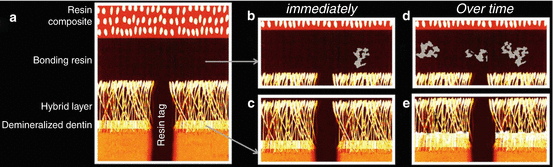
Fig. 8.2
Schematic drawing representing the sequence of resin–dentin bonding degradation over time (Adapted from Perdigao et al. (2013)). (a) Resin–dentin bonding interface. (b) The presence of silver nitrate deposits (in gray) in the adhesive layer soon after bonding indicates the presence of retained water/solvent, as well as areas of inadequate polymerization. (c) Areas of denuded collagen fibers, incompletely infiltrated by resin monomers, are present at the base of the hybrid layer. These collagen fibers are prone to degradation by host-derived proteinases. (d) Degradation continues over time because of water sorption. The polymer swelling leads to the release of oligomers and residual monomers, which are not cross-linked to the main polymer chains. This can be identified by an increase in the number of silver nitrate deposits in the adhesive layer. (e) The area of incomplete collagen infiltration also increases with time (Reprinted with permission)
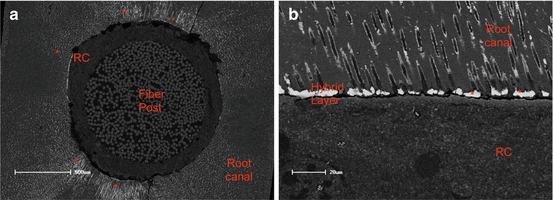
Fig. 8.3
SEM of the apical third of the post cemented as simplified ER adhesive associated to a dual-cure resin cement (RC). (a) Occlusal view of the interface between post and root canal. Observe the higher amount of nanoleakage around the interface between adhesive/resin cement interface and root canal (red asterisks). In (b), in higher magnification, nanoleakage inside the hybrid layer
Thus, instead of achieving optimal macromolecular packing density, the polymer network may have its free space greatly increased to a level directly related to the amount of remaining organic solvent during polymerization. Once the extent and rate of water uptake is dependent upon the chemistry of adhesive systems (Ito et al. 2005a; Malacarne et al. 2006; Malacarne-Zanon et al. 2009; Reis et al. 2013), the poorly polymerized resin will eventually expedite water sorption and compromise the long-term integrity of adhesive–dentin interface (Reis et al. 2013).
Although the bonding mechanisms on root dentin are similar to coronal dentin (Mannocci et al. 1999; Ferrari et al. 2000; Breschi et al. 2009), polymer degradation in root dentin is more problematic than that observed in any other region. As previously mentioned, because of root canal anatomy, removal of phosphoric acid and moisture control when ER adhesive systems are used cannot be successfully accomplished (Breschi et al. 2009). As a consequence, higher amount of residual water remains on the demineralized dentin, mainly in the middle and cervical thirds of the root (Thitthaweerat et al. 2013).
Additionally, attenuation of light emitted by the light-curing unit due to root anatomy may result in even poorer monomer conversion of bonding agent and resin cement layers at the middle and cervical thirds (Roberts et al. 2004; Faria e Silva et al. 2007). The resulting poor polymerization added to the higher content of remaining residual water/solvent will eventually turn the adhesive interface at the middle and apical thirds more prone to hydrolytic degradation than that from the cervical third, leading to lower short- and long-term bond strengths as reported in the literature (Foxton et al. 2003; Monticelli et al. 2006).
8.2.1.2 Self-Etching (SE) Adhesive Systems
The use of SE adhesive systems along with resin cements to bond posts to root canals became an option to overcome the limitations imposed by the technique-sensitive ER adhesion strategy. In contrast to ER adhesive systems, SE adhesives systems have acidic monomers capable of partially involving original smear layer in the HL (Watanabe et al. 1994; Nakabayashi and Saimi 1996). In other words, the acidic monomers penetrate into the smear layer, smear plug, and underlying intact dentin. Therefore, the HL created by SE systems is a combined structure of adhesive resin, collagen fibrils, and minerals. For this reason, SE adhesive systems do not require previous phosphoric acid etching or wet-bonding technique (Watanabe et al. 1994), so they allow clinicians to control the application technique to root canals better than do conventional ER adhesive systems.
However, due to the high hydrophilic monomer and solvent contents, one-step SE adhesive systems have a more hydrophilic nature, so they exhibit higher water sorption rate and hydrolytic degradation than do ER systems (Breschi et al. 2008; Reis et al. 2013). This leads to the creation of HLs that are permeable not only to water from oral environment but also to fluids flowing from the dentinal tubules (Chersoni et al. 2004; Tay et al. 2004; Hashimoto et al. 2004b). In addition, as dual-cured resin cements are usually required when cementing posts to root canals, a chemical incompatibility between acidic monomers from simplified self-adhesive systems and self-curing components of dual-cured resin cements has been reported (Sanares et al. 2001; Tay et al. 2003b; Arrais et al. 2009).
When light emitted by light-curing units is severely attenuated at the apical third due to root anatomy and the polymerization of resin cement solely relies on self-curing components, the interaction between acidic monomers from self-etching adhesive systems or even from simplified two-step ER systems and resin cement layer will not allow the cement polymerization to occur at the apical third (Sanares et al. 2001; Arrais et al. 2009). This incompatibility between SE adhesive systems and dual-cured resin cements results in higher permeability and hydrolytic degradation of the adhesive layer (Tay et al. 2003b).
8.2.1.3 Self-Adhesive Resin Cements
Recently, following the trend toward simplification, manufacturers have developed resin cements that do not require previous application of bonding agents, the so-called self-adhesive resin cements. These cements have acid-functionalized monomers, such as with 4-methacryloxyethyl trimellitic anhydride (4-META) and pyromellitic glycerol dimethacrylate (PMGDM), or phosphoric acid groups, as with 2-methacryloxyethyl phenyl hydrogen phosphate (phenyl-P), 10-methacryloyloxydecyl dihydrogen phosphate (MDP), bis(2-methacryloxyethyl) acid phosphate (BMP), and dipentaerythritol pentaacrylate monophosphate (Penta-P), in their composition to achieve demineralization and bonding to the tooth surface (Ferracane et al. 2011).
When self-adhesive resin cements are mixed, the acidic monomers create a pH ranging between 1.5 and 3 to gently demineralize dentin and enamel surfaces (Ferracane et al. 2011). The acidic groups bind with calcium in the hydroxyapatite to form a stabilizing ionic attachment between the methacrylate network and dentin. Ions released from the acid-soluble filler neutralize the remaining acidic groups to create a chelate-reinforced three-dimensional methacrylate network. For the first minutes, the cements are fairly hydrophilic, which allows proper wetting and adaptation to the tooth surface.
As the acid functionality is consumed through reaction with calcium from hydroxyapatite and a variety of metal oxides from the cement ion-leachable fillers, these materials become more hydrophobic (Ferracane et al. 2011), so these products would be less prone to hydrolytic degradation than simplified ER and SE adhesive systems. Although short-term results have shown better results in terms of bond strength promoted by these cements in comparison to those obtained with conventional dual-cured resin cements (Sarkis-Onofre et al. 2014), recent long-term studies have shown a drop in bond strength of posts cemented with self-adhesive resin cements over time (Mazzoni et al. 2009a; Marchesi et al. 2013). In general, researchers have attributed this decrease in bond strength to the plasticization of the polymer matrix to water, as these cements may not all shift to neutrality and hydrophobicity as expected (Mazzoni et al. 2009a; Goracci and Ferrari 2011; Marchesi et al. 2013).
Overall, hydrolytic degradation may be considered the main mechanism responsible for the decrease in bond strength over time when adhesive systems are applied to in vivo coronal dentin (Breschi et al. 2008, 2009; Perdigao et al. 2013; Reis et al. 2013). In that scenario, fluid movement through dentin tubules toward the adhesive interface, along with residual water from wet-bonding technique when ER systems are used, or water/solvent present in the bonding agents, is responsible for the hydrolytic degradation within the HL (Breschi et al. 2008, 2009; Perdigao et al. 2013; Reis et al. 2013). Nonetheless, no fluid movement through dentin tubules is observed in the intra-radicular dentin. Moreover, direct intraoral exposure of the post-composite interface is usually avoided by immediately restoring a tooth with a direct composite restoration or a crown, which means that water is not in direct contact with the bonding/luting/post system (Vano et al. 2006; Ferrari et al. 2008 ).
Indeed, only in the worst-case scenario, when the coronal restoration fails to seal the margins and oral fluids reach the bonding/luting/post, faster polymer degradation may be expected. For this reason, despite the evidence that water does have important effects on polymer degradation of ER, SE, or even self-adhesive resin cements, water from the dentin tubules should not be regarded as the main factor related to aging of the adhesive interface in the intra-radicular dentin (Breschi et al. 2009).
8.2.2 Degradation of Collagen Fibrils
After endodontic treatment followed by the use of calibrated burs to prepare the post space, intra-radicular and coronal dentin present similar composition and structure (Ferrari et al. 2000). This substrate is a nonhomogeneous tissue characterized by the presence of tubules extending from the pulp to the root periphery (Ferrari et al. 2000), so intertubular and peritubular dentin are present. For these reasons, degradation of collagen fibrils within the HL at the intra-radicular dentin may be similar to that observed in the coronal dentin (Breschi et al. 2009).
Recently, it has been reported that the enzymes involved in the breakdown of the collagen matrices during the pathogenesis of dentin caries (Tjaderhane et al. 1998; van Strijp et al. 2003) and periodontal disease (Lee et al. 1995) have potential and relevant implications in the degradation of resin–dentin-bonded interfaces (Pashley et al. 2004). More specifically, dentin collagen fibrils contain inactive proforms of proteolytic enzymes called matrix metalloproteinases (MMPs), secreted as proenzymes (zymogens), that were identified in both odontoblasts and mineralized or demineralized human dentin (Bourd-Boittin et al. 2005; Mazzoni et al. 2007; Sulkala et al. 2007). Several MMPs have been identified in mineralized human dentin, in an inactive state – MMP-8 collagenase (Sulkala et al. 2007), MMP-2 and MMP-9 gelatinases (Mazzoni et al. 2007, 2009b), and MMP-20 enamelysin (Sulkala et al. 2002). Although MMP-2 and MMP-9 are considered as gelatinases, other researchers have described collagenolytic activity associated with these two MMPs (Aimes and Quigley 1995; Garnero et al. 2003).
MMPs can be activated by proteinases or by some chemical agents, including reactive oxygen species. In other words, such enzymes can be activated when exposed to acidic agents during adhesive bonding procedures. If these matrix-bound, activated MMPs are not fully infiltrated with adhesive resin, they may be able to slowly degrade the collagen fibrils inside the resin–dentin-bonded interface (Carrilho et al. 2007). Therefore, the degradation of collagen fibrils is dependent upon the lack of proper collagen fibril encapsulation by resin monomers and MMP activation.
For instance, the HL thickness and the resin tag density decrease from the coronal to the apical third of a root (Ferrari et al. 2000). In addition, other authors noticed that the penetration of adhesives inside radicular dentin proved to be complete in only one-third of extracted teeth in the apical third and in two-thirds of the samples in the middle and coronal thirds (Mannocci et al. 2003).
Regarding the dentin adhesives, when ER bonding agents are applied to a previously acid-etched dentin, a decreasing gradient of resin monomer diffusion within the acid-etched dentin (Wang and Spencer 2002) results in incompletely infiltrated areas along the bottom of the HL that contain denuded collagen fibrils (Wang and Spencer 2002; Hashimoto et al. 2003; Spencer et al. 2004) as a consequence of previous acid etching. Therefore, the discrepancy between the depth of the exposed collagen layer without surrounding minerals and resin infiltration creates an exposed demineralized dentin zone under the HL (Figs. 8.2 and 8.3) (Spencer and Swafford 1999; Pioch et al. 2001). Curiously, intense MMP-2 and MMP-9 activities were detected at the basal part of the HL (De Munck et al. 2009; Breschi et al. 2010).
Conversely, when SE adhesive systems are applied to dentin, the smear layer is completely or partially incorporated into the bonding interface, providing simultaneous demineralization and infiltration during the application of the acidic monomer. For this reason, researchers now believe that simultaneous demineralization and monomer infiltration might overcome the shortcomings related to the presence of an exposed collagen network within the bonding interface created by phosphoric acid in ER adhesives. However, incomplete infiltration has also been observed with SE adhesives based on the presence of nanoleakage within the HL (Tay et al. 2002; Tay and Pashley 2003) as a result of nanometric-sized channels for water impregnation into bonding interfaces (water–trees). In both scenarios, when dentin MMPs are exposed and activated by SE or ER adhesive systems (Mazzoni et al. 2006), these enzymes degrade type I collagen (Carrilho et al. 2009).
As previously described in this chapter, regardless of the bonding strategy when using simplified adhesives (two-step ER and one-step SE adhesive systems), by combining hydrophilic and ionic resin monomers into the adhesive solution, the bonded interface would benefit from a non-solvated hydrophobic resin coating. This leads to the creation of HLs that are permeable not only to water from oral environment but also to water flow coming from the dentinal tubules (Chersoni et al. 2004; Tay et al. 2004; Hashimoto et al. 2004b). For this reason, the adhesive interfaces formed with simplified adhesives are considered semipermeable membranes (Tay et al. 2002; Carvalho et al. 2003; Tay and Pashley 2003). The use of a non-solvated hydrophobic resin coating will be discussed in this chapter.
As a consequence, the elution of resin from hydrolytically unstable polymers within the HL results in further exposure of collagen fibrils (Perdigao et al. 2013). Therefore, in root dentin, under the condition of a breach of the coronal seal, or in the case of the water-rich simplified SE adhesives, water may promptly permeate these HLs, allowing the activated MMPs to exert their hydrolytic function upon the collagen fibrils. In addition, recent studies have shown that by-products of both root canal sealers (Huang et al. 2008) and bacteria related to endodontic infections (Itoh et al. 2009) were able to activate at least proMMP-2 and proMMP-9, two of the dentinal MMPs that are thought to be involved with the degradation of collagen fibrils within resin-bonded dentin interfaces (Mazzoni et al. 2007, 2009b). Within this context, this scenario becomes more critical in root canals as naturally occurring tissue inhibitors of matrix metalloproteinases (TIMPs) that are normally present in extracellular matrices for regulating MMP activities (Reynolds and Meikle 1997; Malemud 2006) are depleted after the extirpation of the pulpal tissues and filling of the root canals with synthetic materials.
In addition to MMPs, different cysteine cathepsins are also expressed in human pulp tissue or odontoblasts and present in human dentin (Tersariol et al. 2010). Cathepsins, a family of lysosomal papain-like cysteine proteases, can degrade most of the extracellular matrix proteins, such as collagen, laminin, and proteoglycans (Turk et al. 1997; Dickinson 2002), and their activity can be found in both sound and carious human dentin (Tersariol et al. 2010; Nascimento et al. 2011). As cathepsins are autoactivated in slightly acidic environments (Dickinson 2002), some authors recently noticed that these endogenous enzymes can be activated by low-pH SE adhesive systems (Zhang et al. 2014). According to these authors, the acidic monomers can demineralize the dentin matrix and expose and activate these enzymes. Therefore, along with MMPs, cathepsins might also contribute to the endogenous proteolytic activity of dentin, resulting in collagen degradation within the HL, which may influence dentin bond durability (Liu et al. 2011; Tjaderhane et al. 2013b).
In conclusion, in order to achieve long-term post bonding to root canals, care must be taken to ensure that exposed collagen fibrils are fully enveloped by resin monomers after ER or SE adhesive systems are applied to avoid collagen exposure to such enzymes. In addition, remaining water and residual solvent should be removed as much as possible, and the bonding agents must be properly exposed to light emitted by the light-curing unit so optimal polymerization can be obtained. Due to the limitations imposed by the root canal anatomy, other clinical approaches may be required to fulfill these conditions.
8.3 How to Improve the Resin–Dentin Bond Stability
Based on the foregoing discussion, one may assume that the quality of the adhesive interface will rely on the use of techniques that favor the formation of a fully resin- impregnated HL, with proper enveloping of the exposed collagen fibrils with a highly cross-linked polymer. Several studies have focused on the effects of modified standard clinical protocols to obtain adhesive interfaces with higher resistance to degradation. Unfortunately, most of the in vitro studies were conducted on coronal dentin and most of them still lack evaluation on root dentin. On the other hand, considering the similar composition and structure of coronal and root dentin, the protocols with promising results on coronal dentin will probably improve the immediate and long-term adhesion of fiber posts to root dentin.
In summary, these clinical protocols focus on (1) improving resin impregnation into mineralized and demineralized dentin substrates, (2) improving the strength of the polymer formed by the adhesive systems, and (3) improving the resistance of collagen fibrils to enzymatic degradation. Some clinical approaches may improve bond durability using two or even three of these mechanisms; however, for didactic reasons, they will be classified into one of these approaches.
8.3.1 Improving Resin Impregnation into Mineralized and Demineralized Dentin Substrates
8.3.1.1 Preparation of Root Canal with Diamond Bur
The use of a phosphoric acid after post space preparation with a carbide bur resulted in discontinuous areas of deep intertubular demineralization alternating with areas characterized by open tubules and other areas covered by debris, smear layer, and gutta-percha and/or sealer remnants. This makes the chemical dissolution of this layer rather difficult (Serafino et al. 2004; Breschi et al. 2009). On the other hand, when the preparation of the root canal was performed with a diamond bur, the same etching procedure was capable of removing the smear layer more efficiently, ensuing more open dentin tubules and less smear layer remnants (Gomes et al. 2012).
As no manufacturer provides diamond burs for root wall preparations, clinicians may abrade the root walls with a diamond bur after the use of the manufacturer-provided carbide bur. In this case, the use of a diamond bur with similar diameter will replace the acid-resistant smear layer produced by the carbide bur with a smear layer more susceptible to phosphoric acid dissolution.
8.3.1.2 Use of a Liquid Acid Etchant
As previously reported, dissolution of the smear layer remnants using a phosphoric acid gel is not effectively accomplished in the root dentin walls. After endodontic treatment, obturation, and post space preparation, SEM analysis of canal walls along post space shows large areas covered by smear layer, debris, and sealer/gutta-percha remnants not available for adhesive bonding and resin cementation of fiber posts (Serafino et al. 2004).
Etchants with a gel consistency preclude a close interaction between the acid and the dentin substrate. Some recent studies have reported that liquid phosphoric acid applied with an endodontic needle yielded better smear layer removal from the canal wall and higher bond strengths when an ER adhesive system was used, especially if applied actively (Salas et al. 2011; Scotti et al. 2013).
8.3.1.3 Vigorous Adhesive Application
The gentle application of simplified ER systems limits the diffusion of resin monomers (especially those with high molecular weight) into the wet demineralized root dentin. This is attributed to factors such as the difficulty in the management of moisture inside the root canal. If the adhesive is rubbed vigorously, the adhesive solution may be better drawn into the dentin collagen network (Jacobsen and Soderholm 1998; Reis et al. 2007a), increasing solvent diffusion outward and improving the polymer cross-linking inside the HL.
Recently, Cuadros-Sanchez et al. (2014) evaluated three simplified ER adhesives into the root canal when applied as per manufacturers’ instructions or with the aid of a sonic device (to simulate vigorous application). The authors reported increased bond strength, higher in situ degree of conversion of the adhesive, and reduced nanoleakage mainly in the apical third for the groups where adhesive was applied with the sonic device.
Promising results were also obtained when this sonic device was used for application of SE adhesives (unpublished data). For SE adhesives, vigorous agitation has an additional advantage – carry fresh acidic resin monomers to the basal part of the demineralized dentin, producing a deeper demineralization and carrying more resin, which, in turn, promotes a better interaction with the smear layer and underlying dentin. In comparison with the manual vigorous application, the use of a sonic device may provide a less sensitive procedure, but it warrants further evaluation.
8.3.1.4 Multiple Coats
It has been demonstrated that the application of multiple coats of simplified ER and SE adhesives to coronal dentin yields higher immediate bond strengths (Hashimoto et al. 2004a; Ito et al. 2005b) and also makes the adhesive interface less prone to degradation over time (Reis et al. 2008). For both types of adhesives (i.e., ER and SE), the application of multiple coats improves the saturation of the HL with resin monomers while favoring solvent evaporation. Additionally, additional layers of unpolymerized comonomers of SE adhesives may improve the etching ability of the adhesive by carrying more acid monomers to the basal part of the HL to replace those that were already buffered by dentin (Camps and Pashley 2000).
The benefits of this approach into the root canal have not been investigated so far but may likely be similar to those of coronal dentin. This protocol, however, should be applied with caution as multiple adhesive coats may also increase the thickness of the adhesive layer, which may in turn affect the post seating inside the post space. The use of paper points to remove the excess of solvent may prevent such undesirable consequences.
8.3.2 Improving the Strength of the Polymer Formed by Adhesive Systems
8.3.2.1 Hydrophobic Resin Coating
One way to counteract the hydrophilicity of simplified adhesives is to place an additional coating of hydrophobic resin onto the polymerized simplified adhesive. The use of a hydrophobic coating after the application of simplified SE and ER adhesive systems results in a thicker and more uniform adhesive layer with low concentration of retained water and solvent and significant reduction in the fluid flow rate (King et al. 2005; de Andrade e Silva et al. 2009).
This technique is also capable of eliminating the chemical and physical incompatibility of simplified SE adhesives with chemically cured composites (King et al. 2005; Van Landuyt et al. 2006). Dentin adhesive systems that utilize the separated non-solvated hydrophobic bonding resin (three-step ER and two-step SE) show higher degree of polymerization and less permeability to water (Cadenaro et al. 2005; Breschi et al. 2007).
The application of an extra hydrophobic resin coating over a one-step SE adhesive would convert the simplified adhesive in a more stable two-step SE adhesive. In turn, if the hydrophobic coating is applied over a two-step ER adhesive, this would become a more stable three-step ER adhesive. The simplified adhesive would be further concentrated with more hydrophobic monomers from the additional surface coating (Breschi et al. 2008), as well as shown by Lombardo et al. (2008). Consequently, a more stable resin–dentin interface can be formed over time (Reis et al. 2008). This protocol transforms a simple-layer adhesive into a multilayer one, with the difference that the most hydrophilic simplified adhesive is photoactivated before the application of the most hydrophobic, non-solvated bonding resin.
More densely compacted HLs are produced by reducing the concentration of unreacted monomers between the primed and bonded layers (de Andrade e Silva et al. 2009). This approach, however, should be carried out with caution to avoid the formation of a thick layer of adhesive that would interfere with the post seating inside the post space.
8.3.2.2 Ethanol Wet Bonding
As previously mentioned, simplified ER and SE adhesives are composed of hydrophilic monomers with higher potential for water sorption and degradation. Unfortunately, hydrophobic resins, as neat Bis-GMA/TEGDMA resins, are insoluble in water-saturated dentin. The rationale behind ethanol wet bonding (Breschi et al. 2007; Sadek et al. 2008) is to use ethanol to replace water in acid-etched collagen so that hydrophobic resins can infiltrate the demineralized dentin to create hydrophobic HLs.
Hydrophobic resins absorb little water from dentin (Sadek et al. 2008). The application of hydrophobic resins to ethanol-wet dentin may provide resin interfaces with a fivefold reduction in the water sorption rates (Yiu et al. 2004; Ito et al. 2005a; Malacarne et al. 2006). Also, as MMPs become inactive in the absence of water, the replacement of water with an organic solvent, such as ethanol, is also a potential mechanism for extending the longevity of resin–dentin bonds (Tjaderhane et al. 2013a). The ethanol wet bonding technique yielded higher immediate bond strength and lower nanoleakage in root canals (Duan et al. 2011; Pei et al. 2012). Stable bond strengths to intra-radicular dentin were reported after 6- and 12-month in vitro evaluations (Bitter et al. 2014; Ekambaram et al. 2014), although this benefit is not supported by other data (Cecchin et al. 2011).
The ethanol saturation is achieved by using a series of ascending ethanol concentrations, taking approximately 3–4 min, which defies the principles of user-friendliness and technique simplification (Osorio et al. 2010). A recent study, however, showed promising results using simpler protocol: the authors applied a higher concentration of ethanol for 60 s (Sauro et al. 2010). This simplified protocol can be translated to a routine clinical practice without much difficulty.
8.3.2.3 Improved Polymerization Through the Root Canal
Manufacturers of some ER and SE adhesives provide a separate bottle of activator solution containing ternary catalyst that must be mixed with the adhesive before application. However, these materials also depend on light curing to reach a high degree of conversion (Faria-e-Silva et al. 2008).
Similarly, dual-curing resin cements develop better mechanical properties when they are exposed to curing light (Pegoraro et al. 2007; Manso et al. 2011). Therefore, exposure to curing light has been suggested even when dual-cure cements are used (Caughman et al. 2001; Goracci et al. 2008; Breschi et al. 2009; Wu et al. 2009). Thus, to overcome the insufficient light diffusion in the narrow post space, clinicians have been advised to use a high-intensity light-curing unit and/or prolong the irradiation time of adhesive systems/resin cements to improve the adhesion of adhesives/resin cement to root canal dentin (Akgungor and Akkayan 2006; Aksornmuang et al. 2008; Teixeira et al. 2009; Miguel-Almeida et al. 2012).
Extension of the light-curing time was found to be effective to improve the bond strength to root dentin (Aksornmuang et al. 2006). The use of translucent post is not considered a definitive solution, mainly because it did not transmit enough light to the apical third of the root canal (Teixeira et al. 2006; dos Santos Alves Morgan et al. 2008; Goracci et al. 2008). Other options, such as using a LED fiber or a transparent light-guiding attachment to deliver light into the deepest parts of the root canal, might be considered as viable options (Goracci and Ferrari 2011).
8.3.2.4 Oxalate-Containing Desensitizers
One of the drawbacks of using simplified adhesive systems is that they behave as semipermeable adhesive interfaces that allow transudation of fluids from the underlying dentin. Dentin permeability of root dentin can be reduced by applying oxalate-containing desensitizers (Gillam et al. 2001; Tay et al. 2003a; Garcia et al. 2010). Following acid etching, dentin permeability is significantly increased by removal of the smear layer. The sequential application of an oxalate desensitizer and a two-step ER adhesive on acid-etched hydrated dentin reduces convective water fluxes through the dentin. This allows better contact of the resin cement and the adhesive layer, preventing the well-known physical incompatibility between simplified adhesives and resin cements.
Depletion of calcium ions by the use of phosphoric acid on dentin forces the oxalate ions to diffuse further down into the dentinal tubule, until calcium ions are encountered for reaction (Tay et al. 2003a). Oxalic acid (pH 2.3) is acidic enough to etch dentin and liberate sufficient calcium ions to form insoluble calcium oxalate crystals that occlude patent tubules in exposed dentin. This can also limit the osmotically induced water movement during bonding procedures, thus decreasing the amount of water entrapped within these adhesives.
Although this technique manages well the physical incompatibility between adhesive and resin cements, its use is restricted to ER adhesives, as oxalates must be applied on acid-etched dentin. Another disadvantage of the use of oxalate-containing desensitizer is that its use with fluoride-containing adhesives or adhesives with pH lower than 2.8 should be avoided as they would dissolve the oxalate crystals (Yiu et al. 2005a).
8.3.3 Improving the Resistance of Collagen Fibrils to Enzymatic Degradation
8.3.3.1 Chlorhexidine (CHX)
Despite its long-lasting use as a disinfectant and antimicrobial agent in restorative dentistry and endodontics, especially as a final irrigant of root canals (Mohammadi and Abbott 2009; Gomes et al. 2013), only recently has CHX been employed as a protease inhibitor to preserve the HL against degradation through inhibition of MMPs (Pashley et al. 2004) and cysteine cathepsins (Tersariol et al. 2010) in coronal and root dentin.
The application of CHX-containing aqueous primer during the adhesive procedure did not influence the adhesion to the root canal for ER and SE adhesives (Lindblad et al. 2010; Pelegrine et al. 2010). The use of CHX to preserve the root bond strengths and the integrity of the HL with time is controversial, because some studies have shown that the application of CHX preserves the push-out bond strength in post bond cementation (Cecchin et al. 2011; Bitter et al. 2014; Toman et al. 2014), while other studies did not show any difference when compared to control after 12 months of water storage (Leitune et al. 2010; Cecchin et al. 2011; Bitter et al. 2014; Ekambaram et al. 2014). Differences in the type and brand of adhesive systems and resin cements, as well as methodological differences, can explain these controversial results.
Although lower CHX concentrations have been reported to inhibit the matrix-bound MMPs and cathepsins (Gendron et al. 1999; Scaffa et al. 2012), the application of 2 % CHX has been recommended for final root canal irrigation in endodontics because 2 % CHX solution has better antimicrobial properties than lower CHX concentrations (Mohammadi and Abbott 2009) with higher substantivity to root canal dentin (Basrani and Lemonie 2005). All these advantages may prevent long-term recontamination of the canal (Roach et al. 2001; Rosenthal et al. 2004). As no side effects in the long-term results were observed with CHX application, 2 % CHX could be considered a plausible alternative for preserving long-term bond strength.
The use of CHX-containing phosphoric acid may be an alternative to the application of an aqueous solution of 2 % CHX (Stanislawczuk et al. 2009, 2011). Although it has not been yet evaluated in root dentin, 2 % CHX results in stable bond strengths after 2 years in coronal dentin (Stanislawczuk et al. 2009, 2011) and may be considered an alternative when ER adhesives are used for adhesive procedures in the root canal.
8.3.3.2 EDTA
EDTA (ethylenediaminetetraacetic acid) is one of the most commonly used agents for irrigation during mechanical instrumentation of the root canal system. It has been also used during mechanical root periodontal therapy (Hulsmann et al. 2003). EDTA decalcifies smear-layer-covered dentin superficially, but its action is self-limiting. EDTA acts as a chelating agent, reacting with calcium ions from dentin hydroxyapatite, and forms soluble calcium salts (Hulsmann et al. 2003). As EDTA is an effective Zn2+ and Ca2+ chelator, it inhibits MMP activity (Osorio et al. 2011a; Thompson et al. 2012). In fact, EDTA has inhibitory effect against human dentin MMP-2 and MMP-9 when applied for 1–5 min (Osorio et al. 2011a; Thompson et al. 2012).
As a consequence, the use of EDTA has been suggested as a dentin pretreatment for dentin adhesives. The results showed an increase in resin–dentin bond strengths, compared to phosphoric acid and other types of dentin-conditioning agents (Torii et al. 2003; Jacques and Hebling 2005; Sauro et al. 2010). EDTA also preserved the dentin–adhesive interface in in vitro longevity tests (Sauro et al. 2010).
One drawback is that EDTA is removed from dentin by extensive rinsing with water. There may be no residual EDTA left to inhibit the activity of MMPs (Osorio et al. 2011a; Thompson et al. 2012). It has also been shown that collagen in EDTA-demineralized dentin is as susceptible to MMP degradation similarly to collagen in dentin etched with phosphoric acid (Osorio et al. 2011b). Therefore, it is not clear if the preservation of HL with EDTA is a result of a shallow dentin demineralization or from MMP inhibition.
8.3.3.3 Cross-Linkers
The base of resistance and longevity of dentin matrix is their intrinsic intermolecular and intermicrofibrillar cross-linking. So, it is believed that an increase in the extent of cross-linking of the collagen fibrils prior to adhesive application may result in increased durability (Liu et al. 2011; Bedran-Russo et al. 2014).
Collagen cross-linkers have been primarily used in demineralized dentin to enhance the mechanical properties of these substrates (Bedran-Russo et al. 2014). More recently, however, it was observed that cross-linking agents also have anti-MMP properties (Liu et al. 2011; Bedran-Russo et al. 2014), reducing the enzymatic degradation by allosteric-silencing collagenolytic enzymes or by altering the enzyme-binding site in the collagen molecule (Tjaderhane 2015). Different cross-linkers have been recently evaluated (Perdigao et al. 2013; Bedran-Russo et al. 2014). Of particular interest to dental application are the proanthocyanidins, mainly because they are a naturally occurring compound with no cytotoxicity (Bedran-Russo et al. 2014).
The major disadvantage of using cross-linking agents is that the application time needed to achieve the desirable therapeutic effect is not clinically feasible (Castellan et al. 2010, 2011). However, simplified protocols have been developed such as the incorporation of proanthocyanidins into the etchants and adhesive bottles (Green et al. 2010; Epasinghe et al. 2012; Liu et al. 2013, 2014). Unfortunately, the literature lacks sufficient evaluation of these cross-linkers for root canal use. So far, only one study evaluated the effect of proanthocyanidin application before obturation of the root canal with a resin-based sealer, reporting enhanced durability of the root canal and sealer after 3 months of water storage (Kalra et al. 2013).
8.3.3.4 Benzalkonium Chloride (BAC)
The use of aqueous solution of BAC or BAC-containing acid or adhesive may be another simpler approach to inhibit the activity of matrix-bound MMPs (Tezvergil-Mutluay et al. 2011; Sabatini and Patel 2013) as it produced more stable bonds over time for coronal dentin (Sabatini et al. 2015; Sabatini and Pashley 2015). This procedure has not yet been investigated in root dentin, but due to the similarities between coronal and root dentin, it may provide similar beneficial results.
8.4 Self-Adhesive Cements
One recent meta-analysis of laboratory studies indicated that the use of self-adhesive resin cements could improve the retention of fiberglass posts when compared with SE or ER adhesives associated to a conventional resin cement (Sarkis-Onofre et al. 2014). However, most of these studies only evaluated the immediate bonding and refer to only a specific material brand (Radovic et al. 2008
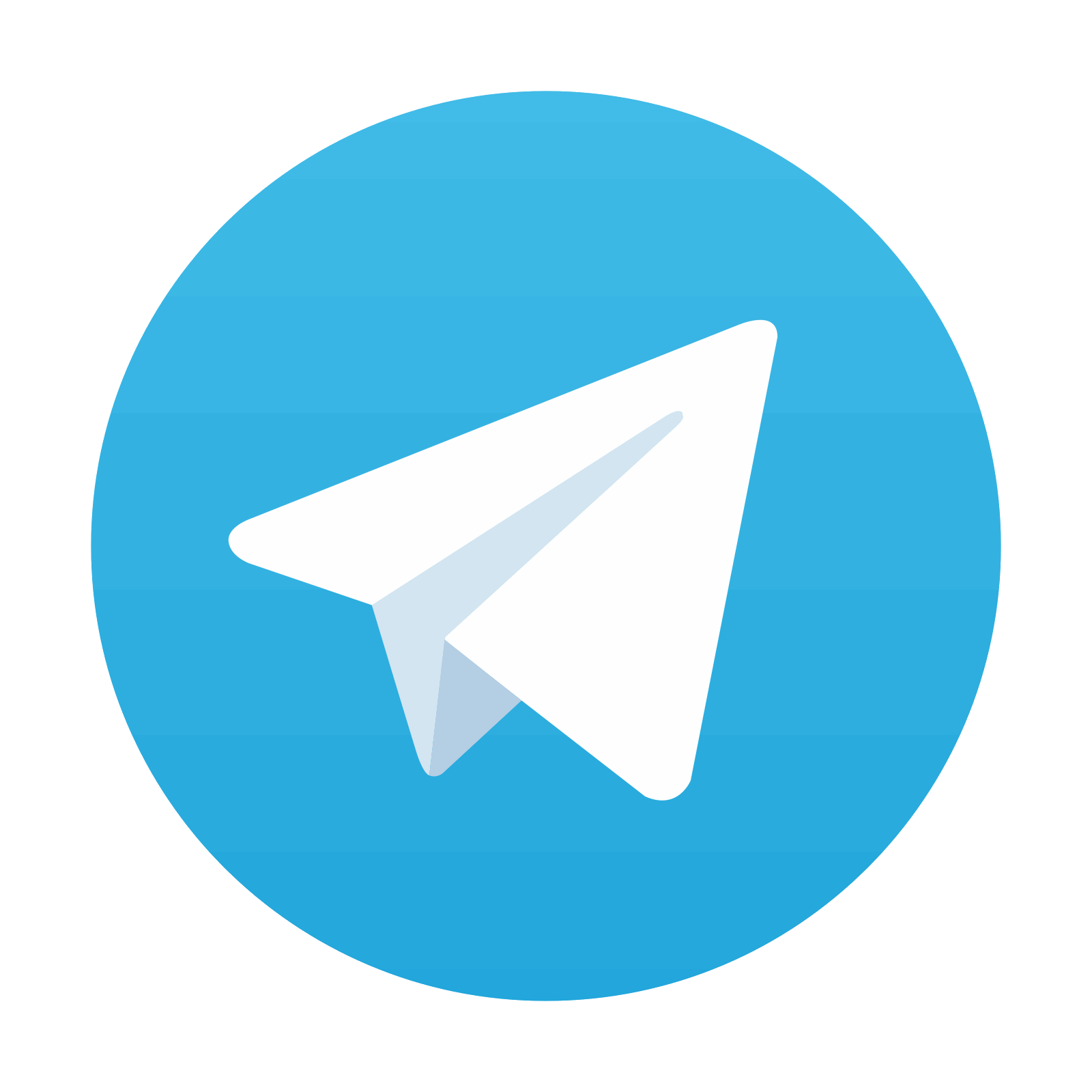
Stay updated, free dental videos. Join our Telegram channel

VIDEdental - Online dental courses
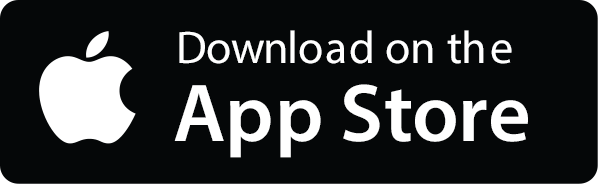
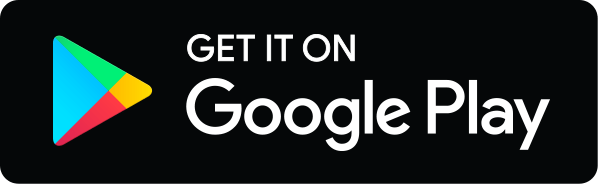