Introduction
Control of root torque is often achieved by introducing a twist in a rectangular archwire. The purpose of this study was to investigate third-order torque on different types of self-ligated brackets by analyzing the bracket’s elastic and plastic deformations in conjunction with the expressed torque at varying angles of twist.
Methods
An orthodontic bracket was mounted to a load cell that measured forces and moments in all directions. The wire was twisted in the bracket via a stepper motor, controlled by custom software. Overhead images were taken by a camera through a microscope and processed by using optical correlation to measure deformation.
Results
At the maximum torquing angle of 63° with 0.019 × 0.025-in stainless steel wire, the total elastic and plastic deformation values were 0.063, 0.033, and 0.137 mm for Damon Q (Ormco, Orange, Calif), In-Ovation R (GAC, Bohemia, NY), and Speed (Strite Industries, Cambridge, Ontario, Canada), respectively. The total plastic deformation values were 0.015, 0.006, and 0.086 mm, respectively, measured at 0° of unloading.
Conclusions
In-Ovation R had the least deformation due to torquing of the 3 investigated bracket types. Damon Q and Speed on average had approximately 2.5 and 14 times greater maximum plastic deformation, respectively, than did In-Ovation R.
Orthodontic treatment involves controlling crown and root positions in all 3 planes of space. Tooth long-axis buccolingual angulations are often referred to as “root torque” and are important in establishing functionally stable occlusal relationships. In fixed edgewise orthodontic treatment mechanics, control of root torque is achieved by the interaction of a rectangular archwire in a rectangular bracket slot. As the archwire is twisted relative to the bracket slot, a moment is introduced. This can be achieved by altering the bracket slot angle relative to the bracket base (bracket prescription) or by introducing a twist along the long axis of the wire (third-order bend). The moment generated by this third-order torque is often called “torque expression” and is measured in Newton millimeters (Nmm).
Torque expression is influenced by the play between the wire and the bracket slot, the material properties of the wire, the ligation method, and the bracket design. “Torque play” is the range of angles at which the torque is approximately zero. “Zero position” is an angle, defined as 0°, near the center of the torque-play region before any plastic deformation. “Angle,” “angle of twist,” and “torquing angle” all refer to the angle of the wire’s rotation in the bracket slot, relative to the set zero position.
As the wire is twisted relative to the bracket slot, stresses are introduced in the wire and bracket, and strain (deformation) of the wire or bracket will occur. “Elastic deformation” is a material’s nonpermanent deformation caused by a load. “Plastic deformation,” which occurs at higher stresses than elastic deformation, is permanent deformation of the material.
Several studies have investigated torque expression among different bracket types, ligation methods, and wire types. A recent numeric analysis by Huang et al evaluated torque expression with various wire-bracket combinations but did not report bracket or wire strain in response to the loading stress. To date, no published studies have evaluated the role of elastic and plastic bracket deformation in torque expression.
The purpose of our study was to investigate third-order torque on different types of self-ligated brackets by analyzing the bracket’s elastic and plastic deformation in conjunction with the expressed torque at varying angles of twist. Since this was an early study meant to develop a basis for future procedures, the maximum of the selected range of angles is beyond the typical clinical range to build a basis for studying the mechanical behavior in orthodontic brackets while subjected to third-order torque. Further studies will be required to identify the loading and unloading curves of various clinically relevant torquing angles.
Material and methods
The experimental system was a modified version of the torque device used by Lacoursiere et al. The device consisted of an orthodontic bracket bonded with Epoxy adhesive (Loctite, E-20HP; Hysol, Henkel, Rocky Hill, Conn) to a mounting system attached to the top of a 6-axis load cell (Nano 17 Multi-Axis force/torque transducer; ATI Industrial Automation, Apex, NC). An assembly consisting of 2 dies, which gripped the orthodontic wire on either side of the bracket at 5 mm from the edge of the bracket to the die, rotated the wire via a worm gear and stepper motor (Cool Muscle CM1-C-11L30; Myostat Motion Control, Newmarket, Ontario, Canada). The torque and force data were collected through a data acquisition card (DAC 16-bit series NI PCI-6033E; National Instruments, Austin, Tex). A high-resolution charge-coupled device camera (piA2400-12 g, 2448 × 2050 pixels, 8 bit, gray scale; Basler Vision Technologies, Exton, Pa) with a microscope (55-908 MMS R4; Edmund Optics, Barrington, NJ) with epi-illumination was mounted above the bracket to take overhead images. The motor control and data logging (both torque-force and image data) were performed with custom software (LabWindows CVI, National Instruments). To improve image contrast and ease of image processing, the top surfaces of the brackets were etched with a microetcher (Arum Group, Spokane, Wash).
We made some modifications to the assembly and procedure of Lacoursiere et al. By using a coordinate measurement system (FaroArm; FARO USA, Lake Mary, Fla), the distance between the load cell and the bracket was measured. A mathematical transformation was applied to the data with a spreadsheet (Microsoft Office Excel 2003; Microsoft, Redmond, Wash) so that the final torsion results were measured at the bracket slot instead of at the load cell. The transformation has the following equation:
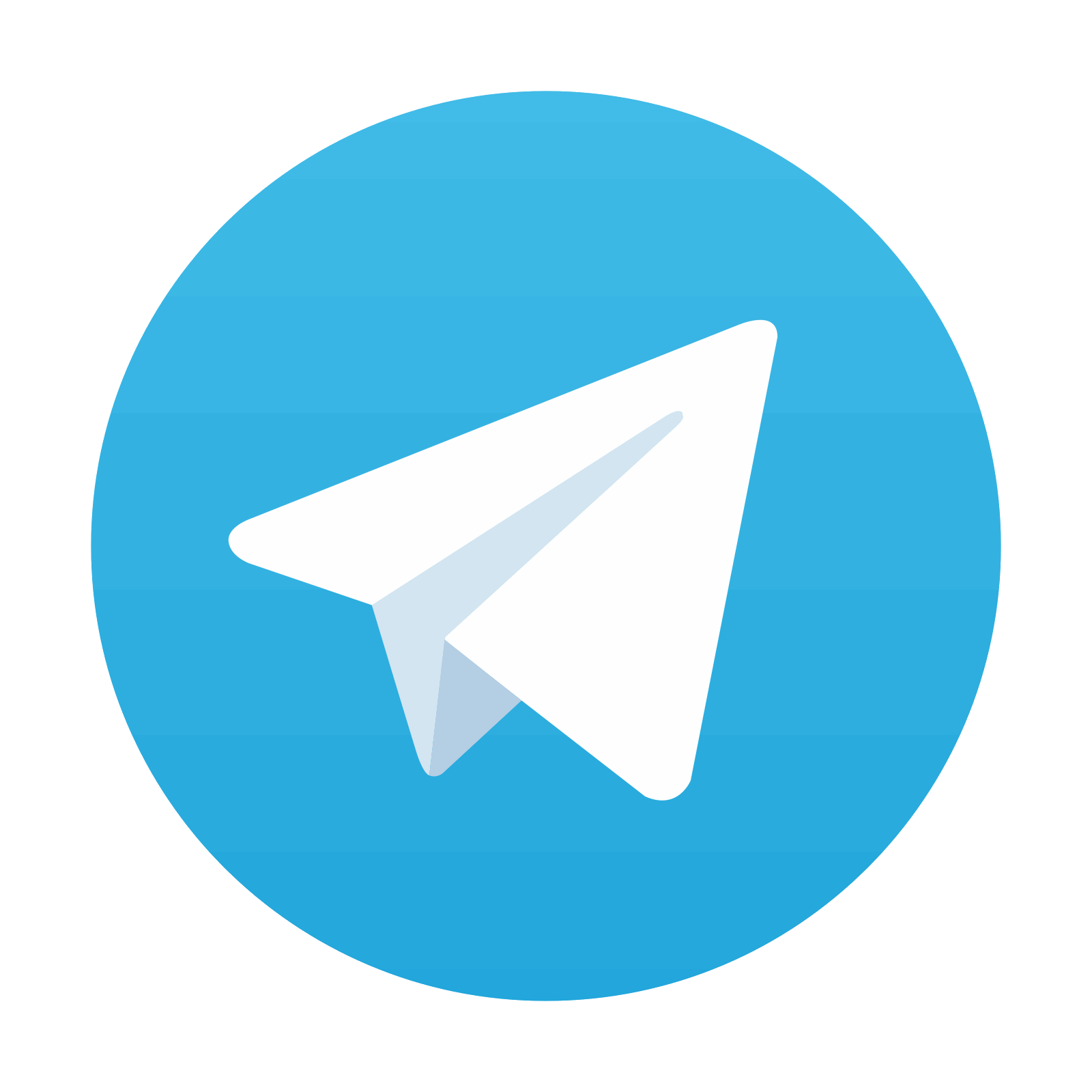
Stay updated, free dental videos. Join our Telegram channel

VIDEdental - Online dental courses
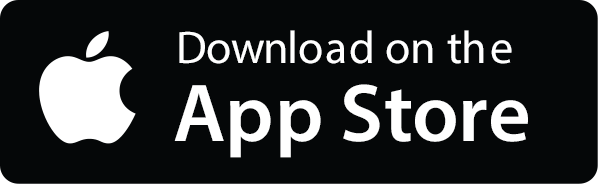
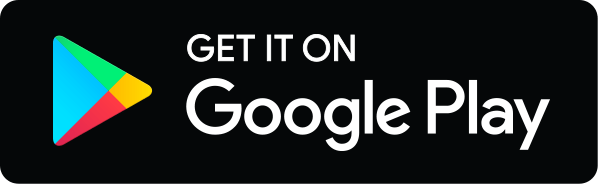