Graphical abstract

Highlights
- •
Magnetic nanofiber scaffolds stimulate growth and odontogenesis of human dental pulp cells.
- •
Magnetic nanofiber scaffolds induce pro-angiogenic effects on endothelial cells.
- •
Wnt/β-catenin, MAPKs, and NF-κB pathways are involved in the stimulatory events.
Abstract
Objective
Magnetic biomaterials have recently gained great attention due to their some intriguing cell and tissue responses. However, little attention has been given to the fields of dental tissue regeneration. In this sense, we aim to investigate the effects of magnetic nanofiber scaffolds on the human dental pulp cell (HDPC) behaviors and to elucidate the underlying signaling mechanisms in the events.
Methods
Magnetic nanofiber scaffolds incorporating magnetic nanoparticles at varying contents were prepared into nanofibrous matrices to cultivate cells. Cell growth by MTS assay, odontoblastic differentiation by alkaline phosphatase (ALP) activity, mineralization, and the mRNA expression of differentiation-related genes of HDPCs, in vitro angiogenesis by migration and capillary tube formation in endothelial cells on the conditioned medium obtained from HDPSCs in the presence or absence of scaffolds. Western blot analysis and confocal immunofluorescene were used to asses signaling pathways.
Results
The growth of HDPCs was significantly enhanced on the magnetic scaffolds with respect to the non-magnetic counterpart. The odontogenic differentiation of cells was significantly up-regulated by the culture with magnetic scaffolds. Furthermore, the magnetic scaffolds promoted the HDPC-induced angiogenesis of endothelial cells. The expression of signaling molecules, Wnt3a, phosphorylated GSK-3β and nuclear β-catenin, was substantially stimulated by the magnetic scaffolds; in parallel, the MAPK and NF-κB were highly activated when cultured on the magnetic nanofiber scaffolds.
Significance
This study is the first to demonstrate that magnetic nanofiber scaffolds stimulate HDPCs in the events of growth, odontogenic differentiation, and pro-angiogenesis, and the findings imply the novel scaffolds can be potentially useful as dentin-pulp regenerative matrices.
1
Introduction
Regenerative endodontics generally involves the treatment of infected root canal systems in combination with apical enlargement to permit revascularization, where the use of cells, growth factors and scaffolds can play significant roles . Scaffolds are the essential component of tissue engineering and provide a suitable environment for stem cells in dental pulp to recapitulate the dentin-pulp generation processes . In general, scaffolds need to satisfy physical (porous three-dimension structure), mechanical (mechanical strength and stiffness), and biological properties (biocompatibility and biodegradation) for the appropriate cellular adhesion, growth, migration and differentiation .
Biodegradable synthetic polymers such as polycaprolactone (PCL), poly(lactic acid) (PLA), poly(glycolic acid) (PGA), and their co-polymer have been widely used to prepare scaffolds for tissue engineering because of their biocompatibility and ability to form desired shapes . Compared to PLA and PGA, PCL is relatively more slowly degradable, more flexible, and less expensive . However, those synthetic polymers lack surface bioactivity thus have initial slow cell responses, and the hydrolytic degraded acidic products often induce inflammation. Therefore, to improve the cell interaction and tissue responses of the scaffolds, bioactive inorganic additives, including hydroxyapatite and bioactive glasses, have been incorporated . Results have demonstrated substantial improvement in the growth and differentiation of cells including osteoblasts, bone marrow stromal cells, and dental pulp cells.
Among the additives, magnetic nanoparticles (MNPs), mainly comprised up of iron oxide magnetite, have recently gained great interest due to their excellent magnetic properties with relatively good biocompatibility . The addition of MNPs to biopolymers has been shown to increase the mechanical properties such as stiffness and strength as well as the biological responses required for bone repair . While the MNPs-incorporated biomaterials have been shown to have high potential for the stimulation of cells involved in the osteogenic process and bone formation due to the magnetic field generated which might alter the local cellular microenvironment , little has been documented for the repair and regeneration of dental tissues including dentin and pulp.
Therefore, we focus our interest in the application of the magnetic scaffolds in dental pulp-dentin regeneration. As a first step to this, we have prepared the magnetic scaffolds in a nanofiber form and examine the growth and odontoblastic differentiation of human dental pulp cells (HDPCs). Furthermore, the pro-angiogenic effects by the magnetic scaffolds are also examined. Lastly, the molecular mechanisms underlying the magnetic scaffold-induced cellular events were investigated. The aim of this study was to evaluate the hypotheses of these studies for the potential of the magnetic nanofiber scaffolds in the dentin-pulp regeneration purposes.
2
Materials and methods
2.1
Fabrication and characterizations of magnetic nanofiber scaffolds (MNS)
As shown in the appendix, the MNPs were first synthesized and functionalized by a citric-acid treatment to anchor carboxylic acid functional group (COOH), after which the MNS were fabricated by electrospinning, according to the previous protocol . Briefly, after ferrous chloride tetrahydrate in 1 M HCl and ferric chloride hexahydratate were mixed at room temperature, the mixture was dropped into NaOH for precipitation. The MNPs were dispersed in citric acid solution for conjugation with COOH, the COOH-MNPs were precipitated by addition of acetone. 10% w/v PCL in DCM-ethanol solution were mixed with the prepared COOH-MNPs (10 or 20 wt%). This mixture was injected through the stainless steel needle connected to a high-voltage power supply (15 kV). All experiments were performed at room temperature.
The micro- and nano-structure of the samples was characterized by scanning electron microscopy (SEM, S-3000H, Hitachi, Japan) and transmission electron microscope (TEM, 7100, JEOL, USA). The crystalline phase was determined by X-ray diffraction (XRD, Danvers, MA, USA). Fourier transformed infrared (FT-IR, Perkin-Elmer) spectroscopy was used to observe the chemical bond status of the samples. The water affinity of the samples was observed by water contact angle using a Phoenix300 analyzer at 25 °C. To investigate apatite forming ability, samples were immersed in 45 ml of (1.5×) simulated body fluid (SBF) with pH 7.4 and appropriate ion concentrations (Na + 142.0, K + 5.0, Mg 2+ 1.5, Ca 2+ 2.5, Cl − 147.8, HCO 3− 4.2, HPO 4 2− 1.0, SO 4 2− 0.5 mM) at 37 °C for 30 days . The magnetic properties of the samples were measured by vibrating sample magnetometer (VSM) in an applied magnetic field of ±20 kOe at room temperature, in terms of saturation magnetization and hysteresis loops . VSM was calibrated using a standard reference (high purity nickel sphere), supplied with the instrument.
2.2
In vitro study
Immortalized HDPCs transfected with human telomerase catalytic component provided by Prof. Takashi Takata was used for in vitro study (Hiroshima University, Japan) . As shown in the appendix, HDPCs grown on the scaffolds were subjected to cell proliferation, ALP activity, Alizarin Red S staining, mRNA expression, in vitro angiogenesis and scratch wound–healing assay, Western blot, and cell double-labeling immunofluorescence assays. The cells were grown in alpha-MEM medium supplemented with 10% fetal bovine serum (FBS), penicillin (100 units/ml), and streptomycin (100 μg/ml) at 37 °C in a humidified atmosphere of 5% CO 2 /95% air. Cells were seeded onto each scaffold (3 × 10 5 cells/6 well plate). To induce odontogenic differentiation, the cells were cultured with odontogenic supplement medium (OS) which containing 50 μg/ml l -ascorbic acid ( l -AA) and 10 mM beta-glycerophosphate (beta-GP). The medium was replaced every three days during the incubation period.
2.3
Cell proliferation
Cell proliferation was measured by a 3-(4,5-dimethylthiazol-2-yl)-5-(3-carboxymethoxyphenyl)-2-(4-sulfophenyl)-2H-tetrazolium (MTS) assay kit (Cell Titer 96 Aqueous One Solution; Promega, Madison, WI) Absorbance was measured at a wavelength of 490 nm using an enzyme linked immunosorbent assay (ELISA) reader (Beckman Coulter, Fullerton, CA).
2.4
ALP activity
ALP activity was measured using p-nitrophenyl phosphate (3 mM final concentration) as the substrate in 0.7 M 2-aminomethyl-1-propanol (pH 10.3) and 6.7 mM MgCl 2 . Briefly, Cells were harvested and lysed in 200 μl. After 50 μl of pNPP solution were added in each well containing 80 μl of lysates, 10 μL of ALP enzyme solution and were incubated at 25 °C for 60 min protected from light. The absorbance was measured at a wavelength of 405 nm using an ELISA reader (Beckman Coulter).
2.5
RNA isolation and reverse transcriptase-polymerase chain reaction (RT-PCR)
The total RNA of cells was extracted with Trizol reagent (Life Technologies, Gaithersburg, MD) according to the manufacturer’s instructions. 1 μg quantity of RNAs isolated from each sample was reverse-transcribed with oligo (dT) 15 primers with AccuPower RT PreMix (iNtRON Biotechnology, Gyeonggi-do, South Korea). Next, the generated cDNAs were amplified with AccuPower PCR PreMix (Bioneer Corporation, Daejeon, South Korea). Primer sequences are listed in Table 1 . To visualize, PCR products were subjected to electrophoresis on 1.5% agarose gels, stained with ethidium bromide. All assays were repeated 3 times to ensure reproducibility.
Genes | Primer Sequence (5’-3’) | Annealing Temp ( °C) | Cycle Number | Product Size (bp) |
---|---|---|---|---|
ALP | F: 5′-GGGACCCAAGAAACCAAAGT-3′ | 55 | 30 | 142 |
R: 5′-TGCCTGCCCAAGAGAAATC-3′ | ||||
OPN | F: 5′-GCTTCTTTCTCAGTTTATTGGTTGA-3′ | 55 | 35 | 111 |
R: 5′ – TTAGTTTACAGGGAGTTTCCATGA-3′ | ||||
DSPP | F: 5′-GCAAAAGTCCAGGACAGTGGGCC-3′ | 55 | 30 | 342 |
R:5′-GTCAAATTTCCACCTCAGTTGGCCA-3′ | ||||
VEGF | F: 5′-CAGGACATTGCTGTGCTTTG-3′ | 50 | 35 | 122 |
R: 5′-CTCAGAAGCAGGTGAGAGTAAG-3′ | ||||
Ang-1 | F: 5′-GATGTCAATGGGGGAGGTTG-3′ | 55 | 35 | 424 |
R: 5′-CATTTAGATTGGAGGGGCCA-3′ | ||||
β-actin | F: 5′-CTGGACTTCGAGCAAGAGATG-3′ | 50 | 30 | 138 |
R: 5′-GAAGGAAGGCTGGAAGAGTG-3′ |
2.6
Alizarin Red S staining
HDPCs were fixed in 70% ice-cold ethanol for 1 h and rinsed with distilled water. Cells were stained with 40 mM Alizarin Red S (pH 4.2) for 10 min with gentle agitation. The level of Alizarin Red S staining was observed under light microscopy.
2.7
In vitro angiogenesis assay
Human umbilical vein endothelial cells (HUVECs) obtained from ATCC (University Blvd. Manassas, VA) were cultured in endothelial cell medium (ECM, ScienCell Research Laboratories, Carlsbad, CA) at 37 °C under a 5% CO 2 atmosphere. ECM Gel solution (Cell Biolabs, San Diego, CA) of 50 ml was poured onto a 96-well culture plate and were allowed to solidify (37 °C, 1 h). HUVECs (1.5 × 10 4 cells/well) were seeded on the ECM gel and were cultured in mixture of CM and endothelial cell medium (5:5) in the absence or presence of scaffolds for three days. After 12 h, tube formation was observed under a light microscope and quantified honeycomb-like tubular structures as capillary formation in three random fields.
2.8
Migration assay
Cell migration was accessed by in vitro scratch assay. HUVECs were seeded (1 × 10 6 ) onto 6-well plates and allowed to grow to 90% confluency in ECM (ScienCell Research Laboratories) at 37 °C under a 5% CO 2 atmosphere. Cell monolayers were wounded by a 200 μl pipette tip and washed three times with 1× PBS to remove cell debris. HUVECs were incubated with CM up to 24 h, and compared the images to quantify the migration rate of the cells. Cell migration viewed under a light microscope and evaluated relative migration distance using Photoshop program. The graph was expressed as percentage of the initial area.
2.9
Western blot analysis
HDPCs were lysed and protein concentration was determined by Bradford reagent (Bio-Rad, Hercules, CA). Equal amounts of lysates were resolved on sodium dodecyl-polyacrylamide gel electrophoresis (SDS–PAGE) were transferred to a polyvinylidene fluoride (PVDF) membrane (Millipore, Bedford, MA), and the membrane was blocked with 1× TBS containing 0.05% Tween 20 and 5% skim milk or 2% BSA for 1 h at room temperature. After blocking, the membranes were incubated overnight at 4 °C with the respective primary antibodies. The membranes were washed thrice and incubated with diluted horseradish peroxidase (HRP)-conjugated secondary antibodies (1:10,000, Jackson ImmunoResearch, West Grove, PA) for 1 h at room temperature. After three washes, the membranes were detected using the enhanced chemiluminescence (ECL) kit (Millipore).
2.10
Confocal immunofluorescence microscopy
HDPCs were washed with 1× PBS, and fixed in 10% formalin for 15 min at room temperature. After washing three times in 1× PBS, the cells were permeabilized with 0.2% Triton X-100 in 1× PBS PBS for 20 min, washed three times in 1× PBS, and then blocked with 5% BSA in 1× PBS for 1 h at room temperature. After then, the cells were incubated with anti-p65 antibody (1:200, Santa Cruz Biotechnology, Santa Cruz, CA) for overnight at room temperature, washed three times, and incubated with Alexa-488 conjugated secondary antibodies (1:500, Invitrogen, Carlsbad, CA) for 2 h at room temperature. The cells were washed three times, mounted on glass slides, and viewed on confocal microscopy (Cell Voyager, Yokohama, Japan).
2.11
Statistical analysis
The data are expressed as mean ± S.D. of at least three independent experiments. Statistical significance was evaluated by one-way analysis of variance using the SPSS (Version 21.0; SPSS, Chicago, IL) computer program. A value of p < 0.05 was considered to be statistically significant.
2
Materials and methods
2.1
Fabrication and characterizations of magnetic nanofiber scaffolds (MNS)
As shown in the appendix, the MNPs were first synthesized and functionalized by a citric-acid treatment to anchor carboxylic acid functional group (COOH), after which the MNS were fabricated by electrospinning, according to the previous protocol . Briefly, after ferrous chloride tetrahydrate in 1 M HCl and ferric chloride hexahydratate were mixed at room temperature, the mixture was dropped into NaOH for precipitation. The MNPs were dispersed in citric acid solution for conjugation with COOH, the COOH-MNPs were precipitated by addition of acetone. 10% w/v PCL in DCM-ethanol solution were mixed with the prepared COOH-MNPs (10 or 20 wt%). This mixture was injected through the stainless steel needle connected to a high-voltage power supply (15 kV). All experiments were performed at room temperature.
The micro- and nano-structure of the samples was characterized by scanning electron microscopy (SEM, S-3000H, Hitachi, Japan) and transmission electron microscope (TEM, 7100, JEOL, USA). The crystalline phase was determined by X-ray diffraction (XRD, Danvers, MA, USA). Fourier transformed infrared (FT-IR, Perkin-Elmer) spectroscopy was used to observe the chemical bond status of the samples. The water affinity of the samples was observed by water contact angle using a Phoenix300 analyzer at 25 °C. To investigate apatite forming ability, samples were immersed in 45 ml of (1.5×) simulated body fluid (SBF) with pH 7.4 and appropriate ion concentrations (Na + 142.0, K + 5.0, Mg 2+ 1.5, Ca 2+ 2.5, Cl − 147.8, HCO 3− 4.2, HPO 4 2− 1.0, SO 4 2− 0.5 mM) at 37 °C for 30 days . The magnetic properties of the samples were measured by vibrating sample magnetometer (VSM) in an applied magnetic field of ±20 kOe at room temperature, in terms of saturation magnetization and hysteresis loops . VSM was calibrated using a standard reference (high purity nickel sphere), supplied with the instrument.
2.2
In vitro study
Immortalized HDPCs transfected with human telomerase catalytic component provided by Prof. Takashi Takata was used for in vitro study (Hiroshima University, Japan) . As shown in the appendix, HDPCs grown on the scaffolds were subjected to cell proliferation, ALP activity, Alizarin Red S staining, mRNA expression, in vitro angiogenesis and scratch wound–healing assay, Western blot, and cell double-labeling immunofluorescence assays. The cells were grown in alpha-MEM medium supplemented with 10% fetal bovine serum (FBS), penicillin (100 units/ml), and streptomycin (100 μg/ml) at 37 °C in a humidified atmosphere of 5% CO 2 /95% air. Cells were seeded onto each scaffold (3 × 10 5 cells/6 well plate). To induce odontogenic differentiation, the cells were cultured with odontogenic supplement medium (OS) which containing 50 μg/ml l -ascorbic acid ( l -AA) and 10 mM beta-glycerophosphate (beta-GP). The medium was replaced every three days during the incubation period.
2.3
Cell proliferation
Cell proliferation was measured by a 3-(4,5-dimethylthiazol-2-yl)-5-(3-carboxymethoxyphenyl)-2-(4-sulfophenyl)-2H-tetrazolium (MTS) assay kit (Cell Titer 96 Aqueous One Solution; Promega, Madison, WI) Absorbance was measured at a wavelength of 490 nm using an enzyme linked immunosorbent assay (ELISA) reader (Beckman Coulter, Fullerton, CA).
2.4
ALP activity
ALP activity was measured using p-nitrophenyl phosphate (3 mM final concentration) as the substrate in 0.7 M 2-aminomethyl-1-propanol (pH 10.3) and 6.7 mM MgCl 2 . Briefly, Cells were harvested and lysed in 200 μl. After 50 μl of pNPP solution were added in each well containing 80 μl of lysates, 10 μL of ALP enzyme solution and were incubated at 25 °C for 60 min protected from light. The absorbance was measured at a wavelength of 405 nm using an ELISA reader (Beckman Coulter).
2.5
RNA isolation and reverse transcriptase-polymerase chain reaction (RT-PCR)
The total RNA of cells was extracted with Trizol reagent (Life Technologies, Gaithersburg, MD) according to the manufacturer’s instructions. 1 μg quantity of RNAs isolated from each sample was reverse-transcribed with oligo (dT) 15 primers with AccuPower RT PreMix (iNtRON Biotechnology, Gyeonggi-do, South Korea). Next, the generated cDNAs were amplified with AccuPower PCR PreMix (Bioneer Corporation, Daejeon, South Korea). Primer sequences are listed in Table 1 . To visualize, PCR products were subjected to electrophoresis on 1.5% agarose gels, stained with ethidium bromide. All assays were repeated 3 times to ensure reproducibility.
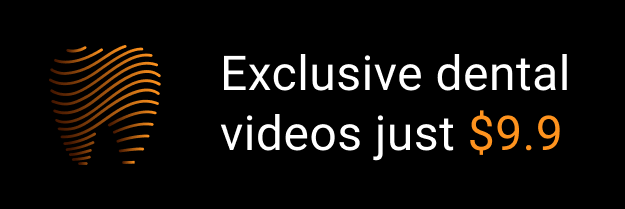