Graphical abstract

Highlights
- •
Role of different roughness components in the healing after implantation is scarce.
- •
Surfaces with similar micronscale roughness might differ in spatial roughness.
- •
The role of different roughness components in osseointegration must be studied.
- •
Surfaces differing in micronscale roughness – not in other parameters – were used.
- •
Surfaces with Ra parameters of about 1–2 μm are optimal for osteblasts.
Abstract
Objectives
Titanium surface roughness is recognized as an important parameter influencing osseointegration. However, studies concerning the effect of well-defined surface topographies of titanium surfaces on osteoblasts have been limited in scope. In the present study we have investigated how Ti surfaces of different micrometer-scale roughness influence proliferation, migration, and differentiation of osteoblasts in-vitro .
Methods
Titanium replicas with surface roughnesses (Ra) of approximately 0, 1, 2, and 4 μm were produced and MG-63 osteoblasts were cultured on these surfaces for up to 5 days. The effect of surface micrometer-scale roughness on proliferation, migration in time-lapse microscopy experiments, as well as the expression of alkaline phosphatase, osteocalcin, vascular-endothelial growth factor (VEGF), osteoprotegerin (OPG), and receptor activator of nuclear factor kappa-B ligand (RANKL) were investigated.
Results
Proliferation of MG-63 cells was found to decrease gradually with increasing surface roughness. However, the highest expression of alkaline phosphatase, osteocalcin and VEGF was observed on surfaces with Ra values of approximately 1 and 2 μm. Further increase in surface roughness resulted in decreased expression of all investigated parameters. The cell migration speed measured in time-lapse microscopy experiments was significantly lower on surfaces with a Ra value of about 4 μm, compared to those with lower roughness. No significant effect of surface roughness on the expression of OPG and RANKL was observed.
Significance
Thus, surfaces with intermediate Ra roughness values of 1–2 μm seem to be optimal for osteoblast differentiation. Neither proliferation nor differentiation of osteoblasts appears to be supported by surfaces with higher or lower Ra values.
1
Introduction
Dental implantation is a safe and long-term approach for restoring edentulous patients . The formation of a bone-implant interface—a process known as osseointegration—is a key requirement for stable implant anchoring and clinical success. Titanium is largely used as a material for dental implants, because it causes no tissue reaction and exhibits the ability to fuse with bone . Promoting the rate and extent of osseointegration immediately after dental implantation is crucial for the success of implant therapies. The modification of titanium surfaces is a key instrument to enhance osseointegration and improve the clinical outcome of implant therapy. Titanium (Ti) implant surface characteristics, such as chemical composition, surface topography, roughness, surface energy, nanostructures as well as coating of Ti implants with different bioactive materials are considered to have a substantial impact on the osseointegration process during initial wound healing after implantation .
The micrometer-scale roughness of Ti surfaces is one of the key parameters influencing osseointegration . The roughness of Ti surfaces can be increased by several methods, such as sand blasting, acid etching, laser etching, and anodic oxidation . Most studies describe surface micrometer-scale roughness in terms of either Ra or Sa parameters, which represent mean arithmetic profile roughness and mean arithmetic 2D roughness, respectively. Nowadays, surfaces with moderate roughness with Sa parameters of about 1–2 μm are widely used in implant dentistry and exhibit improved osseointegration and clinical outcome . Arithmetic roughness, however, does not provide information about spatial characteristics of surface and roughness profiles. These topographical surface features are characterized by parameters such as average width of profile elements ( R Sm ), profile asymmetry parameter surface skewness ( R Sk ), and surface kurtosis describing peaks’ sharpness. These parameters are differently affected by different roughening procedures but their influence on osseointegration is not known. Furthermore, different roughening protocols might influence surface structures not only at the micrometer scale, but also at the nanometer scale. Therefore, further studies that can discriminate the effect of different surface characteristics on osseointegration and specify the relationship between mean arithmetic roughness and osseointegration are required.
Improved osseointegration of Ti surfaces with micro-scale roughness could be related to its positive effect on osteoblasts, which are directly involved in the process of bone formation. Previous in vitro studies show that Ti surfaces with moderate microroughness inhibit proliferation and promote differentiation of osteoblasts . This effect of surface microroughness on osteoblasts seems to be associated with activation of α2β1 integrin signaling in osteoblasts . Nevertheless, the effect of a large range of micro-scale roughness values on osteoblast behavior still remains to be investigated. Most previous studies have compared two Ti surfaces with defined microscale roughness values, whereas studies with controlled micrometer-scale roughness are rather rare. Notably, Kunzler et al. showed, in a study with titanium-coated microroughness gradient replicas with Ra values in the range 0–4 μm, that the proliferation of rat calvarial osteoblasts increases with increasing surface roughness . Another study with controlled microroughness shows that osteogenic differentiation of bone marrow mesenchymal stem cells is promoted by surfaces with Ra values of about 2–3 μm . A relationship between surface micrometer-scale roughness and osteoblast differentiation is investigated in two studies with controversial results: one study suggests an improved osteoblast differentiation with an increased surface roughness ; the other study suggests a biphasic relationship, where surfaces with moderate roughness better support osteoblast differentiation compared to very smooth or very rough surfaces . Therefore, additional studies on the relationship between the controlled micrometer-scale roughness and osteoblast response would be highly desirable. Therefore, in the present study, we have investigated the behavior, proliferation, and differentiation of osteoblast-like MG-63 cells grown on Ti-coated replicas with Ra parameters of about 0, 1, 2, and 4 μm. The workflow of the present study is shown in Fig. 1 .

2
Material and methods
2.1
Production of Ti surfaces with different micrometer-scale roughness values
2.1.1
Micrometer-featured roughness masters
Micrometer-featured masters with different roughness values were prepared in a similar way as described previously . A two-step process was used to create micrometer-scale-featured roughness masters. Rolled aluminum sheets (purity 99.5%, dimensions: 20 mm × 40 mm × 2 mm (Metall Service Menzikon AG, Switzerland)) were sand-blasted with corundum particles (81500-826-074, Sablux, Switzerland) to achieve a uniformly rough surface morphology. In a second step, the roughened substrate was fully immersed into a chemical polishing solution (77.5% (v/v) phosphoric acid, 16.5% (v/v) sulfuric acid and 6% (v/v) nitric acid), which preferentially removes small and sharp features as a function of time. The substrates were exposed to the chemical polishing solution for 33, 12 or 2 min. The different polishing times result in homogenous roughness masters with a Ra value of approximately 1, 2 or 4 μm, respectively (referred as surfaces 1 , 2 and 4 ). A flat silicon wafer (〈1 0 0〉 orientation, Si-mat, Germany) was also used as a master with an Ra value of approximately 0 μm (referred as surface 0 ).
2.1.2
Replication
In order to produce multiple identical samples for reproducibility reasons, the masters were replicated, as described by Wieland et al. and Schuler et al. . Impressions of the different roughness masters were prepared in polyvinylsiloxane (PROVIL novo light, Heraeus-Kulzer, Switzerland). A 24-well plate (TPP, Switzerland) was used as a mold to achieve cylindrical negatives with a diameter of 16.2 mm. Negative replicas were inserted into a polytetrafluoroethylene (PTFE) template with holes of a diameter of 15 mm, leaving a rim of 1 mm height. Epoxy resin (EPO-TEK 302-3M, Polyscience AG, Switzerland) was cast onto the polyvinylsiloxane negatives and cured at 65 °C for at least 24 h. The epoxy samples were cleaned for 10 min in an ultrasonic bath containing a 2 vol% Hellmanex solution (HELLMA GmbH & Co, Müllheim, Germany) and extensively rinsed in ultra-pure water 10 times. Samples were pre-dried with a jet of nitrogen and air dried overnight. Clean epoxy replicas were sputter-coated with 40 nm of metallic titanium (Reactive magnetron sputtering, Paul Scherrer Institute, Villigen, Switzerland). SEM investigation of freeze-fractured cross-sections of epoxy samples with highest roughness (Ra ∼ 4 μm) showed that the epoxy surface is entirely covered with a 30–50 nm thick layer of TiO 2 . No tendency of a thinner coating on peaks and thicker coating in valleys could be observed (see also exemplary images in Supplementary material). The replicas were cleaned in a plasma cleaner (Harrick, PDC-32G, USA) for 2 min in an oxygen atmosphere of roughly 2 × 10 −3 mbar at high RF level. The replicas were sterile packed under laminar flow in containers containing ultra-pure water and stored at room temperature until further use.
2.1.3
Characterization
The micrometer-scale roughness of the titanium-coated epoxy replicas was analyzed by an optical profilometer (Sensofar Plu Neox, Sensofar, Spain) in confocal mode. 2.75 mm long strips were stitched together by using the 20× objective. The evaluation was carried out with the SensoMap software (v.6.0, Sensofar, Spain) according to the DIN EN ISO 4287 standard.
The contribution of different wavelength windows to the overall surface roughness was determined using a fast-Fourier-transformation (FFT)-based analysis method . With this method, contributions in different wavelength windows can be individually evaluated. Three different windows with wavelengths between 3–10 μm, 10–50 μm and 50–250 μm were used to calculate the arithmetic roughness value Ra.
2.2
Cell culture
Osteoblast-like MG-63 cells (American Type Culture Collection, Rockville, USA) were used in the present study. MG-63 is a cell line that exhibits osteoblastic features and has constant properties , whereas characteristics of primary osteoblasts may differ depending on the source, donor, and isolation method . The cells were cultured in modified Eagle’s minimum essential medium (MEM, Gibco ® , Carlsbad, USA) supplemented with 10% fetal bovine serum, penicillin (100 U/ml) and streptomycin (50 μg/ml) at 37 °C in a humidified atmosphere containing 5% CO 2 . Cells were grown in culture up to passage four, in order to obtain sufficient cell numbers for experiments. Cells between the fourth and the seventh passage were used in the experiments.
2.3
Measurements of cell proliferation and viability
Cell proliferation was measured by two methods: manual cell counting and cell counting kit 8 (CCK-8, Dojindo Laboratories, Japan). In proliferation experiments, 2 × 10 4 cells were seeded on each Ti-coated (diameter 15 mm, cylinder height 1 mm) surface in 500 μl MEM. Cells seeded at similar density on tissue culture plastic (TCP) were used as control. Each group included three different wells. Cell proliferation was measured after 2, 5, and 9 days of culture. For manual cell counting, cells were collected by accutase and counted in blind fashion using a cell-counting chamber under a microscope. Cell viability/proliferation was determined using the cell counting kit-8 (CCK-8; Dojindo Laboratories, Japan) assay. After incubation, 50 μl of CCK-8 reagent were added into each well and culture plates were incubated in 5% CO 2 at 37 °C for 4 h. Thereafter 100 μl of each well were transferred to a separate 96-well plate and the optical density (OD) was measured at 450 nm using a microplate reader (Spectramax Plus 384; Molecular Devices, USA).
2.4
Time-lapse microscopy
The behavior of MG-63 cells on different surfaces was observed using time-lapse microscopy, as described in our previous studies . Cells were stained with CellTracker™ Orange CMRA (Molecular Probes™, Invitrogen, UK) according to manufacturer’s instructions and 1 × 10 5 stained cells, resuspended in 800 μl of MEM, were seeded on Ti discs with different surface treatments. Experiments for each surface were repeated at least three times. Cells were observed with an upright fluorescence microscope (Nikon Eclipse E 800 M microscope; Nikon Instruments Europe B.V, Badhoevedorp, Netherlands), and photographed with a digital imaging system (Photometrics1, Cascade 512F, Germany) every 30 min for over 120 h with the aid of NIS-elements software (Nikon Instruments). Cell motility was analysed using the tracking module in manual tracking mode. For each experiment, 10 randomly selected cells per well/group were tracked in the time period from 6 h until 12 h after seeding. Cell motility was described by average migration speed.
2.5
Alkaline phosphatase activity
MG-63 cells were seeded at an initial density of 5 × 10 4 cells per well in 0.5 ml of MEM on Ti surfaces with different micrometer-scale roughness values, as well as on tissue-culture plastic. After 2, 5, or 9 days of culture, the activity of alkaline phosphatase was measured with a similar method to that described in our previous studies . In brief, culture cells were collected by accutase and lysed in 200 μl phosphate-buffered saline (PBS) containing 0.2% Triton X-100, which facilitates the destruction of the plasma membranes, and then homogenized by sonification. The total protein content in cell homogenates was determined using a commercially available kit (Micro/Macro BCA; Pierce Chemical Co., Rockford, IL, USA). The ALP activity was assayed using the conversion of a colorless p -nitrophenyl phosphate to a colored p -nitrophenol according to the manufacturer’s protocol (Sigma, St. Louis, MO, USA). The color changes were measured spectrophotometrically at 405 nm. The amounts of released phosphate were quantified by comparison with a standard curve and normalized to the total protein content in cell homogenates. ALP activity experiments were repeated three times.
2.6
Real-time PCR
The expression levels of different osteogenesis-related proteins in MG-63 cells were quantified by means of real time PCR, applying a similar method to those described earlier . MG-63 cells were seeded at a density of 5 × 10 4 cells per well in 0.5 ml MEM and cultured for 2, 5, or 9 days. Isolation of mRNA and transcription into cDNA was performed using the TaqMan ® Gene Expression Cells-to-CT™ kit (Ambion/Applied Biosystems, Foster City, CA, USA) according to the manufacturer’s instructions. This kit provides high accuracy and superior sensitivity in gene-expression analysis . qPCR was performed on an ABI StepOnePlus device (Applied Biosystems) in paired reactions using TaqMan ® gene expression assays with the following ID numbers (all from Applied Biosystems): alkaline phosphatase (ALP), Hs01029141_g1; osteocalcin (OC), Hs00609452_g1; VEGF, Hs00900055_m1; Hs; β-actin, Hs99999903_m1. β-actin was used as a house-keeping gene. The PCR reactions were performed in triplicate under the following thermocycling conditions: 95 °C for 10 min; 40 cycles, each for 15 s at 95 °C and at 60 °C for 1 min. The point at which the PCR product was first detected above a fixed threshold (cycle threshold, C t ), was determined for each sample. Changes in the expression of target genes were calculated using the 2 −ΔΔCt method, where <SPAN role=presentation tabIndex=0 id=MathJax-Element-1-Frame class=MathJax style="POSITION: relative" data-mathml='ΔΔCt=(Cttarget−Ctβ-actin)sample−(Cttarget−Ctβ-actin)control’>ΔΔCt=(Cttarget−Ctβ-actin)sample−(Cttarget−Ctβ-actin)controlΔΔCt=(Cttarget−Ctβ-actin)sample−(Cttarget−Ctβ-actin)control
Δ Δ C t = ( C t t a r g e t − C t β -actin ) s a m p l e − ( C t t a r g e t − C t β -actin ) c o n t r o l
, taking MG-63 cells grown on plastic as a control.
2.7
Measurement of osteocalcin, and VEGF in conditioned medium
Commercially available ELISA kits were used for osteocalcin (Ebioscience, San Diego, USA) and VEGF (BosterBio, Pleasanton, USA) assays in the conditioned media after 5 days of culturing. Undiluted samples were used for measurements.
2.8
Statistical analysis
The normal distribution of all data was tested with the Kolmogorov–Smirnov test. For normally distributed data, the statistical differences between different groups were analysed by one-way analysis of variance (ANOVA) followed by a post-hoc LSD test. Data are expressed as mean ± S.E.M. Differences were considered to be statistically significant at p < 0.05.
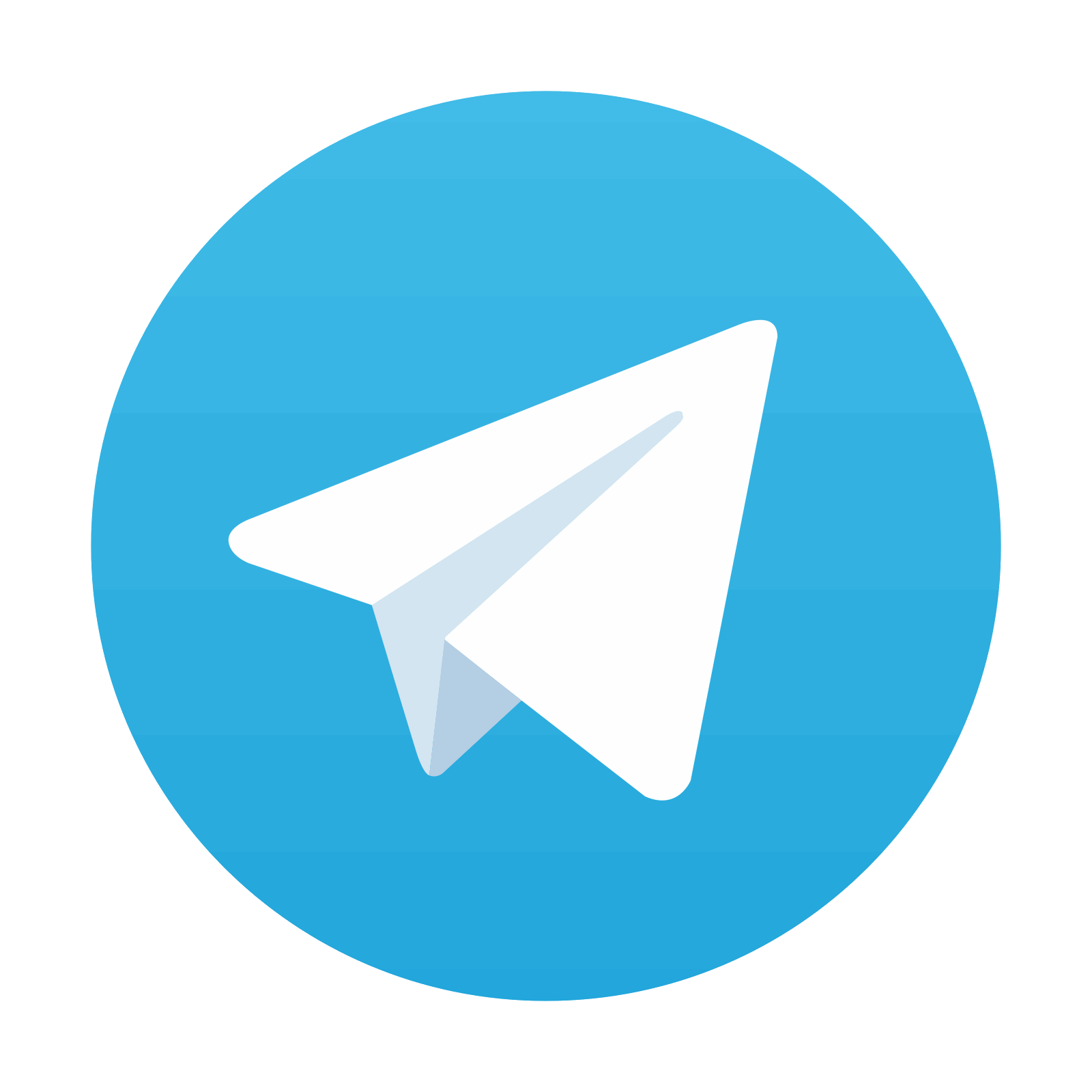
Stay updated, free dental videos. Join our Telegram channel

VIDEdental - Online dental courses
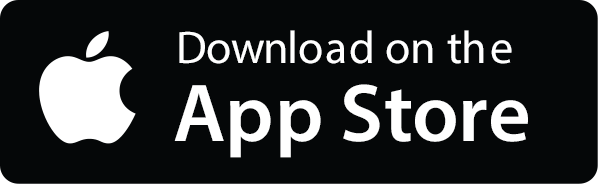
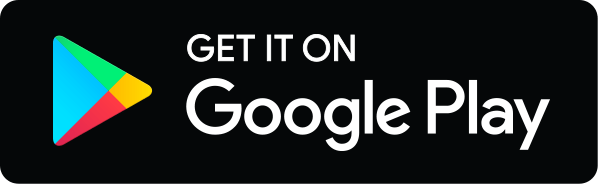