• Figure 2-1 Properties of electromagnetic waves. Amplitude is the height of the wave from the zero axis to the peak. Wavelength is the horizontal distance between two adjacent parts of a wave.
Amplification
Amplification is the part of this process that occurs inside the laser. In this section, the components of a laser instrument are identified to show how laser light is produced.
The center of the laser is called the laser cavity. The following three components make up the laser cavity:
• Active medium
• Pumping mechanism
• Optical resonator
The active medium is composed of chemical elements, molecules, or compounds. Lasers are generically named for the material of the active medium, which can be (1) a container of gas, such as a canister of carbon dioxide (CO2) gas in a CO2 laser; (2) a solid crystal, such as that in an erbium-doped YAG (Er:YAG) laser; (3) a solid-state semiconductor, such as the semiconductors found in diode lasers; or (4) a liquid, such as that used in some medical laser devices.
Surrounding this active medium is an excitation source, such as a flash lamp strobe device, electrical circuit, electrical coil, or similar source of energy that pumps energy into the active medium. When this pumping mechanism drives energy into the active medium, the electrons in the outermost shell of the active medium’s atoms absorb the energy. These electrons have absorbed a specific amount of energy to reach the next shell farther from the nucleus, which is at a higher energy level. A “population inversion” occurs when more of the electrons from the active medium are in the higher energy level shell farther from the nucleus than are in the ground state (Figure 2-2). The electrons in this excited state then return to their resting state and emit that energy in a form known as a photon (Figure 2-3). This is called spontaneous (not stimulated) emission (Figure 2-4).
Completing the laser cavity are two mirrors, one at each end of the optical cavity, placed parallel to each other; or in the case of a semiconductor diode laser, two polished surfaces at each end. These mirrors or polished surfaces act as optical resonators, reflecting the waves back and forth, and help to collimate and amplify the developing beam. A cooling system, focusing lenses, and other controlling mechanisms complete the mechanical components. Figure 2-5 shows a schematic of a gas or solid active-medium laser (e.g., CO2, Nd:YAG). Figure 2-6 shows a schematic of a semiconductor diode device.
Stimulated Emission
Stimulated emission is the process by which laser beams are produced inside the laser cavity. The theory of stimulated emission was postulated by Albert Einstein in 1916.3 He based his work on some earlier work by physicists from Germany (Max Planck) and Denmark (Niels Bohr), who theorized a model of the atom as well as the quantum theory of physics, which defined a quantum as the smallest unit of energy emitted from an atom.4,5 Einstein, using this concept, further theorized that an additional quantum of energy may be absorbed by the already-energized atom, resulting in a release of two quanta (Figure 2-7). This energy is emitted, or radiated, as identical photons, traveling as a coherent wave. These photons in turn are then able to energize more atoms in a geometric progression, which further causes the emission of additional identical photons, resulting in an amplification of the light energy—thereby producing a laser beam (Figure 2-8).
Radiation
The light waves produced by the laser are a specific form of electromagnetic energy.6 The electromagnetic spectrum is the entire collection of wave energy, ranging from gamma rays, with wavelengths of 1 x 10−12 m, to radio waves, with wavelengths of thousands of meters. All currently available dental laser devices have emission wavelengths of approximately 500 to 10,600 nm, which places them in either the visible or the invisible (infrared) nonionizing portion of the electromagnetic spectrum, as shown in Figure 2-9. Of note, the dividing line between the ionizing, cellular DNA–mutagenic portion of the spectrum and the nonionizing portion is at the junction of ultraviolet and visible-violet light. Thus all current dental lasers emit either a visible-light wavelength or an invisible, infrared-light wavelength in the portion of the nonionizing spectrum called thermal radiation.7 The word radiation in this context does not imply radioactive or carcinogenic but simply means the emission of electromagnetic energy.
The following four dental laser instruments emit visible light:
• Argon laser: blue wavelength of 488 nm
• Argon laser: blue-green wavelength of 514 nm
• Frequency-doubled Nd:YAG laser, also called a potassium titanyl phosphate (KTP) laser: green wavelength of 532 nm
• Low-level lasers: red nonsurgical wavelengths of 600 to 635 nm (for photobiomodulation) and 655 nm (for caries detection)
Argon lasers are no longer manufactured as dental surgical instruments, although they are still used for medical procedures.
Other dental lasers emit invisible laser light in the near, middle, and far infrared portion of the electromagnetic spectrum. These include photobiomodulation devices with wavelengths between 800 and 900 nm, as well as surgical instruments, as follows:
• Diode lasers: various wavelengths between 800 and 1064 nm, using a semiconductor active medium of gallium and arsenide, with the addition of either aluminum or indium in some devices
• Nd:YAG laser: 1064 nm
• Erbium-chromium–doped yttrium-scandium-gallium-garnet (Er,Cr:YSGG) laser: 2780 nm
• Er:YAG laser: 2940 nm
• CO2 laser: 9300 nm and 10,600 nm
Laser Delivery Systems
Laser energy should be delivered to the surgical site by a method that is ergonomic and precise.8 Shorter-wavelength instruments, such as KTP, diode, and Nd:Y-AG lasers, have small, flexible fiberoptic systems with bare glass fibers that deliver the laser energy to the target tissue (Figure 2-10). Because the erbium and CO2 laser wavelengths are absorbed by water, which is a major component of conventional glass fibers, these wavelengths cannot pass through these fibers. Erbium and CO2 devices are therefore constructed with special fibers capable of transmitting the wavelengths, with semiflexible hollow waveguides, or with articulated arms (Figure 2-11). Some of these systems employ small quartz or sapphire tips that attach to the laser device for contact with target tissue; others employ noncontact tips (Figure 2-12). In addition, the erbium lasers incorporate a water spray for cooling hard tissues. Lasers may have different fiber diameters, handpieces, and tips (Figure 2-13). Each of these elements plays a significant role in the delivery of energy (Figure 2-14).
All conventional dental instrumentation, either hand or rotary, must physically touch the tissue being treated, which gives the operator instant feedback. As mentioned, dental lasers can be used either in contact or out of contact. The fiber tip can easily be inserted into a periodontal pocket to remove small amounts of granulomatous tissue or treat an aphthous ulcer (Figures 2-15 to 2-17). In noncontact use, the beam is aimed at the target some distance away (Figure 2-18). This modality is useful for following various tissue contours, but with the loss of tactile sensation, the surgeon must pay close attention to the tissue interaction with the laser energy. All of the invisible-light dental lasers—Nd:YAG, CO2, diode, and erbium—are equipped with a separate aiming beam, which can be either a laser or a conventional light. The aiming beam is delivered coaxially along the fiber or waveguide and shows the operator the exact spot at which the laser energy will strike the tissue.
Spot Size
Lenses focus the active beam. With hollow-waveguide or articulated-arm delivery systems, there is a precise spot at the point where the amount of energy is the greatest. This focal point is used for incision and excision surgery. For fiberoptic contact delivery systems, the focal point is at or near the tip of the fiber, which again has the greatest amount of energy. For CO2 lasers, which are used out of contact, the focal point may be anywhere from 1 to 12 mm from the tissue surface, depending on the handpiece being used (Figure 2-19). When the handpiece is moved away from the tissue and away from the focal point, the beam is defocused (out of focus) and becomes more divergent and therefore delivers less energy to the surgical site (Figure 2-20). At a small divergent distance, the beam can cover a wider area, which would be useful in achieving a wide yet shallow ablation of tissue or, at a more divergent distance, hemostasis. At a greater distance, the beam will lose its effectiveness because the energy will dissipate.
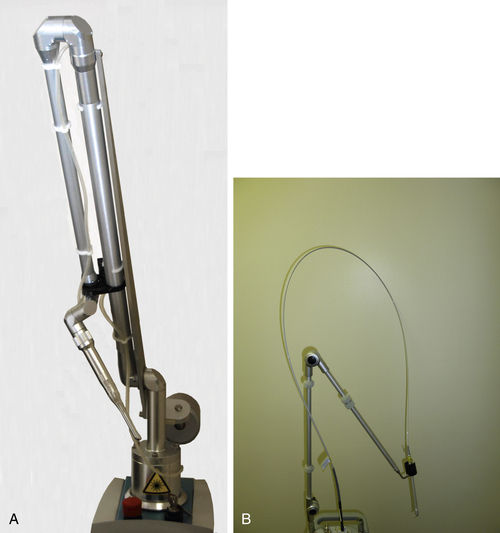
• Figure 2-11 A, Articulated-arm delivery system, typical of CO2 lasers and some erbium devices. B, Hollow waveguide delivery system of a CO2 laser.
Emission Modes
Dental laser devices can emit light energy in two modalities as a function of time: (1) constant on or (2) pulsed on and off.8 The pulsed lasers can be further divided into gated and free-running modes for delivering energy to the target tissue. Thus three different emission modes are described, as follows:
1. Continuous-wave mode, in which the beam is emitted at only one power level for as long as the operator depresses the foot switch.
2. Gated-pulse mode, characterized by periodic alternations of the laser energy, similar to a blinking light. This mode is achieved by the opening and closing of a mechanical shutter in front of the beam path of a continuous-wave emission. All surgical devices that operate in continuous-wave mode have this gated-pulse feature. Some instruments can produce pulses as short as microseconds (μsec) or milliseconds (msec). Peak powers of approximately 10 to 50 times that of continuous-wave power measurements are produced, and charring of the tissue can be reduced. The more advanced units have computer-controlled shutters that allow for these very short pulses. Manufacturers have coined many terms to describe these short pulse durations, including “super pulse” and “ultra speed.”
3. Free-running pulsed mode, sometimes referred to as true pulsed mode. This emission is unique in that large peak energies of laser light are emitted usually for microseconds, followed by a relatively long time in which the laser is off. For example, with a free-running pulsed laser with pulse duration of 100 μsec and pulses delivered at 10 per second (10 Hz), the energy at the surgical site is present for 0.01% of a second and absent for the remaining 99.99% of that second. Free-running pulsed devices have a rapidly strobing flash lamp that pumps the active medium. With each pulse, high peak powers in hundreds or thousands of watts are generated. Because the pulse duration is short, however, the average power that the tissue incurs is small. Free-running pulsed devices cannot have a continuous-wave or gated-pulse output.
True pulsed lasers are driven by the action of the pumping mechanism within the laser cavity. Gated-pulse lasers are pulsed as a result of a shutter outside the laser cavity. Medical and scientific laser instruments are available with pulse durations in the nanosecond (one billionth of a second), picosecond (one trillionth of a second), or smaller range. These can generate tremendous peak powers, but the calculated average pulse energies are small, allowing increased surgical precision. Some instruments can be controlled to emit a single pulse.
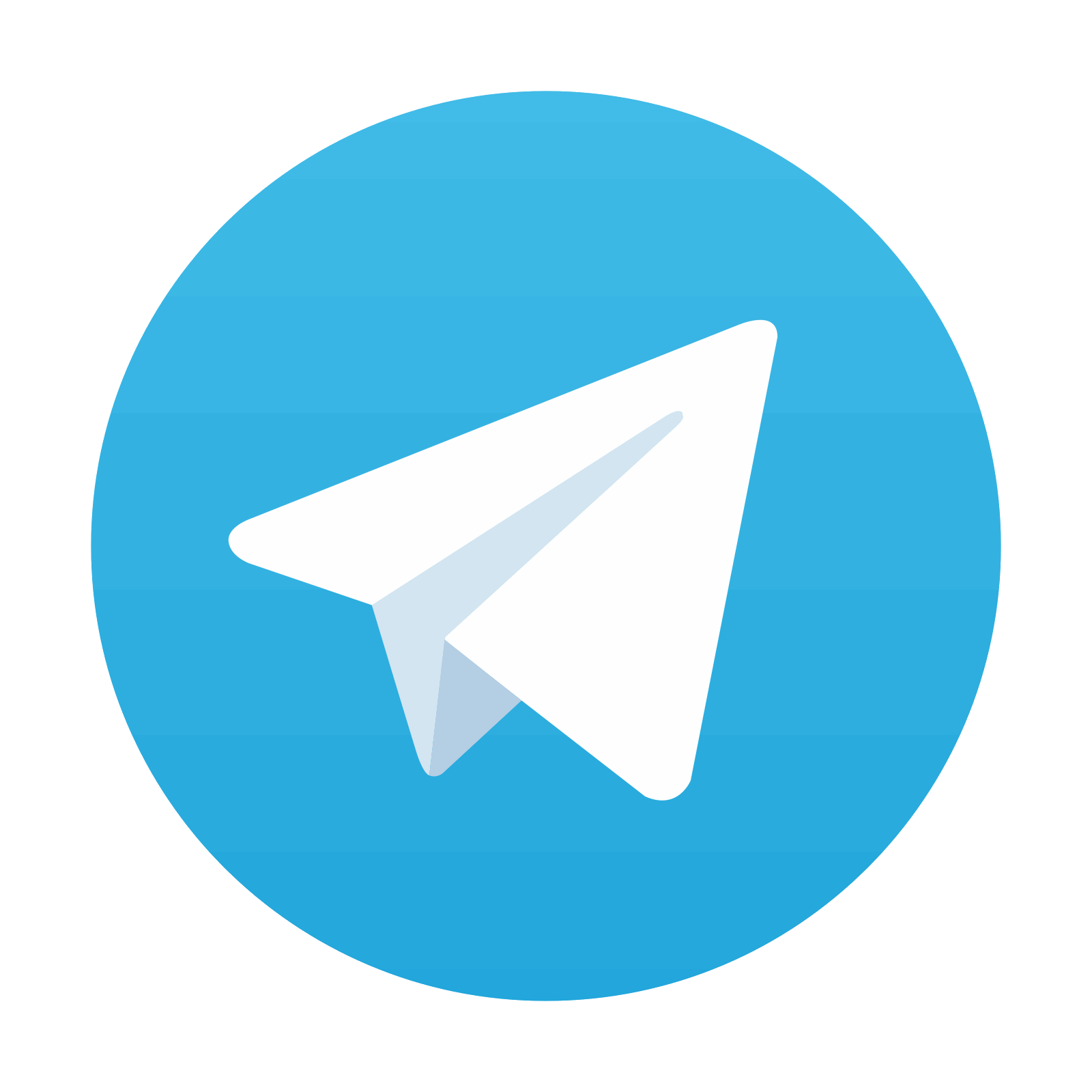
Stay updated, free dental videos. Join our Telegram channel

VIDEdental - Online dental courses
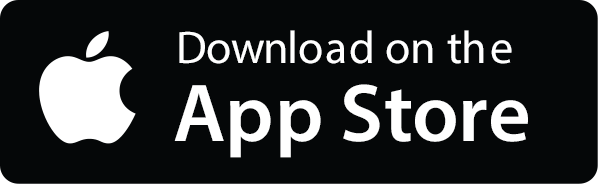
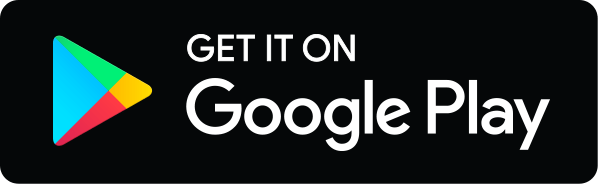