Fig. 16.1
Biofilm is the main form that bacteria are found in endodontic infections. (a) Bacterial biofilm covering the entire perimeter of the canal in cross section. (b) Higher magnification showing the biofilm attached to the canal walls. An accumulation of polymorphonuclear neutrophils can be seen close to the biofilm. These defense cells are usually ineffective in eliminating the endodontic biofilm (Taylor’s modified Brown & Brenn staining, courtesy of Dr. Domenico Ricucci)
The main steps of endodontic treatment involved with infection control are represented by chemomechanical preparation and intracanal medication. Chemomechanical preparation is of paramount importance for root canal disinfection, since instruments and irrigants act primarily in the main canal, which is the most voluminous area of the system and consequently harbors the largest bacterial density. Bacterial elimination from the root canal is carried out by means of the mechanical action of instruments and irrigation as well as the chemical (antibacterial) action of the irrigant solutions. Although substantial amounts of bacteria are eliminated by chemomechanical preparation, studies have demonstrated that 40–60 % of the root canals still present detectable levels of bacteria after instrumentation using either NaOCl or chlorhexidine as the irrigant [18, 32–44]. Because treatment outcome is significantly improved in the absence of detectable bacteria at the time of filling [18, 45–49], some additional step is apparently necessary to optimize disinfection. Several approaches have been proposed to improve disinfection after the instrumentation/irrigation phase [50], including single-visit strategies, but predictably enhanced results have been obtained mostly after an interappointment antimicrobial medication is used.
Intracanal Medication to Supplement Disinfection
Bacteria that escape from the effects of chemomechanical procedures are usually located in areas not reached by instruments and irrigants [7, 24, 26, 51, 52]. These unaffected areas include root canal walls untouched by instruments, dentinal tubules, isthmuses, lateral canals, and apical ramifications [2, 24–26, 31, 53–55].
Irrigants such as NaOCl and chlorhexidine have excellent antimicrobial activities, with pronounced and rapid effects against a large spectrum of species commonly found in endodontic infections. However, these effects are mostly observed when the contact area with the microbial cells is optimal. In the clinical setting, the irrigant should diffuse to reach the areas mentioned above, but the short time they remain in the canal during preparation represents a major limitation. Whereas the irrigant remains in the canal for 10–30 min, which is the usual time taken for preparing most canals, the intracanal medication will remain in the canal for 7 days. This substantial difference in time is the main reason why intracanal medication can enhance disinfection. Because the intracanal medication remains in the canal longer than the irrigation solution, it has more time to diffuse, reach, and eliminate bacteria in areas not affected by chemomechanical procedures.
Numerous substances have been proposed as intracanal medication over the years. The most commonly used substance is calcium hydroxide, so it will be the main focus of this chapter.
Calcium Hydroxide
Calcium hydroxide is possibly the most commonly used intracanal medication. It was introduced in dentistry in 1920 by Bernhard Hermann [56], a German dentist, and since then it has been widely used, especially in endodontics and dental traumatology, for diverse therapeutic purposes [57, 58].
Calcium hydroxide is an inorganic compound, with the formula Ca(OH)2 and molecular weight 74.1 g/mol. The most common presentation is an odorless white powder, obtained by mixing calcium oxide with water:

Under laboratory conditions, it can also be prepared by mixing aqueous solutions of calcium chloride and sodium hydroxide. Calcium hydroxide is a strong base, with a pH of approximately 12.4. Solubility in water is approximately 1.7 g/L at 20 °C. It is soluble in glycerol and insoluble in alcohol.
In the presence of water, calcium hydroxide dissociates into hydroxyl and calcium ions and most of the biological effects attributed to this substance are related to its alkaline pH (hydroxyl ions) [59]. In the presence of carbon dioxide, calcium carbonate is generated through the following process:

Formation of calcium carbonate negatively affects the activities of calcium hydroxide and should be avoided by preventing contact of this substance with air during storage. Calcium hydroxide should be stored at room temperature.
Vehicles for Calcium Hydroxide
Pure calcium hydroxide is available as a powder. Although some clinicians have developed strategies to apply calcium hydroxide powder in the canal, it is undeniable that mixing this substance with a liquid, gel, or creamy carrier (or vehicle) makes application easier and more predictable. Because calcium hydroxide effects are pH-dependent, the ideal vehicle should enable the ionic dissociation of calcium hydroxide. Dissociation will vary according to the type of vehicle used. Because of that, vehicles have been classified according to its consistency and ability to permit calcium hydroxide dissociation as aqueous, viscous, and oily (Table 16.1) [57]. Actually, it is questionable if viscous or oily vehicles are of any value, since they do not permit a high dissociation and consequent release of hydroxyl ions, which is responsible for the main biological effects of calcium hydroxide. Because these effects depend on the magnitude of pH reached in the vicinities where calcium hydroxide was applied, a slow release of calcium hydroxide may not be sufficient to exert the desired effects.
Table 16.1
Classification of the vehicles for calcium hydroxide
Classification according to the consistency and solubility
|
Classification according to the antimicrobial behavior
|
|||
---|---|---|---|---|
Aqueous
|
Viscous
|
Oily
|
Inert
|
Biologically active
|
Distilled water
Saline
Dental anesthetics
Ringer’s solution
Anionic detergent solution
|
Glycerin
Polyethylene glycol
Propylene glycol
|
CPMC
Olive oil
Silicone oil
|
Saline
Distilled water
Dental anesthetics
Glycerin
Propylene glycol
Polyethylene glycol
|
CPMC
Chlorhexidine
Iodine potassium iodide
|
From the standpoint of antimicrobial activity, which is the main property required for an intracanal medicament, vehicles have been classified as inert and biologically active (Table 16.1) [3]. Inert vehicles are for the most part biocompatible but do not significantly influence the antimicrobial properties of calcium hydroxide. These include distilled water, saline, anesthetic solution, glycerin, polyethylene glycol, and propylene glycol. Biologically active vehicles in turn provide additional effects to the calcium hydroxide paste, including improved antimicrobial effects. Examples include camphorated paramonochlorophenol (CPMC), chlorhexidine (CHX), and iodine potassium iodide.
Mechanisms of Antimicrobial Effects
Most bacterial species commonly found in infected root canals are eliminated in vitro after a short period of exposure to the high pH of calcium hydroxide [60]. Calcium hydroxide antimicrobial activity depends on the release of hydroxyl (OH–) ions in an aqueous environment. The hydroxyl ion has a single unpaired electron and is a highly oxidant free radical [61]. The oxidation of organic substrates by hydroxyl ions may occur either by addition of OH– to an organic molecule or due to removal of a hydrogen atom from it. Hydroxyl ions are short-lived and present high and indiscriminate reactivity. As a consequence, they usually react with biomolecules close to its point of generation [61]. Such reactions usually lead to adverse alterations [62].
Actually, lethal effects of hydroxyl ions on bacterial cells are resultant of the effects on lipids, proteins, and DNA, leading to subsequent damage to the cellular apparatus and drastically altered cellular functions. The effects are as follows: [59]
(a)
Effects on lipids. Polyunsaturated fatty acids present in membrane phospholipids are particularly sensitive to attack by hydroxyl ions. These free radicals induce lipid peroxidation. A single OH– can result in peroxidation of many polyunsaturated fatty acids in a cyclic chain reaction. Hydroxyl ions remove hydrogen atoms from polyunsaturated fatty acids, generating a free lipidic radical. This free lipidic radical reacts with oxygen to form a lipidic peroxide radical, which is highly reactive and able to propagate the chain reaction. The peroxide radical removes another hydrogen atom from a second fatty acid, generating another lipidic radical. Therefore, peroxides themselves act as free radicals, initiating an autocatalytic chain reaction and resulting in further loss of polyunsaturated fatty acids, with chain breakage, and extensive membrane damage with increased fluidity and permeability [63, 64].
(b)
Effects on proteins. The effects of hydroxyl ions on structural proteins or enzymes can cause dramatic effects on the cell and lead to death. Protein damage may be resultant of oxidation induced by hydroxyl ions, either causing oxidative modification of specific amino acids or peptide cleavage. Protein containing the amino acids methionine, cysteine, arginine, and histidine seems to be more prone to oxidation [64]. Hydroxyl ions can also induce the breakdown of ionic bonds that maintain the tertiary structure of proteins. As a consequence, the affected protein maintains its covalent structure but the polypeptide chain is randomly unraveled in variable and irregular spatial conformation. These changes frequently result in loss of biological activity, and if the protein is an enzyme, the cellular metabolism can be disrupted. Structural proteins present in the bacterial cell membranes can also be damaged by hydroxyl ions.
(c)
Effects on DNA. Hydroxyl ions cause DNA damage through an oxidative attack that results in deoxyribose oxidation, strand breakage, alteration and removal of nucleotides, and DNA-protein cross-links. Hydroxyl ions react with purine and pyrimidine bases and the deoxyribose backbone [65]. The oxidative attack to DNA bases is usually related to addition of OH– to double bonds; damage to the sugar backbone is mostly related to removal of hydrogen from deoxyribose [65]. Attack to the sugar backbone results in single-strand breaks [61]. The oxidative effects of hydroxyl ions on both DNA and the proteins associated with it may also lead to DNA-protein cross-link formation. DNA-protein cross-links may not be readily repaired and result in cell death under certain circumstances [61].
Antimicrobial Effectiveness in Endodontic Therapy
Laboratory studies have demonstrated that calcium hydroxide is effective against several bacterial species found in endodontic infections [60, 66–68]. Optimum effects were reported when the substance was in direct contact with the test bacteria in solution. In such conditions, concentration of hydroxyl ions is very high, reaching incompatible levels to survival of most bacterial species. However, in the clinical setting, such a direct contact is not always possible to attain.
In fact, clinical studies reveal that the effectiveness of calcium hydroxide in significantly improving disinfection following chemomechanical procedures is somewhat inconsistent [35, 36, 44, 46, 69–71]. This indicates that this substance has its own limitations when it comes to intracanal disinfection. In addition to the difficulties of establishing optimal contact of the medicament with bacteria colonizing the intricacies of the root canal system, other factors may help explain the limitations of calcium hydroxide in promoting predictable root canal disinfection.
Killing of bacteria by calcium hydroxide depends on the availability of hydroxyl ions in solution, which is much higher where the paste is applied (the main root canal). Calcium hydroxide exerts antibacterial effects in the root canal as long as a very high pH is sustained. If this substance needs to diffuse to tissues and the hydroxyl ion concentration is decreased as a result of the action of tissue buffering systems (bicarbonate and phosphate), acids, proteins, and carbon dioxide, its antibacterial effectiveness may be reduced or even impeded [59].
The ability of a medicament to dissolve and diffuse in the root canal system is essential for its successful antimicrobial action. A saturated aqueous suspension of calcium hydroxide possesses a high pH, which has a great toxic potential not only to bacteria but also to host cells. Nevertheless, this highly alkaline substance owes its biocompatibility to its low water solubility and diffusibility [9]. Because of these properties, cytotoxicity is limited to the tissue area in direct contact with calcium hydroxide. On the other hand, the same properties (low solubility and diffusibility) make it difficult for calcium hydroxide to promote a rapid and significant increase in the pH to eliminate bacteria present in biofilms, dentinal tubules, tissue remnants, and anatomical variations. Likewise, the buffering ability of serum and dentin controls pH changes and thereby reduces calcium hydroxide antimicrobial effectiveness [72–74]. As a consequence of all these factors, calcium hydroxide is a slowly working antimicrobial agent and requires prolonged exposure to allow for saturation of the buffering ability of dentin and tissue remnants. Therefore, long-term use of calcium hydroxide, preferably with exchanges of the medication, is necessary to maximize disinfection of the root canal system.
Bacteria located within dentinal tubules can escape from the effects of chemomechanical preparation. Thus, infected dentinal tubules may serve as a reservoir of bacteria to cause persistent infection and posttreatment apical periodontitis (Fig. 16.2) [7, 75, 76]. Intratubular infection is also the main cause of progressive external inflammatory root resorption [77]. One of the effects expected for intracanal medication is to reach and eliminate bacteria located deep within tubules. Numerous in vitro studies demonstrated that calcium hydroxide in inert vehicles has limited effectiveness against intratubular bacteria [78–86]. This is very likely to be resultant of the fact that after a short-term intracanal dressing with calcium hydroxide, the magnitude of pH reached deep in dentin may still be compatible with survival of many microbial species (Fig. 16.3) [87]. Most human bacterial and fungal pathogens grow well within a range of 5–9 pH [88, 89]. Certain bacteria, such as some enterococci, may tolerate even high pH values, varying from 9 to 11.
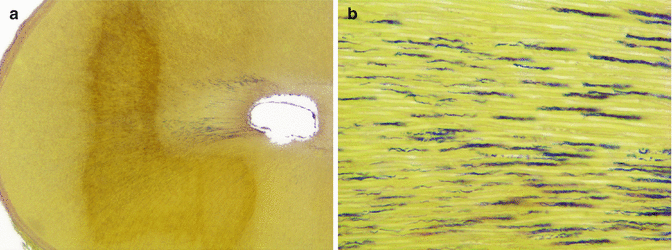
Fig. 16.2
Bacteria colonizing dentinal tubules of the root canal are a challenge for proper disinfection. (a) Cross section of the root canal of a tooth with apical periodontitis showing bacterial invasion of dentinal tubules. (b) Higher magnification revealing heavy dentinal tubule infection (Taylor’s modified Brown & Brenn staining, courtesy of Dr. Domenico Ricucci)
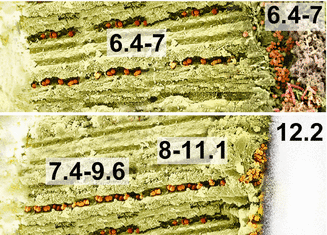
Fig. 16.3
pH changes in different regions of the radicular dentin before and after calcium hydroxide medication (Data according to Tronstad et al. [87])
In fact, resistance to calcium hydroxide has been reported for some microbial species. Enterococcus faecalis and some Candida species can be highly resistant to the alkaline effects of calcium hydroxide [60, 79, 83, 90, 91]. E. faecalis ability to resist high pH values seems to be related to a functioning proton pump, which drives protons into the cell to acidify the cytoplasm [91]. E. faecalis and Candida species are commonly found in root canal-treated teeth with posttreatment disease [49, 92–97].
Inactivation of Bacterial Virulence Factors
Structural components of the bacterial cell are important virulence factors that stimulate and modulate the inflammatory response and induce indirect damage to host tissues. The main examples are lipopolysaccharides (LPS, a.k.a., endotoxins) and the lipoteichoic acid (LTA), components of the cell wall of gram-negative and gram-positive bacteria, respectively.
Lipid A is the portion of LPS that has been regarded as the main responsible factor for the biological effects of this molecule [98, 99]. In vitro studies demonstrated that calcium hydroxide can inactivate LPS by acting primarily on the lipid A portion, inducing the alkaline hydrolysis of ester bonds with consequent release of free hydroxy fatty acids with no or reduced toxic and pro-inflammatory effects [100–106]. However, this inactivating effect has been observed in vitro under optimal contact between LPS and calcium hydroxide. It is highly unlikely that hydroxyl ions released from calcium hydroxide can reach LPS molecules present in areas distant from the main canal in magnitude sufficient to inactivate these molecules. A clinical study revealed that the levels of LPS were reduced but still relatively high in the canal after chemomechanical preparation, and these levels were virtually unaltered after intracanal medication with calcium hydroxide, CHX, or a combination of both [107].
LTA is a polymer of glycerol phosphate linked to fatty acids. It has been demonstrated that calcium hydroxide can detoxify LTA and attenuate its pro-inflammatory ability [108]. These inactivating effects are supposed to be related to deacylation of LTA induced under high alkaline conditions. Deacylated LTA does not stimulate Toll-like receptor 2, the host molecule responsible for recognition of and response to LTA, and the consequent release of pro-inflammatory cytokines [108]. There are no clinical studies reporting on the effects of calcium hydroxide medication on LTA intracanal levels.
Thus far, it remains to be determined whether these inactivating effects of calcium hydroxide on LPS and LTA can be consistently observed in vivo and, if so, what is the actual relevance for the long-term treatment outcome. After all, there is no clear indication that LPS or LTA molecules, in the absence of living bacteria, can induce or maintain periradicular inflammation beyond a certain point in time. Moreover, it is important to point out that virulence factors other than LPS and LTA can also be involved in the pathogenesis of apical periodontitis, usually in a mixture of factors released from multispecies biofilms [109]. This scenario makes the analysis of the effects against specific factors like LPS or LTA somewhat simplistic.
Combination with Biologically Active Vehicles
In an attempt to sidestep the limitations of calcium hydroxide pastes in inert vehicles (e.g., distilled water, saline, glycerin), association of this substance with other antibacterial medicaments, such as CPMC or CHX, has been evaluated [68, 83, 110, 111].
Paste in CPMC
In vitro studies have demonstrated that calcium hydroxide paste in CPMC has a broader antimicrobial spectrum (eliminating microorganisms that are resistant to calcium hydroxide) and a larger radius of antimicrobial action (eliminating microorganisms located in regions more distant from the vicinity where the paste was applied) kills microorganisms faster and is less affected by serum and necrotic tissue than mixtures of calcium hydroxide with inert vehicles [59, 68, 72, 83, 84, 112–119]. The larger radius of action may be a result of the low surface tension of CPMC and/or its high solubility in lipids. Glycerin has been added to the paste to dilute CPMC and facilitate both handling and further removal of the paste from the canal. Although CPMC exhibits high toxicity when used alone, satisfactory biocompatibility results have been observed in animal studies [120, 121]. Clinical studies evaluating the incidence of postoperative pain [122], antibacterial activity [37, 40], and treatment outcome [123] have demonstrated optimal results when using an antibacterial protocol for treatment that includes a 7-day interappointment medication with calcium hydroxide/CPMC/glycerin paste.
Paste in CHX
In vitro studies investigating the antimicrobial effectiveness of the combination calcium hydroxide and CHX have shown conflicting results. Some studies demonstrated that the antimicrobial effects of calcium hydroxide are significantly increased when adding CHX in a paste [110, 124–126], while others have shown no significant increase in activity [112, 127]. However, the antibacterial efficacy of CHX may be significantly reduced after mixing with calcium hydroxide [112, 126, 127].
Although some clinical studies have shown no advantage in using calcium hydroxide combined with CHX [71, 107], others have reported good results for this association [38, 128–130]. Zerella et al. [128] reported that intracanal dressing with a mixture of 2 % CHX and calcium hydroxide was at least as effective as calcium hydroxide in an inert vehicle in the disinfection of root canal-treated teeth with apical periodontitis. In a clinical study evaluating the antibacterial effectiveness of a treatment protocol against primary infections, Siqueira et al. [38] used 0.12 % CHX as the irrigant during chemomechanical preparation and found an incidence of positive cultures of 54 %. Further intracanal medication with calcium hydroxide paste in 0.12 % CHX significantly decreased the number of positive cultures to 8 %. Paiva et al. [129] used several sensitive molecular biology techniques to evaluate the clinical antibacterial effects of chemomechanical preparation using NiTi rotary instrumentation and NaOCl irrigation (S2), a final rinse with CHX (S3), and then 1-week interappointment medication with calcium hydroxide/CHX paste (S4). Treatment procedures promoted a decrease in microbial diversity and significantly reduced the incidence of positive results and the bacterial counts. In general, each subsequent treatment step improved disinfection. In S2, 64 % of samples were still positive for the presence of bacteria, decreasing to 43 % in S3 and then to 14 % in S4. The number of positive results was significantly lower for S4 when compared with S2, and the same was true for bacterial counting analysis. The authors concluded that supplementary steps consisting of a final rinse with CHX followed by calcium hydroxide/CHX interappointment medication promoted further decrease of the bacterial bioburden to levels significantly below those achieved by the chemomechanical procedures alone. Oliveira et al. [130] demonstrated that intracanal medication with calcium hydroxide/CHX paste had significant supplementary effects in eliminating endotoxins from infected canals and/or neutralizing their cytotoxic effects.
CHX remains stable at pH 5–8 and, as the pH increases, ionization decreases. Association of calcium hydroxide with CHX maintains a high pH value, which is similar to calcium hydroxide paste using water as vehicle [110, 128]. CHX antimicrobial activity is influenced by pH conditions, with the optimal range of 5.5–7, and at high pH values, it precipitates and may be unavailable as an antimicrobial agent [128]. Despite the expected high loss of CHX when mixed with calcium hydroxide, the combined resulting antimicrobial effect may still be of clinical significance, as demonstrated by the studies discussed above [38, 128–130]. This combination presents significant antibacterial effects, which may be related to small residues of active CHX still present in the paste, even though the effects of the high pH of the paste cannot be disregarded.
Table 16.2 summarizes several clinical studies investigating the percentage of cases that remained positive for the presence of detectable bacteria after using different treatment protocols.
Table 16.2
Clinical studies evaluating the antimicrobial effects of chemomechanical preparation and intracanal medication
Study
|
Irrigation
|
Medication
|
Time of medication
|
Microbiological technique
|
Cases positive for bacteria after medicationa
|
---|---|---|---|---|---|
Byström et al. (1985) [60]
|
0.5 or 5 % NaOCl
|
Calcium hydroxide
|
30 days
|
Culture
|
0/35 (0 %)*
1/35 (3 %)** 2–4 dl
|
Reit and Dahlén (1988) [156]
|
0.5 % NaOCl
|
Calcium hydroxide
|
14 days
|
Culture
|
8/32 (25 %)*
9/32 (28 %)** 7 dl
|
Orstavik et al. (1991) [157]
|
Saline
|
Calcium hydroxide
|
7 days
|
Culture
|
8/22 (36 %)*
|
Sjögren et al. (1991) [69]
|
0.5 % NaOCl
|
Calcium hydroxide
|
7 days
|
Culture
|
0/18 (0 %)*
0/18 (0 %)** 1–5 wl
|
Yared and Dagher (1994) [158]
|
1 % NaOCl
|
Calcium hydroxide
|
7 days
|
Culture
|
19/60 (32 %)*
|
Shuping et al. (2000) [35]
|
1.25 % NaOCl
|
Calcium hydroxide
|
7–203 days
|
Culture
|
3/40 (7.5 %)*
|
Lana et al. (2001) [159]
|
2.5 % NaOCl
|
Calcium hydroxide
|
7 days
|
Culture
|
4/27 (15 %)*
7/27 (26 %)** 7 dl
|
Peters et al. (2002) [70]
|
2 % NaOCl
|
Calcium hydroxide
|
28 days
|
Culture
|
15/21 (71 %)*
|
Kvist et al. (2004) [160]
|
0.5 % NaOCl
|
Calcium hydroxide
|
7 days
|
Culture
|
16/43 (37 %)*
|
McGurkin-Smith et al. (2005) [33]
|
5.25 % NaOCl
|
Calcium hydroxide
|
7–110 days
|
Culture
|
4/24 (17 %)*
|
Waltimo et al. (2005) [46]
|
2.5 % NaOCl
|
Calcium hydroxide
|
7 days
|
Culture
|
6/18 (33 %)*
|
Zerella et al. (2005) [128]b
|
1 % NaOCl
|
Calcium hydroxide
|
7–10 days
|
Culture
|
10/20 (50 %)*
|
Zerella et al. (2005) [128]b
|
1 % NaOCl
|
Calcium hydroxide/2 % chlorhexidine
|
7–10 days
|
Culture
|
7/20 (35 %)*
|
Chu et al. (2006) [161]
|
0.5 % NaOCl
|
Calcium hydroxide
|
7 days
|
Culture
|
11/35 (31 %)*
|
Manzur et al. (2007) [71]
|
1 % NaOCl
|
Calcium hydroxide
|
7 days
|
Culture
|
2/11 (18 %)*
|
Manzur et al. (2007) [71]
|
1 % NaOCl
|
Calcium hydroxide/2 % chlorhexidine
|
7 days
|
Culture
|
3/11 (27 %)*
|
Manzur et al. (2007) [71]
|
1 % NaOCl
|
2 % chlorhexidine (gel)
|
7 days
|
Culture
|
5/11 (45.5 %)*
|
Paquette et al. (2007) [32]
|
2.5 % NaOCl
|
2 % chlorhexidine (liquid)
|
7–15 days
|
Culture
|
15/22 (68 %)*
|
Vianna et al. (2007) [107]
|
2 % chlorhexidine (gel)
|
Calcium hydroxide
|
7 days
|
Culture
|
5/8 (62.5 %)*
|
Vianna et al. (2007) [107]
|
2 % chlorhexidine (gel)
|
2 % chlorhexidine (gel)
|
7 days
|
Culture
|
4/8 (50 %)*
|
Vianna et al. (2007) [107]
|
2 % chlorhexidine (gel)
|
Calcium hydroxide/2 % chlorhexidine
|
7 days
|
Culture
|
4/8 (50 %)*
|
Wang et al. (2007) [162]
|
2 % chlorhexidine (gel)
|
Calcium hydroxide/2 % chlorhexidine
|
14–29 days
|
Culture
|
3/36 (8 %)*
|
Sakamoto et al. (2007) [163]
|
2.5 % NaOCl
|
Calcium hydroxide/CPMC
|
7 days
|
PCR
|
10/15 (67 %)*
|
Siqueira et al. (2007) [36]
|
2.5 % NaOCl
|
Calcium hydroxide
|
7 days
|
Culture
|
2/11 (18 %)*
|
Siqueira et al. (2007) [37]
|
2.5 % NaOCl
|
Calcium hydroxide/CPMC
|
7 days
|
Culture
|
1/11 (9 %)*
|
Siqueira et al. (2007) [38]
|
0.12 % chlorhexidine
|
Calcium hydroxide/0.12 % chlorhexidine
|
7 days
|
Culture
|
1/13 (8 %)*
|
Rôças and Siqueira (2010) [42]
|
2.5 % NaOCl
|
Calcium hydroxide/CPMC
|
7 days
|
PCR
|
10/15 (67 %)*
|
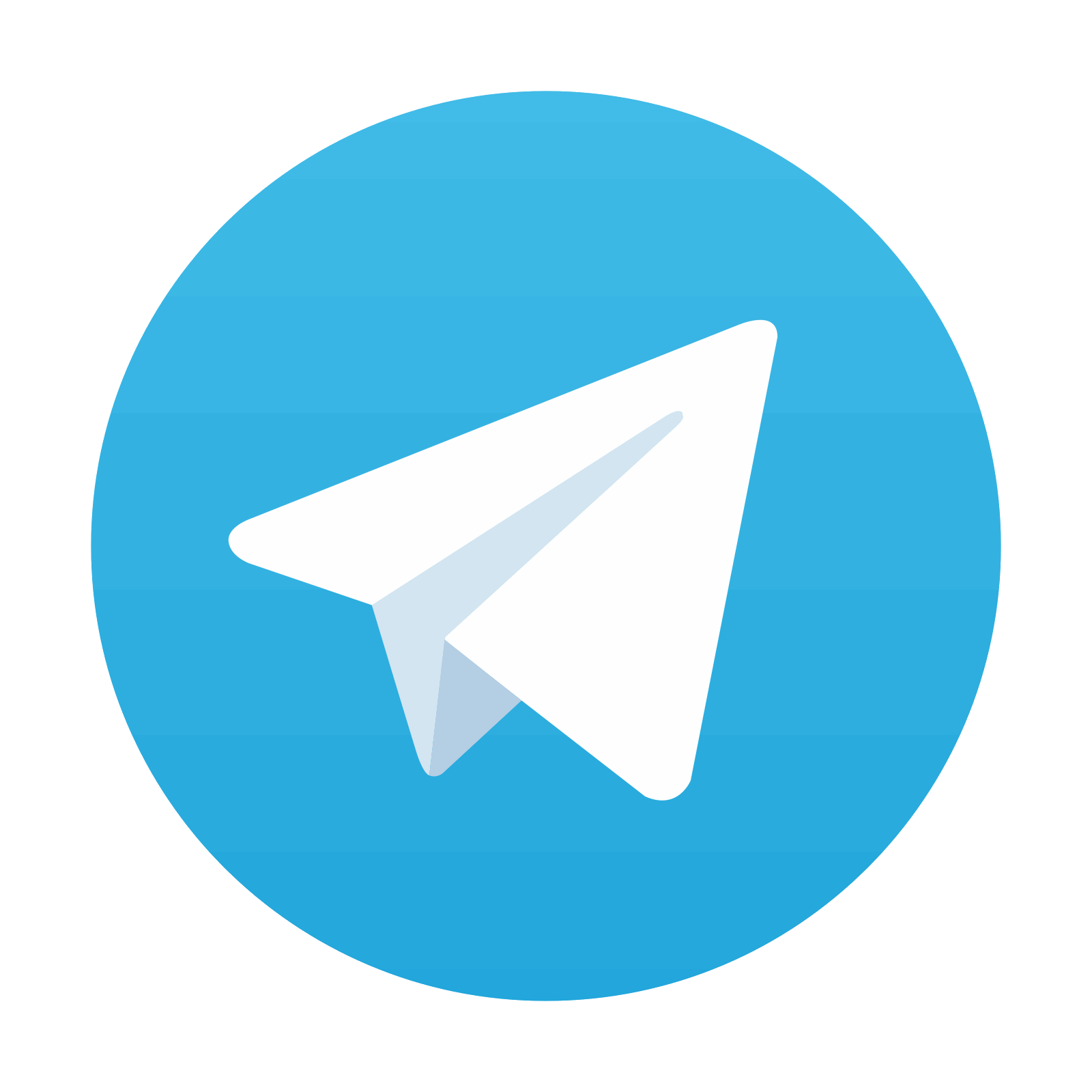
Stay updated, free dental videos. Join our Telegram channel

VIDEdental - Online dental courses
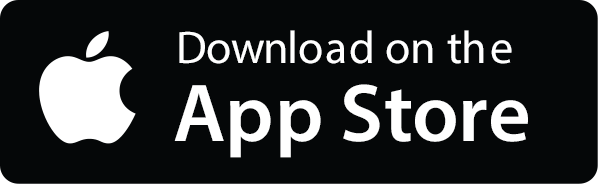
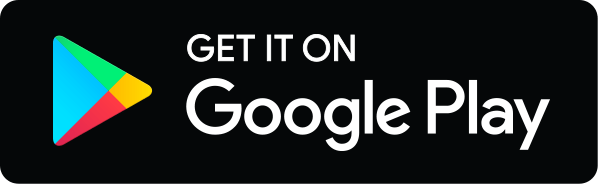