Introduction
In this systematic review, we analyzed whether recommended maximum insertion torque values of 5 to 10 Ncm were associated with higher success rates of orthodontic mini-implants compared with mini-implants inserted with maximum insertion torque values beyond this range. Objective assessments of stability, variables that influence maximum insertion torque values, and adverse effect of interventions were also assessed in the studies selected for our PICO (patient problem or population, intervention, comparison, and outcomes) question.
Methods
Computerized and manual searches of the literature were conducted up to February 24, 2012, for human studies that assessed these objectives. Our eligibility criteria selected studies that (1) used sample sizes of 10 or more, (2) recorded maximum insertion torque during the insertion of orthodontic mini-implants, (3) inserted implants with a diameter smaller than 2.5 mm, and (4) applied orthodontic forces for a minimum duration of 4 months. Confounding was assessed through the analysis of risk of bias, and the validity of outcomes was rated according to the GRADE approach. The Cochrane Handbook for Systematic Reviews of Interventions was our main guideline for the methodology.
Results
Seven nonrandomized studies met the eligibility criteria. All associations between specific maximum insertion torque values and success were based on literature rated as having low quality. The reasons for these judgments included subjective definitions of success, poor-quality torque sensors, and high risks for selection, performance, detection, and reporting biases. A risk of multiple publication bias was also suspected. All associations between maximum insertion torque and factors related to implant, patient, location, and surgery were rejected; few studies reported on adverse effects.
Conclusions
Currently, no evidence indicates that specific maximum insertion torque levels are associated with higher success rates for orthodontic mini-implants. Additional research on this topic is therefore necessary. The following guidelines for future studies are suggested: (1) systematically review the animal and laboratory literature, (2) perform maximum insertion torque tests on artificial bone, (3) test associations in animal studies before conducting clinical trials, (4) test associations between maximum insertion torque and the stability of orthodontic mini-implants with objective quantitative recordings rather than subjective qualitative measures, (5) measure maximum insertion torque with digital sensors rather than with mechanical devices, (6) assess the stability of orthodontic mini-implants at preestablished times, (7) consult our risk-of-bias analysis, and (8) analyze the adverse effects of interventions.
Many variables that affect the stability of orthodontic mini-implants (also called temporary anchorage devices) are still poorly understood. It has been suggested that excessive torque forces applied during the insertion of these devices can cause necrosis of the surrounding bone and compromise their success. It is therefore necessary to understand at what levels torque strains remain physiologic and can guarantee the stability of these implants.
Finding reliable sources for stationary anchorage has long been a challenge for orthodontists. Orthodontic mini-implants have been introduced as promising solutions for this problem, but their outcomes are not always consistent. These devices can loosen, become mobile, or even migrate. Variables that influence their success rates include factors related to patient, implant, location, surgery, orthodontics, and implant maintenance. This review will focus on insertion torque, which is a subgroup of surgery-related factors. Insertion torque results from frictional resistance between the screw thread and its surrounding bone and is a standard to evaluate mechanical stability. Maximum insertion torque is expressed in Newton centimeters (Ncm) and is the maximum torque value recorded during the insertion of orthodontic mini-implants. The stability of implants can be divided into primary and secondary. The former is mechanical stabilization achieved immediately after insertion, and the latter is attained when new bone forms at the implant interface.
To achieve initial stability, a certain level of maximum insertion torque is necessary. Studies with dental implants have shown that increases in peak insertion torque can reduce the amount of micromotion and improve their success. However, excessive stress to the bone can cause necrosis and local ischemia and might impede osseointegration and hence secondary stability. Such an association was also suggested in various clinical studies in the orthodontic literature. Animal studies have associated higher maximum insertion torque values and overtightening of orthodontic mini-implants with fractures of the cortical bone. The orthopedic literature has shown that overtightening can damage and cause stripping of the bone, and this can lead to diminished holding strength with losses in pullout strength up to 40% to 50%. To control excessive stress during the insertion of orthodontic mini-implants, torque ratchets or torque sensors have been developed.
Clinicians want to know whether specific maximum insertion torque values are associated with higher success rates. If a range of safe torque levels can be identified, they also want to learn which variables affect these measures. Maximum insertion torque values in the range of 5 to 10 Ncm have been presented as the gold standard in several clinical articles, and Google Scholar has recorded over 135 citations for one of these articles. However, conflicting findings on this issue have also been recorded in the orthodontic literature.
Because of this disagreement and because currently no reviews have addressed these issues, a systematic review was deemed appropriate. The following PICO (patient problem or population, intervention, comparison, and outcomes) question was therefore asked: Is the application of maximum insertion torque values in the range of 5 to 10 Ncm (intervention) during the insertion of orthodontic mini-implants in patients who require maximum anchorage during treatment associated with higher success rates of orthodontic mini-implants (outcomes) than those inserted with maximum insertion torque values beyond this range (comparison)? Adverse effects of interventions and variables that influence insertion torque levels were also assessed in the studies that were selected for our PICO question.
Material and methods
The protocol for undertaking a systematic review is presented in a flow diagram ( Fig 1 ), based on the Cochrane Handbook for Systematic Reviews of Interventions , and the CONSORT and PRISMA statements.
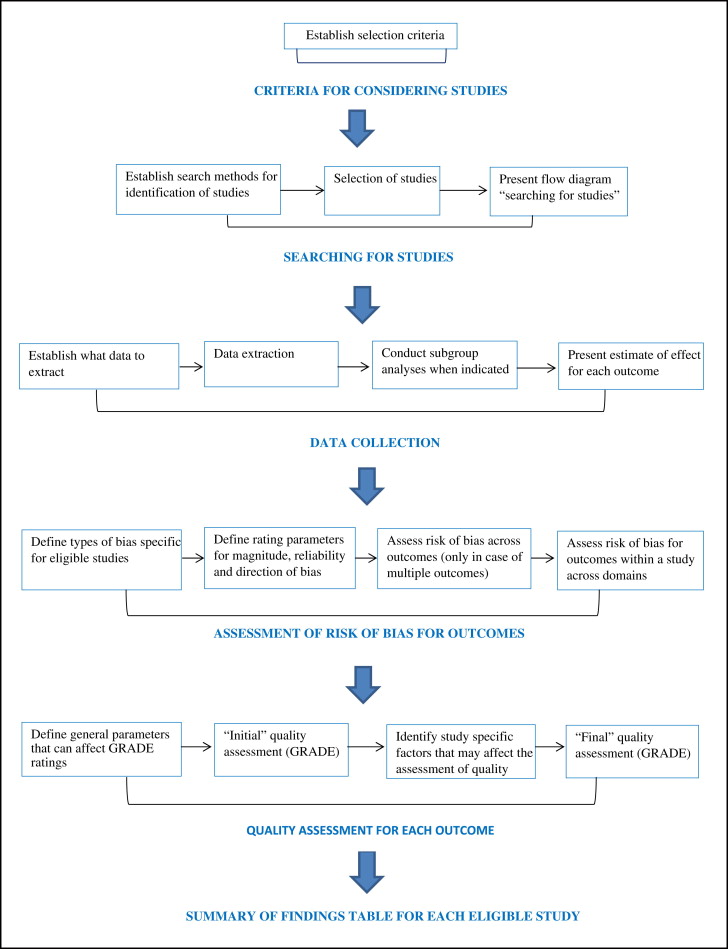
To address the objectives of this review, we defined the selection criteria for the types of studies, interventions, outcomes, and timing of the results as follows.
- 1.
Human studies with minimum samples sizes of 10 were considered. Animal studies, laboratory studies, technique articles, case reports, opinion papers, reviews, and in-vitro studies were excluded.
- 2.
Our preferred choice of research design was the randomized controlled trial, but nonrandomized studies with a low or moderate risk of bias were also assessed. This decision was based on the justifications of the guidelines of the Cochrane Handbook for Systematic Reviews of Interventions and the Centre for Evidence Based Medicine at the University of Oxford in the United Kingdom. The rationales for including nonrandomized studies in a systematic review are the following: (1) high-quality nonrandomized studies could produce a better unbiased effect size compared with low-quality randomized controlled trials; (2) randomized controlled trials could be unavailable for ethical reasons; (3) nonrandomized studies place the validity of the current literature in perspective and show the need for future research; (4) findings of a review of nonrandomized studies might be helpful in designing subsequent studies; (5) nonrandomized studies could reveal potential unexpected or rare harms of interventions ; and (6) the validity of nonrandomized studies could be upgraded for demonstrating a large treatment effect.
- 3.
If moderate- or high-quality randomized controlled trials were identified, nonrandomized studies were not consulted for their treatment effect, but only for additional information on adverse effects of the intervention.
- 4.
Patients of either sex, in any ethnic, socioeconomic, or age group, and in any setting in need of stationary anchorage during treatment with fixed orthodontic appliances were included.
- 5.
Interventions must include inserted orthodontic mini-implants for stationary orthodontic anchorage.
- 6.
Interventions must include records of the maximum insertion torque values during the insertion of orthodontic mini-implants. Maximum insertion torque represents primary stability and is defined as the maximum torque value recorded from the beginning to the end of the insertion process of orthodontic mini-implants. Maximum insertion torque is expressed in Newton centimeters and is recorded with either mechanical or electronic screwdrivers.
- 7.
Interventions must include insertion of orthodontic mini-implants with a diameter smaller than 2.5 mm. This limit was chosen because larger implants would not classify for specific orthodontic indications (eg, interradicular positioning). Articles on miniplates were excluded because of their different biomechanical characteristics.
- 8.
Studies that applied forces for more than 120 days were included. This arbitrary time frame was chosen because most orthodontic objectives cannot be completed in less than 4 months.
- 9.
Primary and secondary outcomes as well as adverse effects were assessed for the intervention under review. Eligibility was established irrespective of the outcomes measured or reported.
Success was selected as the primary outcome for our question. The following characteristics were defined for this parameter.
- 1.
An orthodontic mini-implant was considered successful when it could be loaded with orthodontic forces and fulfill its anchorage objectives during a minimum period of 4 months.
- 2.
Orthodontic mini-implants that were lost or had become unusable were considered to be failures. This group also included implants that fractured at insertion or during orthodontic treatment.
- 3.
The timing for this outcome assessment was divided into 3 time frames: short term (4-6 months), medium term (6 months-1 year), and long term (1 year and longer).
One adverse effect, fractured implants, was also included as a primary outcome and expressed in ratios (number of fractured implants per total number of inserted implants).
Three types of secondary outcomes were assessed: (1) subjective stability of the orthodontic mini-implants, (2) objective stability of the orthodontic mini-implants, and (3) variables that influenced maximum insertion torque values.
The stability of orthodontic mini-implants can be measured either subjectively by a clinician or objectively with various measuring devices. Immobility, mobility, and displacement were used as parameters to classify subjective stability and defined according to a recent systematic review.
- 1.
Success without mobility (score 0): implants with no clinically detectable mobility that could fulfill all necessary orthodontic anchorage objectives.
- 2.
Success with mobility (score 1): implants that had become mobile but could still fulfill all necessary orthodontic anchorage objectives.
- 3.
Success with displacement (score 2): implants that had become displaced but could still fulfill all necessary orthodontic anchorage objectives.
- 4.
Not specified success (score NSS): the type of success of the implants was not specified and included scores of 0, 1, and 2.
The objective stability of orthodontic mini-implants was measured at their removal with mechanical or digital instruments. Values that were specific for each instrument were recorded: eg, removal torque or resonance frequency values.
Variables that might influence maximum insertion torque values of orthodontic mini-implants were classified under the following factors: implant, patient, location, and surgery. Associations between maximum insertion torque and these parameters were tested according to the following criteria:
- 1.
An association with maximum insertion torque was only considered if it was based on samples sizes of 10 patients or more.
- 2.
A proposed association with maximum insertion torque was rejected when the article presented direct proof that at least 1 influencing variable was not controlled. Lack of information about confounding factors was not sufficient to reject an association.
- 3.
Only associations that presented their P values and/or confidence intervals were considered.
Adverse effects of insertion procedures of orthodontic mini-implants were assessed according to the guidelines in a recent systematic review. Adverse effects included implant fracture at insertion, biologic damage, inflammation, and pain and discomfort. Biologic damage was defined according to the following parameters:
- 1.
No biologic damage (score 0): no biologic damage had occurred, and no correcting dental procedures were considered necessary.
- 2.
Reversible biologic damage (score 1): biologic damage that is completely reversible with simple dental procedures. This group included removal of hyperplastic tissue and fractured orthodontic mini-implants that could be removed without causing irreversible damage.
- 3.
Irreversible biologic damage (score 2): biologic damage that is not completely reversible with simple dental procedures. This group included tooth, nerve, sinus, and blood vessel damage; fractured mini-implants that could not be removed; and the need for orthognathic surgery caused by uncontrolled biomechanics with orthodontic mini-implants.
- 4.
Not specified biologic damage (score NSBD): the biologic damage was described, but the type of damage was not identified.
- 5.
Postimplant biologic damage (score PIBD): the biologic damage was caused by treatment with orthodontic mini-implants but occurred or was detected after removal of the screw.
Inflammation was measured either within the first month of implant placement or beyond this time limit, and defined as follows:
- 1.
No inflammation: (score 0): no signs of inflammation during the entire period of treatment with orthodontic mini-implants.
- 2.
Temporary inflammation (score 1): inflammation was confined to the first month.
- 3.
Continuing inflammation (score 2): inflammation lasted longer than the first month.
- 4.
Not specified inflammation (score NSI): the duration of the inflammation was not specified.
Pain and discomfort were measured in the first 2 weeks after implantation or beyond and defined as follows:
- 1.
No pain or discomfort (score 0): no pain or discomfort during the entire treatment period with orthodontic mini-implants.
- 2.
Moderate pain or discomfort (score 1): moderate pain or discomfort noted in the first 2 weeks.
- 3.
Severe pain or discomfort (score 2): severe pain or discomfort noted in the first 2 weeks.
- 4.
Continuing pain or discomfort (score 3): pain lasting longer than 2 weeks.
- 5.
Not specified pain (score NSP): pain and discomfort were described, but their quality or duration were not specified.
Search methods for identification of studies
To find eligible studies for our PICO question, we consulted the following electronic data bases through February 24, 2012: Google Scholar Beta, PubMed (Medline), Embase (Ovid), CENTRAL, Science Direct, Scopus, Web of Science, LILACS, and AJOL. Eligible reports were also searched in the grey literature, because excluding those data bases could introduce publication bias. For this purpose, we consulted Open Grey, the Health Management Information Consortium (HMIC), and the National Technical Information Service (NTIS). The fourth author (F.E.J.), a librarian who specializes in computerized searches of health science publications at the Academic Medical Centre of the University of Amsterdam, assisted with the examination of these databases.
Transparency and reproducibility of our search process was our primary goal, and we aimed for high sensitivity and accepted low precision. To avoid inappropriate exclusion, a wide variety of search terms was combined, including the following subject headings and keywords: orthodontics, torque, implant, mini implant, micro implant, microimplant, screw, mini screw, miniscrew, micro screw, microscrew, and temporary anchorage device. For each search engine, the appropriate characters were used to truncate or explore the search terms. Nouns, adjectival, and singular and plural forms of all keywords were inserted. Search filters were avoided, and the Boolean NOT operator was not applied. To analyze whether the keywords had covered all articles on orthodontic mini-implants, the following journals were handsearched: American Journal of Orthodontics & Dentofacial Orthopedics , Angle Orthodontist , European Journal of Orthodontics , Journal of Orthodontics , Journal of Clinical Orthodontics , Seminars in Orthodontics , World Journal of Orthodontics , and International Journal of Adult Orthodontics and Orthognathic Surgery . In addition, the references in each identified article were manually screened for articles that possibly were missed by the electronic search engines. All manual and electronic searches were solicited for review articles, and references found in these reviews were screened for relevant articles. No language restrictions were applied in the search strategy, and pertinent articles were translated and reviewed.
To minimize the risk of missing eligible studies, 3 authors (R.A.M.R., L.R., L.L.), all topic experts, selected the studies. All selection procedures were performed independently by these reviewers. The fifth author (S.B.) guaranteed the soundness of the methodology and the statistics of this study. To control interexaminer agreement (kappa statistics), we followed the protocol in the Cochrane Handbook for Systematic Reviews of Interventions . According to this protocol, pilot tests on samples of reports were used to refine and clarify the eligibility criteria and ensure that these criteria could be applied consistently. Then, all titles and abstracts were examined to remove obviously irrelevant reports. The full texts of potentially relevant articles were retrieved and reviewed. Ambiguous articles were also read to prevent inappropriate exclusions. To avoid bias through duplicate publications, special attention was paid to identifying multiple reports from the same study. In case of uncertainties, the authors of such articles were contacted for clarification. Disagreements between authors about the eligibility of articles were resolved by rereading and discussion. Our selection procedures are presented in a flow diagram ( Fig 2 ).
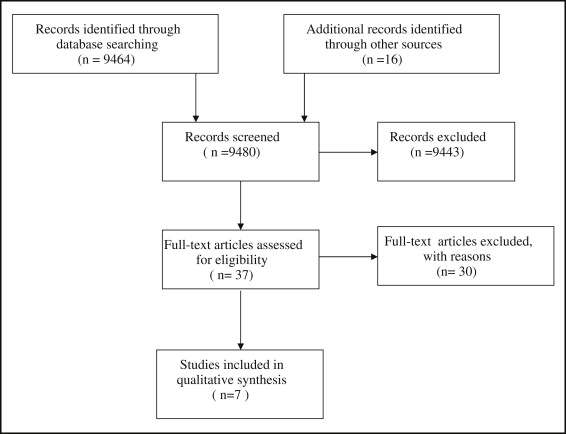
Prior to conducting this systematic review, data collection forms were designed and pilot tested for their validity. These forms included all information about a study: eg, details of methods, participants, settings, contexts, interventions, outcomes, variables, results, publications, and investigators. Although orthodontics and implant-related factors do not influence insertion torque values, they were recorded because they can influence success rates. Data that could facilitate the assessment of risk of bias were also collected. Data extraction procedures were done independently by all 3 topic experts. Disagreements between authors were resolved by rereading and discussion. Investigators of the selected studies were contacted in case of uncertainty or inability to extract all necessary information, or in case of disagreement between reviewers. If possible, individual patient data were sought directly from the authors. Subgroup analyses were performed only if the study itself or the individual patient data provided sufficient data to permit such assessments.
The validity of each study was scored through the assessment of risk of bias in the results. Critical judgments were made for the following domains: multiple publications, selection, performance, detection, attrition, and reporting bias. Performance bias was further subdivided as implant, location, surgery, orthodontics, implant maintenance, outcome assessment, operator, and duration related systematic errors. To guarantee the transparency of these judgments, detailed information on these variables was presented. Judgments were made on the magnitude, reliability, and direction of bias. Descriptions and definitions of each type of bias are given in Table I . Judgments were defined as low, high, or unclear risk of bias. The Cochrane Collaboration assigns the latter score if “1) insufficient detail is reported of what happened in the study; 2) what happened in the study is known, but the risk of bias is unknown; 3) an entry is not relevant to the study at hand.” Domains of bias were scored as high risk only when the article presented direct evidence that an entry was at risk of systematic error. Incorporating assessments of risk of bias was not used as a threshold for inclusion of studies but exclusively as a possible explanation for differences in results.
Type of bias | Description of bias |
---|---|
Multiple publication bias | Judgment on duplicate or multiple publications of the same research. |
Selection bias | Judgment on the type of sample selection concerning random sequence generation, allocation concealment, and description of sample characteristics on outcomes. |
Performance bias, implants | Judgment on the impact of, eg, the description, quality, and other implant-related confounding factors on outcomes. |
Performance bias, patients | Judgment on the impact of, eg, the number, age, physical, dental status, and other human-related confounding factors on outcomes. |
Performance bias, location | Judgment on the impact of, eg, the site of insertion, character of the mucosa, exposure, and other location-related confounding factors on outcomes. |
Performance bias, surgery | Judgment on the impact of, eg, the torquing device, flap/flapless technique, distance between screws, direction and speed of insertion, time of insertion torque assessment, self-drilling/predrilling insertion technique, starter and full-length pilot holes, depth of insertion, axial load, stripping, and other surgery-related confounding factors on outcomes. |
Performance bias, orthodontics | Judgment on the impact of, eg, the type of orthodontic movement, timing and duration of force application, the magnitude, type, and direction of the force, and other orthodontics-related confounding factors on outcomes. |
Performance bias, implant maintenance | Judgment on the impact of, eg, protocols for antibiotics, chlorhexidine rinses, oral hygiene, control of peri-implantitis and mobility, and other implant maintenance-related confounding factors on outcomes. |
Performance bias, outcome assessment | Judgment on the character and quality of outcome assessments: eg, subjective or objective methods of assessments on outcomes. |
Performance bias, operator | Judgment on the impact of operators concerning blinding on outcomes. |
Performance bias, duration | Judgment on the duration of the intervention concerning a precise description of the duration of the study on outcomes. |
Detection bias assessors | Judgment on the impact of assessors concerning blinding on outcomes. |
Attrition bias | Judgment on the impact of incomplete outcome data concerning completeness of sample, follow-up, and data on outcomes. |
Reporting bias | Judgment on the impact of the type of reporting concerning selective or incorrect reporting on outcomes. |
For the assessment of the quality of a body of evidence, we applied the GRADE approach, which was also adopted by the Cochrane Collaboration. According to this grading systems, 4 levels of quality (high, moderate, low, and very low) were scored for individual outcomes. Factors that could decrease the quality of a body of evidence included limitations in the design of the selected studies, high risk of bias, indirectness of evidence, unexplained heterogeneity, imprecision of results, unit of analysis issues, and high probability of publication bias. Factors that could upgrade the body of evidence included a large treatment effect, or when all biases would affect the magnitude of a treatment effect in the same direction. We only considered outcomes of high or moderate quality.
Risk ratio and odds ratio were chosen as measures for our dichotomous primary outcomes. If indicated, unit of analysis issues were assessed according to the level at which randomization occurs and analyzed for each design.
Statistical analysis
The criteria for a meta-analysis of the summary effect size are shown in a flow diagram ( Fig 3 ). Such a synthesis was conducted in the case of (1) low risk of bias in eligible studies, (2) consistent effect sizes (treatment effect) across the range of studies, (3) low reporting bias, (4) a high number of eligible studies, and (5) low heterogeneity between studies. Funnel plots were designed to detect reporting biases. Funnel asymmetry was assessed only when at least 10 eligible articles were identified because, with fewer studies, the power of this test is too low to distinguish chance from real asymmetry.
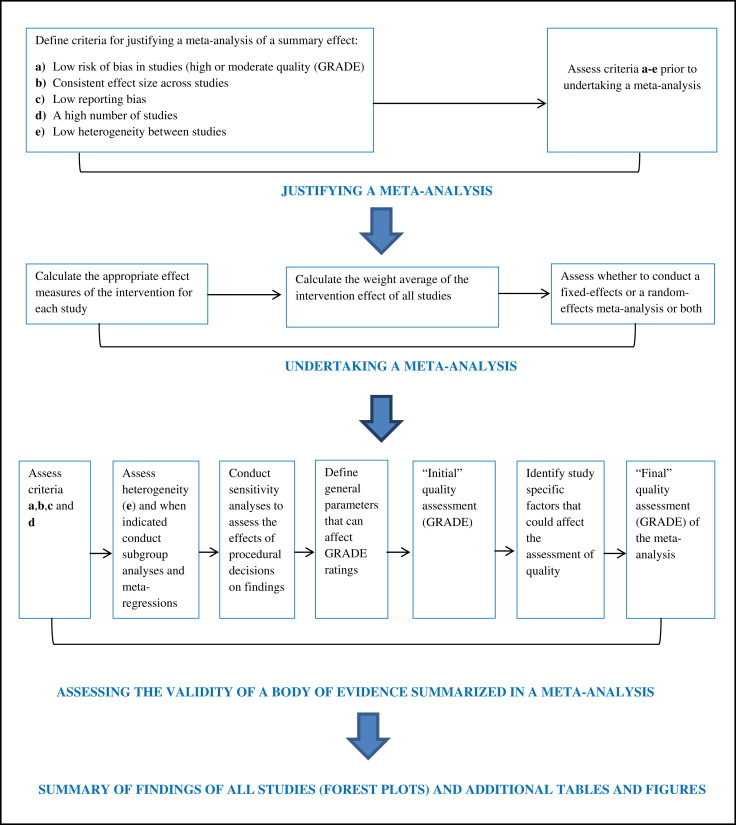
Results
A flow diagram according to the PRISMA group was used to explain the selection procedures ( Fig 2 ). No interexaminer disagreement was recorded for the inclusion of studies, indicating a kappa of 1.0. The various search methods for identification of studies found a total of 9480 abstracts with overlap ( Appendix Table I ). Thirty-seven full-text articles were assessed for eligibility; 30 were excluded. The reasons for exclusion are listed in Appendix Table II . The characteristics of the selected studies are summarized in Table II . All articles were nonrandomized cohorts, and 4 of the 7 articles were published by the same research group.
Authors and year of publication | Design of study | Patients (n) | Implant numbers, types, and characteristics | Implant sites | Insertion technique | MIT |
---|---|---|---|---|---|---|
Motoyoshi et al 2006 | Nonrandomized cohort | 41 | 124 Biodent (tapered) D 1.6 mm L 8 mm |
Posterior buccal alveolar bone in maxilla and mandible | PDI | Maxilla: 8.3 ± 3.3 Ncm Mandible: 10 ± 3.3 Ncm |
Motoyoshi et al 2007 | Nonrandomized cohort | 57 | 169 Biodent (tapered) D 1.6 mm L 8 mm |
Posterior buccal alveolar bone in maxilla and mandible | PDI | Adolescent, early load: Maxilla: 8.9 ± 2.6 Ncm Mandible: 8.5 ± 3.0 Ncm Adolescent, late load: Maxilla: 7.6 ± 2.7 Ncm Mandible: 8.8 ± 3.0 Ncm Adult, early load: Maxilla: 8.8 ± 2.8 Ncm Mandible : 8.8 ± 2.6 Ncm |
Motoyoshi et al 2007 | Nonrandomized cohort | 32 | 87 Biodent (tapered) D 1.6 mm L 8 mm |
Posterior buccal alveolar bone in maxilla and mandible | PDI | Success group: 8.83 ± 2.83 Ncm Failure group: 9.64 ± 6.62 Ncm |
Arismendi et al 2007 | Nonrandomized cohort | 9 | 34 Leone (cylindrical) D 1.5 and 2.0 mm L 10.0 and 12 mm |
Buccal alveolar bone in maxilla and palatal bone | PDI | Range, 11-25 Ncm |
Chaddad et al 2008 | Nonrandomized cohort | 10 | 17 Dual Top (machined and tapered) D 1.4, 1.6, and 2.0 mm L 6.0, 8.0, and 10.0 mm 15 C implants (sandblasted and tapered) D 1.8 mm L 8.5 mm |
Posterior buccal alveolar bone in maxilla and mandible | SDI | Torquing device set at 15 Ncm |
Motoyoshi et al 2010 | Nonrandomized cohort | 52 | 134 Biodent (tapered) D 1.6 mm L 8 mm |
Posterior buccal alveolar bone in maxilla and mandible | PDI | Maxilla: 7.67 ± 2.62 Ncm Mandible: 8.40 ± 2.51 Ncm |
Suzuki and Suzuki 2011 | Nonrandomized cohort | 95 | 120 Sistema Nacional de Implantes (cylindrical) D 1.5 mm, L 6 and 8 mm 160 ACR (tapered) D 1.5 mm, L 6 and 8 mm |
Alveolar bone in maxilla and mandible and in midpalatal suture area | PDI SDI |
PDI maxilla: 7.2 ± 1.4 Ncm PDI palate : 14.5 ± 1.6 Ncm PDI mandible: 12.4 ± 1.2 Ncm SDI maxilla: 12.1 ± 3.1 Ncm SDI palate: 21.1 ± 2.2 Ncm SDI mandible: 15.7 ± 2.3 Ncm |
High heterogeneity was found between and within the selected articles, and large standard deviations were recorded for the maximum insertion torque values ( Table II ). In most studies, the implants were inserted in poorly defined locations, various types of screws were used, and different surgical and orthodontic techniques were applied ( Appendix Tables III-IX ). Tests for funnel plot asymmetry were not indicated because only 7 eligible studies were identified. Most studies measured maximum insertion torque with mechanical torque sensors ( Table III ), but these devices cannot record insertion torque during the entire insertion process. The curves of insertion torque vs time were therefore not available, and possible stripping of the bone could not be analyzed. Suzuki and Suzuki used a digital torque sensor but measured maximum insertion torque only at the final turn of the screwdriver. Success rates ranged from averages of 55.6% to 100%, depending on the subgroup, but the timing of these assessments varied widely. These rates were based on a wide variety of subjective definitions of stability. Objective stability of orthodontic mini-implants was assessed in 3 studies ( Table III ). Two of these studies recorded removal torque values, and 1 study measured stability with digital calipers.
Authors | Torquing device | Time of success measurement | Success rate and subjective stability of OMIs | Objective stability of OMIs |
---|---|---|---|---|
Motoyoshi et al | Mechanical | 6 months or more | 85.5% (score NSS) | ND |
Motoyoshi et al | Mechanical | 6 months or more | Early load, adolescents: 63.8% (score 0); range: 55.6%-71.4% Late load, adolescents: 97.2% (score 0); range: 90.9%-100% Early load, adults: 91.9% (score 0); range: 85%-100% Success rates combined: 85.2% (score 0); range: 55.6%-100% |
ND |
Motoyoshi et al | Mechanical | 6 months or more | 87.4% (score 0); range: 85.7%-90.9 % | ND |
Arismendi et al | ND | Mean, 6.5 months Range, 3-9 months |
97.1% (score 1) | Mobility of 0.6 mm or more: At 3 months: 3% of OMIs At 8 months: 13.6% of OMIs |
Chaddad et al | Mechanical | 150 days | Machined implants: 82.5% (score 0) Surface treated: 93.5% (score 0) Success rates combined 87.5% (score 0) |
ND |
Motoyoshi et al | Mechanical | Mean, 23.1 ± 6.7 months | 90.45% (score 1) | Removal torque: Maxilla: 4.37 ± 2.20 Ncm Mandible: 4.09 ± 1.90 Ncm |
Suzuki and Suzuki | Digital | 44 ± 11 weeks | Predrilling group, 94.16% (NSS) Self-drilling group, 92.5% (NSS) |
Removal torque: 15.8 ± 3.6 to 26.9 ± 2.0 Ncm |
The risk of bias analysis was conducted with rigorous precision, because the magnitude and direction of bias is generally higher in nonrandomized studies than in randomized trials. All studies had 2 or more domains scored as high risk of bias ( Table IV ). This score was applied to selection bias in the 7 eligible studies, because all were nonrandomized studies. High-risk scores for performance bias were also identified in all articles. This type of systematic error is specified in Table V , and additional explanations for these judgments can be verified in Appendix Tables III through IX . Four of the 7 selected articles were published by Motoyoshi et al ( Table II ). Various letters were sent to these authors in 2010, 2011, and 2012 to inquire about individual patient data and the potential overlap of studies, but they did not reply. The domain “multiple publication bias” for these studies was therefore scored as an unclear risk of systematic error ( Table IV ).
Authors | Multiple publication bias | Selection bias | Performance bias | Detection bias | Attrition bias | Reporting bias |
---|---|---|---|---|---|---|
Motoyoshi et al | Unclear | High | High | Unclear | Unclear | Low |
Motoyoshi et al | Unclear | High | High | Unclear | Unclear | High |
Motoyoshi et al | Unclear | High | High | High | Unclear | High |
Arismendi et al | Low | High | High | Unclear | Low | Low |
Chaddad et al | Low | High | High | High | Unclear | Low |
Motoyoshi et al | Unclear | High | High | Unclear | Low | High |
Suzuki and Suzuki | Low | High | High | High | Low | High |
Authors | Implant-related bias | Location-related bias | Surgery-related bias | Orthodontics-related bias | Implant maintenance-related bias | Outcome assessment-related bias | Operator-related bias | Duration-related bias |
---|---|---|---|---|---|---|---|---|
Motoyoshi et al | Low | High | High | Unclear | Unclear | High | Unclear | High |
Motoyoshi et al | Low | High | High | Unclear | Unclear | High | Unclear | High |
Motoyoshi et al | Low | High | High | Unclear | Unclear | High | Unclear | High |
Arismendi et al | High | High | High | High | Unclear | Low | Low | High |
Chaddad et al | High | High | High | High | Unclear | High | High | Low |
Motoyoshi et al | Low | High | High | Unclear | Unclear | Unclear | Unclear | High |
Suzuki and Suzuki | Low | High | High | Unclear | Unclear | Unclear | Unclear | High |
Associations between maximum insertion torque and success are summarized in Table VI . Most of these assessments were based on subjective definitions of success. Chaddad et al found higher success rates at torque values above 15 Ncm, and 3 studies by Motoyoshi et al recommended placement torques between 5 and 10 Ncm. Three other articles could not associate specific maximum insertion torque with the stability of orthodontic mini-implants. The quality of the body of evidence for all associations was rated as low according to the GRADE approach ( Table VI ). Complete interexaminer agreement was recorded for these quality readings. Risk ratios were not calculated, and data were not pooled to conduct a meta-analysis of the summary effect size, because of high heterogeneity, inconsistent effect sizes, and high risk of bias across the studies ( Fig 3 ).
Association between MIT and success and character of statistical significance | Studies proposing this association | Quality (GRADE) |
---|---|---|
Mandible: MIT 5-10 Ncm higher success rates than MIT >10 ∗ or <5 Ncm (NS) Maxilla: MIT 5-10 Ncm higher success rates than MIT >10 ∗ or <5 Ncm ∗ |
Motoyoshi et al | Low |
Adolescent, early load: MIT 5-10 Ncm higher success rates than MIT >10 ∗ or <5 Ncm ∗ in the maxilla, with no differences in the mandible, and the sum of maxilla and mandible combined Adolescent, late load: Association between success rates and MIT was not analyzed, because of high success rates Adult, early load: MIT 5-10 Ncm higher success rates than MIT >10 Ncm in the maxilla, ∗ and the sum of maxilla and mandible combined ∗ |
Motoyoshi et al | Low |
MIT 8-10 Ncm higher success rates than implants with MIT >10 ∗ or <8 Ncm ∗ | Motoyoshi et al | Low |
No association between MIT and stability of OMIs | Arismendi et al | Low |
MIT >15 Ncm higher success rates than MIT <15 Ncm ∗ | Chaddad et al | Low |
No significant correlation between MIT and removal torque | Motoyoshi et al | Low |
No significant difference in MIT values between successful and failed OMIs in both PDI and SDI groups (NS) | Suzuki and Suzuki | Low |
Table VII summarizes variables associated with maximum insertion torque levels. Many contrary associations were presented for the same variable, but all 20 proposed associations were rejected because of confounding. Ten associations were excluded because bone drills had modified insertion torque values to preestablished levels, thereby skewing the outcomes of the variables under review. Adverse effects are listed in Table VIII . Inflammation of soft tissues was recorded in 2 articles ; 1 article reported 1 implant fracture during insertion and 4 during the removal of the orthodontic mini-implants.
Association with MIT | Studies proposing association with MIT | Rejected associations and reasons for rejection |
---|---|---|
Implant-related factors | ||
Same MIT in machined and surface treated screws (NS) | Chaddad et al | Rejected (a,b,c,d) |
Patient-related factors | ||
MIT and sex: no | Motoyoshi et al | Rejected (c,d,e) |
Higher MIT with increasing age ∗ | Motoyoshi et al | Rejected (c,d,e) |
MIT and age: no | Motoyoshi et al | Rejected (d,e) |
Location-related factors | ||
Same MIT on left and right sided in the success group (NS) | Motoyoshi et al | Rejected (c,d) |
Same MIT on left and right sided (NS) | Motoyoshi et al | Rejected (d,e) |
Same MIT on left and right sided (NS) | Motoyoshi et al | Rejected (c,d,e) |
Higher MIT with increasing cortical bone thickness in maxilla ∗ | Motoyoshi et al | Rejected (c,d,e) |
Higher MIT with increasing cortical bone thickness for the maxilla and mandible combined † | Motoyoshi et al | Rejected (c,d) |
MIT and cortical bone thickness in mandible: no | Motoyoshi et al | Rejected (c,d,e) |
Higher MIT in mandible than maxilla † | Motoyoshi et al | Rejected (c,d) |
Higher MIT in mandible than maxilla ∗ | Motoyoshi et al | Rejected (c,d) |
Same MIT in maxilla and mandible | Motoyoshi et al | Rejected (d,e) |
Same MIT in maxilla and mandible | Motoyoshi et al | Rejected (c,d,e) |
Higher MIT on palatal side compared with buccal side ∗ | Arismendi et al | Rejected (a,b,c,e) |
Higher MIT in midpalatal suture than in dentoalveolar area in maxilla in the predrilling and self-drilling groups ∗ | Suzuki and Suzuki | Rejected (b,c,d) |
Higher MIT in the dentoalveolar area in the mandible than in the maxilla in the predrilling and self-drilling groups ∗ | Suzuki and Suzuki | Rejected (c,d) |
No difference in MIT values between the mandible and midpalatal suture in the predrilling group (NS) | Suzuki and Suzuki | Rejected (b,c,d) |
Higher MIT in midpalatal suture than in mandible in the self-drilling group ∗ | Suzuki and Suzuki | Rejected (b,c,d) |
Surgery-related factors | ||
Higher MIT in self-drilling insertion group compared with the predrilling insertion group ‡ | Suzuki and Suzuki | Rejected (c,d) |
Total | 20 associations | 20 rejected |
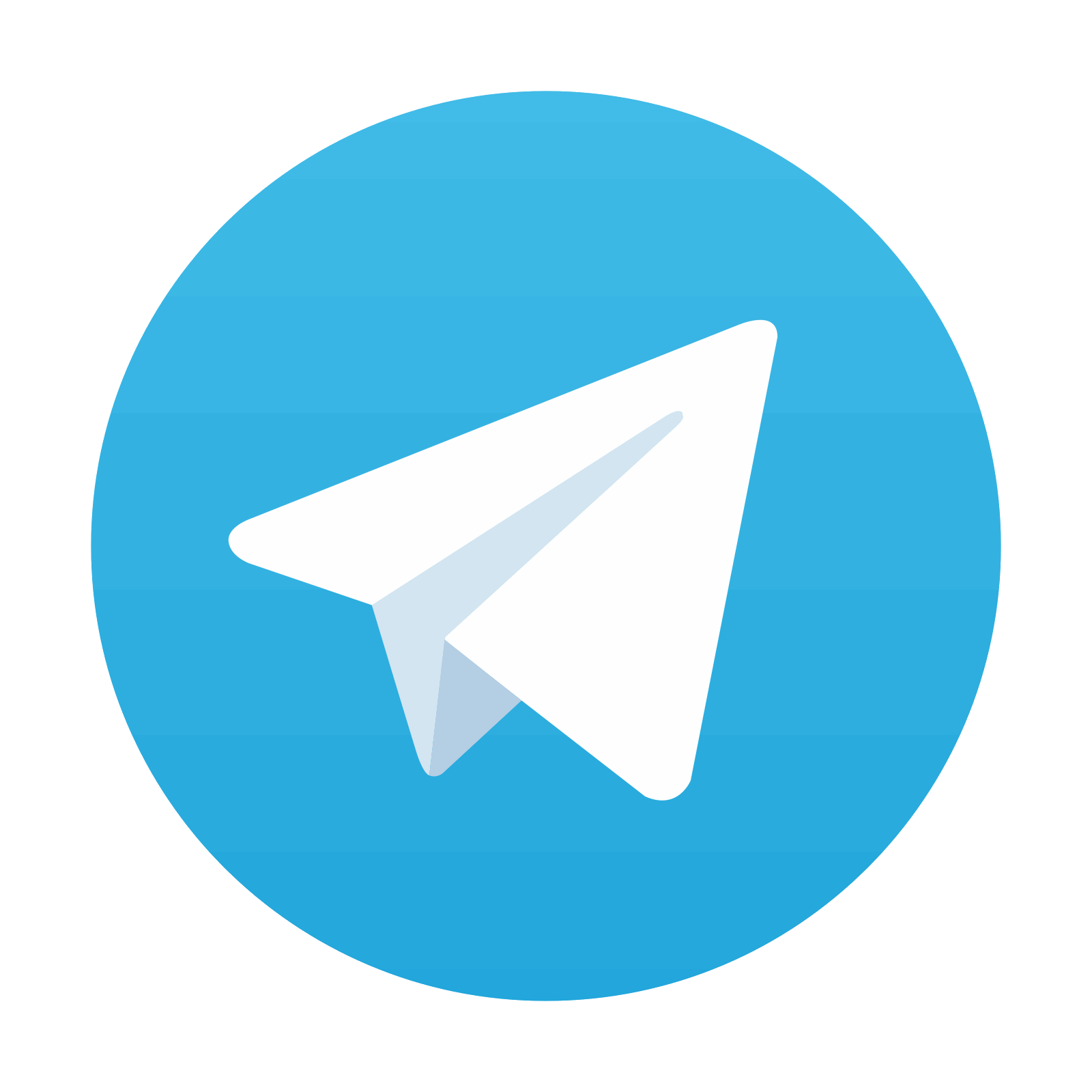
Stay updated, free dental videos. Join our Telegram channel

VIDEdental - Online dental courses
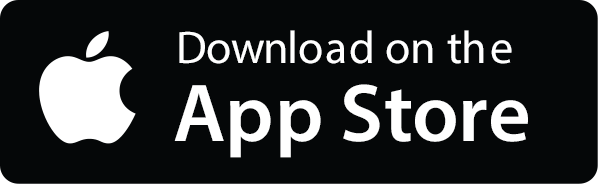
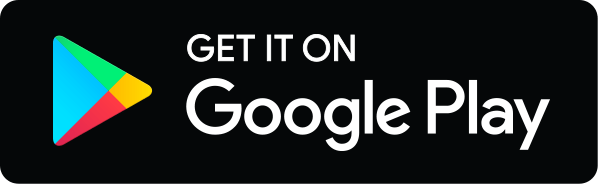
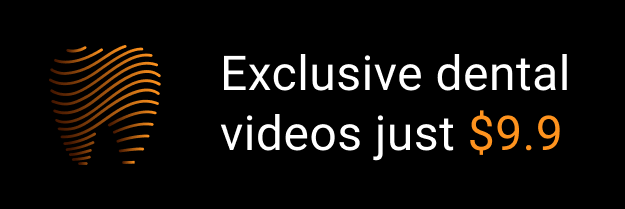