7
Injectable Anesthetic Solutions Used in Dentistry
This chapter describes the chemical and pharmaceutical composition of injectable anesthetic solutions in dental cartridges. It also analyzes procaine, which while rarely used today constitutes a reference against which the efficacy of all the others are compared.
Solution Composition
Local anesthetic solutions available in cartridges have a number of components. In addition to the two most important, the local anesthetic and the vasoconstrictor, they contain other non‐anesthetic constituents with specific functions that may have toxic or allergenic implications (Klein 1983).
Local Anesthetic
This is the main drug and the one that induces the anesthetic effect. The five modern anesthetics used in dentistry today are all amides: lidocaine, articaine, mepivacaine, prilocaine, and bupivacaine.
With the exception of lidocaine, which is achiral, i.e. has no optical isomers (Tucker 1986; Calvey 1995; Mather and Chang 2001), the others are administered as a racemic mixture, i.e. equal parts of the two (levo or S‐ and dextro or R+) stereoisomers or asymmetric carbons (Tucker 1986; Strichartz et al. 1990; Calvey 1995; Mather and Chang 2001). In most, the two isomers have approximately the same anesthetic activity (Calvey 1995).
Vasoconstrictor
This second most important component enhances the effect of the local anesthetic by promoting vasoconstriction of the blood vessels near the target tissue and inducing a mass‐volume‐time effect that heightens potency, lengthens the duration of the anesthetic effect, reduces hemorrhage where the solution is injected, and lowers the toxicity of the anesthetic by slowing its absorption into the bloodstream. There are two types of vasoconstrictors: one derived from vasopressin, namely felypressin (Octapressin®), which is less powerful and much less frequently used, and sympathomimetic vasoconstrictors such as epinephrine, norepinephrine, and levonordefrin, the three most powerful. Epinephrine is the one most commonly used worldwide.
These sympathomimetic vasoconstrictors are characterized by features with implications for dentistry.
- As they are highly sensitive to primarily oxidation‐induced degradation, they determine the shelf life of local anesthetic solutions.
- They are combined with an antioxidant (sulfite) to increase shelf life (Milano et al. 1982; Klein 1983).
- They have an acidic pH, normally around 4 (Annex 14), but ranging from 2.7 to 5.5 (USP 38 2015), which also improves their stability and enhances their solubility in aqueous solutions.
- Their levo isomeric form is used, as it is 10–200 times more powerful than the dextro form (Table 6.5, Chapter 6).
- The bitartrate form of epinephrine is used because it is more stable than the base or hydrochloride forms and like those forms is water soluble (Smith 1920; Bonica 1959).
Antioxidants (Sulfites)
These compounds lengthen the half‐life of sympathomimetic vasoconstrictors such as epinephrine because they capture the oxygen penetrating the cartridge before it engages with and inactivates the vasoconstrictor (Milano et al. 1982; Klein 1983; Huang and Fraser 1984; Schwartz and Sher 1985; Seng and Gay 1986).
The sulfites most widely used are 0.5 mg/ml sodium bisulfite (Seng and Gay 1986), sodium or potassium metabisulfite (Klein 1983; Seng and Gay 1986), and acetone sodium bisulfite 1 mg/ml (Seng and Gay 1986). Other characteristics associated with these compounds include their bitter taste, to which local anesthetics owe that same feature (Klein 1983), their contribution to maintaining an acid pH to preserve vasoconstrictors (Moorthy et al. 1984), and their efficacy in protecting vasoconstrictors for the first 18 months (American Dental Association 1983; Bennett 1984; Jastak et al. 1995). That capacity gradually declines with the amount of active antioxidant, lowering sympathomimetic vasoconstrictor activity and pH (Hondrum and Ezell 1996).
Although deemed to be safe (Bush et al. 1986; Seng and Gay 1986), low allergenic additives (Bush et al. 1986), the sulfites in local anesthetics used in dentistry have been known to trigger allergic reactions (Huang and Fraser 1984; Schwartz and Sher 1985; Schwartz et al. 1989; Dooms‐Goossens et al. 1989; Campbell et al. 2001). For poorly understood reasons this intolerance is associated with severe, corticoid‐treated asthma (Bush et al. 1986; Schwartz et al. 1989).
Seng and Gay (1986) proposed replacing sulfites with antioxidants suggested by Klein (1983), such as ascorbic acid and thioglycerol (only with Marcaine®), but they are less effective, more costly, and also pose health risks (Bush et al. 1986).
Preservatives (Methylparaben)
Preservatives are used to keep dental cartridges containing local anesthesia sterile (i.e., bacterium‐free) (Latronica et al. 1969; Larson 1977; Luebke and Walker 1978). These compounds are parabens (propyl‐, butyl‐, and methylparaben), the most widely used being methylparaben or methyl 4‐hydroxy‐benzoate (Larson 1977; Klein 1983) at concentrations of 1 mg/ml = 0.1% (Larson 1977).
Parabens are used for their bacteriostatic and fungistatic power (Schorr 1968; Latronica et al. 1969; Nagel et al. 1977; Larson 1977; Luebke and Walker 1978), low‐dose efficacy (Larson 1977; Luebke and Walker 1978), and scant toxicity (Luebke and Walker 1978). Their primary drawback is that, like the alkyl‐esters of aminobenzoic acid (Latronica et al. 1969; Nagel et al. 1977; Larson 1977; Luebke and Walker 1978; Giovannitti and Bennett 1979), they share a chemical structure with ester‐type anesthetics. Allergic reactions to anesthetic solutions induced by these compounds and the cross‐sensitivity to ester‐type local anesthetics (Aldrete and Jonhson 1969; Latronica et al. 1969; Larson 1977; Luebke and Walker 1978) are consequently common (Aldrete and Jonhson 1969; Luebke and Walker 1978; Giovannitti and Bennett 1979). The US Food and Drug Administration (FDA) has therefore banned these compounds in dental cartridges and single (although not multi‐dose) vials (Malamed 2004) because cartridge preparation and single‐dose packaging with modern industrial techniques guarantee sterility and prevent infectious disease transmission. As a result, the use of parabens, the primary cause of allergic reactions, is no longer necessary. Since their prohibition this type of adverse reaction to local dental anesthesia has declined drastically (Malamed 2004).
Non‐parabenic preservatives exist, but are seldom used. One, 0.25% (0.25 mg/ml) chlorobutanol (Klein 1983), is less effective (Luebke and Walker 1978) and edetate disodium calcium is only used in Marcaine, a brand name for bupivacaine (Klein 1983).
pH Adjustment
Solutions with sympathomimetic vasoconstrictors such as epinephrine are the most acidic, around pH = 4, ranging from 3.5 to 4.5 (Annex 14), because a low (acid) pH is needed to preserve the vasoconstrictor, which breaks down in basic media (Tainter et al. 1939; Fyhr and Brodin 1987; Bowles et al. 1995). Epinephrine is most stable at pH = 3.4 (Hondrum et al. 1993) and breaks down in a matter of hours at pH > 6 (De Jong and Cullen 1963). Acidity poses problems, however.
- Clinical trials have shown that injections are more painful, creating a burning or stinging sensation (Oikarinen et al. 1975; Moorthy et al. 1984; Kramp et al. 1999; Wahl et al. 2001).
- The anesthetic effect may be delayed because at acidic pHs, most of the anesthetic molecules are cationic and unable to penetrate the cell membrane. Very few are free bases, the lipid‐soluble form that crosses the membrane, establishing a new equilibrium with the cations in the axoplasm. That in turn activates the receptor that blocks the sodium channels, generating the anesthetic effect. The tissue is alkaline in pH (= 7.4) and buffers in the tissue mitigate the initial acidity of the solution (Tainter et al. 1939; Björn 1947b), however, although some studies (Björn 1947b) have shown that to take longer than initially believed. Today, it is known that cations can penetrate the membrane in other ways, crossing TRPV1 channels, for example (Butterworth and Oxford 2009) (see Chapter 4).
- If the acidic solution comes into contact with a metal, such as in former hypodermic metal syringes, within a few hours the release of nickel, zinc, and especially copper ions begins to irritate the tissues, an effect that may last for several days (Lundqvist et al. 1948). Dental solutions fortunately come in glass cartridges that elude such problems.
For anesthetic solutions with sympathomimetic vasoconstrictors (epinephrine and levonordefrin) the USP has established pH ranges of 3.3–5.5 for solutions with lidocaine, mepivacaine, prilocaine, and bupivacaine, and of 2.7–5.2 for articaine with epinephrine (USP 38 2015). As these solutions age, their pH tends to decline further, with both the active antioxidant and vasoconstrictor content decreasing (Hondrum and Ezell 1996).
Solutions with non‐sympathomimetic vasoconstrictors such as felypressin (Octapressin®), which is available in many European countries although not in the United States, have a pH of around 5 (Annex 14), more or less midway between solutions without vasoconstrictors and those with the sympathomimetic type.
The chemical compounds added to solutions to attempt to regulate their pH include hydrochloric acid (ClH) to lower it, and sodium hydroxide (NaOH) and sodium lactate (C3H5NaO3) (only in Marcaine®, a brand name for bupivacaine) to raise it (Klein 1983).
Other Compounds
Sterile water is used as a vehicle and salts, normally 3–6 mg/ml sodium chloride (NaCl), to render the solutions isotonic (Klein 1983). Clinical studies have shown that non‐isotonic solutions do not raise anesthetic efficacy (Nordenram 1966) and irritate subcutaneous tissue, with concomitant pain (Lewis 1919; Nordenram 1966).
Procaine (Novocaine)
Procaine, or Novocaine, is a derivative of para‐amino benzoic acid, synthesized by German chemist Alfred Einhorn in 1904 (Farbwerke vorm 1904; Einhorn and Uhlfelder 1909). The first clinical trial of this anesthetic together with epinephrine was reported by Heinrich Braun (Braun 1905) under the name Novocaine. In 1910 German dentist Guido Fischer published the first book on local dental anesthesia featuring Novocaine as the primary anesthetic (Fischer 1910). It met with such success that it was translated into several languages and its second edition was translated into English (Fischer 1912). (The first German edition sold out in just a few months.) Around 1916, Novocaine began to be known among clinicians as procaine in the United States (Benedict et al. 1932; Link and Alfred Einhorn 1959).
In the first half of the twentieth century procaine was the prototypical local anesthetic and it is the standard used in this chapter for comparison of all others. Its most prominent characteristics are summarized in Table 7.1.
Metabolism
When injected alone, procaine is retained in the injection site for 1.5 hours and in the presence of epinephrine for up to 3 h (Sung and Truant 1954). It has a short half‐life, under 8 minutes (Seifen et al. 1979), because once in the bloodstream it is hydrolyzed by pseudocholinesterase (or plasma cholinesterase or butyrylcholinesterase). It scarcely penetrates the placenta. Its metabolites are eliminated in the urine, with only 2% eliminated in the free non‐metabolized form (Brodie et al. 1948). Nonetheless, the pseudocholinesterase deficits or alterations found in one per 3000 people (Kalow and Gunn 1959) lengthen the half‐life of procaine. Its possible biotransformation pathways are listed in Annex 12.
Procaine with Epinephrine
Procaine is no longer marketed in dental cartridges, although 2% procaine ampoules and 1:1000 (1000 μg/ml) epinephrine ampoules are available. Therefore 50 ml of 2% procaine can be mixed with 1 ml of 1:1000 epinephrine to obtain 51 ml of 2% procaine with 1:50 000 (20 μ/ml) epinephrine.
The maximum absolute dose of this local anesthetic solution for adults weighing 70 kg or more is five and a half 1.8‐ml cartridges. The limiting factor is the high epinephrine concentration, at 1:50 000. The maximum absolute dose established for procaine alone in dentistry is 400 mg (5.7 mg/kg) (American Dental Association 1984).
Remarks
While procaine is less efficacious than other anesthetics (see anesthetic parameters and physical‐chemical properties in Table 7.1), in the first half of the twentieth century it proved to be sufficiently effective and much safer than its predecessor cocaine, the first of the local anesthetics. Its primary drawback is the potential for hypersensitivity reactions (allergy reactions) and in particular its cross‐sensitivity with all ester‐type anesthetics (Aldrete and Johnson 1970).
Today procaine can be used in the event of multiple and severe allergies to several amide group anesthetics, although it has fallen into disuse and been replaced by modern amide‐type anesthetic solutions that are less allergenic and exhibit an anesthetic parameter that attests to higher potency and efficacy.
Lidocaine (Lignocaine)
Lidocaine was synthesized in 1943 by Swedish researchers Nils Löfgren (1913–1967) and Bengt Lundqvist (1922–1953) (Björn and Huldt IV 1947a; Löfgren 1948; Gordh et al. 2010), who first published their findings in 1946 (Löfgren and Lundqvist 1946). It was initially known as compound LL 30, the letters designating the two researchers’ initials and the number the consecutive order of the compound of the many they studied (Gordh et al. 2010). After 1946 it was renamed Xylocaine® (Löfgren and Lundqvist 1946), a combination of the product from which it is derived, “xylidine,” and the local anesthetic suffix “caine” (Gordh et al. 2010). In 1948 it was patented in Sweden and the United States (American Dental Association 1984; Gordh et al. 2010), where it quickly replaced procaine (American Dental Association 1984). In 1951 Xylocaine was adopted as the brand name and lidocaine as the generic name (McMahon and Woods 1951). It is presently known as lidocaine in the United States and most of the rest of the world and lignocaine in the United Kingdom. Lidocaine was the first amide‐type local anesthetic to be successfully used and the only anesthetic discussed in this book, with the exception of tetracaine, that is achiral, i.e. with no levo/dextro stereoisomers (Calvey 1995).
Table 7.1 Procaine.
Pharmacological factor | Reference | |
---|---|---|
|
||
|
Farbwerke vorm (1904) | |
|
American Dental Association (1984) | |
|
||
|
||
|
Seifen et al. (1979) | |
|
Seifen et al. (1979) | |
|
Seifen et al. (1979) | |
Physical–chemical property | ||
Denotes retarded onset |
Annex 6 | |
n‐octanol 2 |
Annex 7 | |
Denotes short duration of the anesthetic effect |
Annex 9 | |
Denotes need for a vasoconstrictor to be effective |
Du Mesnil de Rochemont and Hensel (1960) Lindorf et al. (1974) |
|
Clinical factor | Reference | |
|
||
|
Annex 8 | |
|
American Dental Association (1984) | |
|
Haas et al. (2000) | |
|
||
|
||
Anesthetic parameter | Variable | 2% procaine + 1:50 000 epinephrine |
Buccal infiltration Superior lateral incisor 1 ml (Annex 21) |
Successful pulpal anesthesia (%) Duration, pulpal anesthesia (minutes) Duration, anesthesia upper lip (minutes) |
80 25 95 |
Mandibular block 1.8 ml (Annex 27) |
Duration, anesthesia lower lip (minutes) | 110 |
Pharmacological, physical–chemical and clinical properties, and anesthetic parameter.
Summary: 2% procaine with 1:50 000 (20 μg/ml) epinephrine: 80%‐25’/95’‐110′.
Lidocaine has also been used since the 1960s as an antiarrhythmic drug in medical emergencies (Harrison et al. 1963; Katz and Epstein 1968). Its most prominent characteristics are summarized in Table 7.2.
Metabolism
At a 2% concentration with 1:100 000 (10 μg/ml) epinephrine, lidocaine is removed from the injection site in around 4 hours and without epinephrine in less than 2 hours (Sung and Truant 1954). Once in the bloodstream it accumulates, in descending order, in the kidneys, lungs, brain, and heart (Sung and Truant 1954; Akerman et al. 1966). The hepatic microsomal system (Sung and Truant 1954; Akerman et al. 1966; Boyes et al. 1971; Stenson et al. 1971; Thomson et al. 1973), primarily isoform 3A of cytochrome P450 (Bargetzi et al. 1989), catabolizes 70% of the anesthetic (Boyes et al. 1971; Stenson et al. 1971). Around 80% is eliminated in the urine, although only 4% of it is excreted unchanged (Annex 12). Elimination is enhanced in acidic urine (Eriksson et al. 1966; Mihaly et al. 1978).
Lidocaine has a half‐life of around 110 minutes (Annex 11). Severe liver and kidney disorders affect its catabolism, albeit in different manners (Thomson et al. 1973). Severe liver disease may raise its half‐life by up to three times, whereas severe kidney conditions affect not half‐life but the metabolites in the blood (Thomson et al. 1973), particularly monoethylglycinexylidide (MEGX) and glycinexylidide (GX), active agents involved in lidocaine toxicity (Blumer et al. 1973; Strong et al. 1973).
This drug diffuses passively across the placenta, reaching a concentration in the umbilical vein of 60% of that found in the maternal blood vessels (Covino 1971). Its possible biotransformation pathways are listed in Annex 12.
Remarks
L‐100 or 2% lidocaine with 1:100 000 (10 μg/ml) epinephrine and L‐80, the same solution with 1:80 000 (12.5 μg/ml) epinephrine, are deemed to be the standard solutions in dentistry for several reasons (Cowan 1964):
- Their efficacy in attaining pulpal anesthesia via buccal infiltration is only exceeded by potent solutions such as 4% articaine (double the anesthetic content) with 1:100 000 (10 μg/ml) epinephrine (A‐100), or 2% lidocaine with 1:50 000 (20 μg/ml) epinephrine (L‐50) (double the vasoconstrictor content) (Annex 21).
- It is very safe, with a maximum absolute dose in dentistry of eight and a half cartridges in adults weighing 70 kg or over, more than most other solutions (Annex 10).
- As an FDA pregnancy risk category B drug, it is very safe for pregnant or nursing women.
Lidocaine concentrations of 5% have shown to be highly toxic in animal experiments and are consequently not recommended (Lambert et al. 1994; Strichartz et al. 1994; Strichartz and Lambert 1995). Conversely, 2% lidocaine solutions with 1:200 000 (5 μg/ml) epinephrine (half the vasoconstrictor as in the standard solution) have proven to be scantly effective in clinical trials (Annex 21).
Indications
Two local anesthetic solutions with epinephrine are presently available. Their characteristics and uses are discussed below.
Standard 2% Lidocaine: L‐100 with 1:100 000 (10 μg/ml) Epinephrine or L‐80 with 1:80 000 (12.5 μg/ml) Epinephrine
The epinephrine concentration used in the United States and many other countries is 1:100 000, although in some European countries, Japan, and India the standard is 1:80 000 (12.5 μg/ml). As in practice the two deliver similar results, they are indistinctly deemed to be the standard (Cowan 1964; Yamazaki et al. 2006). Nonetheless, while some authors eschew the 12.5 μg/ml dose where epinephrine is relative contraindicated, they find the 10 μg/ml concentration acceptable (Annex 17).
The maximum absolute dental dose in adults weighing 70 kg or more is 300 mg (4.3 mg/kg) of lidocaine, or eight and a half 1.8‐ml cartridges (Annex 10). Its uses are as follows:
- In mandibular blocks because:
- Pulpal anesthetic efficacy in mandibular nerve blocks depends less on the potency of the local anesthetic solution (higher anesthetic or vasoconstrictor concentration or both) (Annex 24) than on the proximity to the inferior alveolar nerve, the aponeuroses separating the anesthetic from the nerve, accessory innervation, or anatomical variations (see Chapter 19).
- Solutions with high concentrations of local anesthesia (4% articaine, 4% prilocaine) have not exhibited greater clinical efficacy in attaining pulpal anesthesia under normal circumstances (Annex 24), while inducing four to nine times more long‐term paresthesia in the inferior alveolar and lingual nerves (Haas and Lennon 1995; Garisto et al. 2010) (see Chapter 22).
- Pulpal anesthetic efficacy in mandibular nerve blocks depends less on the potency of the local anesthetic solution (higher anesthetic or vasoconstrictor concentration or both) (Annex 24) than on the proximity to the inferior alveolar nerve, the aponeuroses separating the anesthetic from the nerve, accessory innervation, or anatomical variations (see Chapter 19).
- In children, for both mandibular blocks and maxillary and mandibular buccal infiltrations to achieve pulpal anesthesia because:
- The standard solution penetrates their smaller, more porous bones very well.
- A larger volume in milliliters per child kilogram can be administered because the maximum values are higher than with most other anesthetic solutions used in dentistry (Annex 10). The result is greater safety.
- Where infiltrations need not achieve deep pulpal anesthesia, such as in gingivectomies, scaling and root planing, or gross debridement in patients with high dentinal sensitivity where only the gingival needs to be anesthetized because while it is as effective as other solutions a larger volume of anesthetic can be used at lower risk (before the maximum absolute dental dose is reached, therefore lowering the risk of toxicity) (Annex 10).
Table 7.2 Lidocaine.
Pharmacological factor | Reference | ||
|
|||
|
Löfgren (1948) | ||
|
American Dental Association (1984) | ||
|
Löfgren (1948) | ||
|
Löfgren (1948) De Jong (1977) Strichartz (1990) |
||
|
Annex 11 | ||
|
Annex 11 | ||
|
Annex 11 | ||
Physical–chemical property | |||
Denotes fast onset |
Annex 6 | ||
|
Annex 7 | ||
Denotes medium duration of the anesthetic effect |
Annex 9 | ||
Denotes need for a vasoconstrictor to be effective |
Du Mesnil de Rochemont and Hensel (1960) Lindorf et al. (1974) |
||
Clinical factor | Reference | ||
|
|||
|
Annex 8 | ||
|
American Dental Association (1984) | ||
|
Table 5.11 (Chapter 5) | ||
|
Table 5.11 (Chapter 5) | ||
|
|||
Anesthetic parameter | Variable | L‐100 or L‐80 | L‐50 |
Buccal infiltration Superior lateral incisor 1 ml (Annex 21) |
Successful pulpal anesthesia (%) Duration, pulpal anesthesia (minutes) Duration, anesthesia upper lip (minutes) |
95 45 190 |
100 60 165 |
Mandibular block 1.8 ml (Annex 27) |
Duration, anesthesia lower lip (minutes) | 200 | 200 |
Pharmacological, physical–chemical, and clinical properties and anesthetic parameter.
Summary: (L‐100) 2% lidocaine with 1:100 000 (10 μg/ml) epinephrine and (L‐80) 2% lidocaine with 1:80 000 (12.5 μg/ml) epinephrine: 95%‐45’/190’‐200′.
(L‐50) 2% lidocaine with 1:50 000 (20 μg/ml) epinephrine: 100%‐60’/165’‐200′.
L‐50, 2% Lidocaine with 1:50 000 (20 μg/ml) Epinephrine
As this solution has double the standard epinephrine concentration (20 μg/ml), it has a very beneficial hemostatic effect, raising the potency to achieve pulpal anesthesia more readily by keeping more local anesthetic in the infiltration area.
The maximum absolute dose of epinephrine in dentistry is 200 μg (2.8 μg/kg in adults >70 kg), the amount in five and a half 1.8‐ml cartridges (Annex 10). Its uses are as follows:
- Where hemostasis is required (Buckley et al. 1984) because abundant bleeding is expected, such as in oral surgery, scaling, and root planing (Chaikin 1977), the area to be treated (the gum during proximal or class V fillings or in the papillae when making impressions for fixed prosthesis) is infiltrated.
- When pulpal anesthesia is not achieved with 2% lidocaine and 1:100 000 (10 μg/ml) epinephrine L‐50 can reinforce buccal infiltration (Gruber 1950). As noted Chapter 5, two local anesthetics should not be used in the same site (see). The approach suggested here therefore calls for the same anesthetic, although the epinephrine concentration is doubled to make pulpal anesthesia more effective (Annex 21).
Interestingly, since the high epinephrine concentration in this solution delivers greater anesthetic potency by keeping more solution in the target site, the effect is shorter‐lived (Klingenström and Westermar 1964) due to the significant vasodilatory reaction observed 2–3 hours later (Lindorf 1979). The result is a shorter duration of soft tissue anesthesia than with other less potent solutions (Annex 21).
Articaine
Articaine was synthesized in 1969 (Frenkel 1989; Rahn and Ball 2001) by German pharmacologist Roman Muschaweck (1918–2007) for Farbwerke Hoechst AG at Frankfurt (Rahn and Ball 2001; Vogel 2007). In the first paper, published in 1972, it was given the experimental name Hoe 40 045 (Winther and Nathalang 1972). In 1974 it was renamed carticaine (Muschaweck and Rippel 1974; Winther and Patirupanusara 1974), acquiring its present name, articaine, in 1983 (Kirch et al. 1983; Mehta et al. 1983). It was first marketed in Germany and Switzerland in 1976 and afterwards very successfully in several countries (Table 7.3). Initially packaged in 1.7‐ml cartridges under the brand name Ultracaín®, it is now available in 1.7‐ or 1.8‐ml cartridges.
Articaine is an amide‐type anesthetic but with two special characteristics. The first is that although the intermediate linkage is an amide, it also contains an ester linkage in the side chain of its aromatic ring. The other is that its aromatic ring is a thiophene rather than a benzene. Articaine’s most prominent characteristics are summarized in Table 7.4.
Table 7.3 Articaine commercial availability: dates and countries.
Year | Country | Reference |
---|---|---|
1976 | Germany, Switzerland | Isen (2000) |
1978 | Netherlands | Isen (2000) |
1980 | Austria, Spain | Isen (2000) |
1983 | Canada | Isen (2000) |
1998 | United Kingdom | Isen (2000) |
2000 | United States Denmark |
Weaver (1999) Hillerup and Jensen (2006) |
2005 | Australiaa | Yapp et al. (2012) |
a In Australia in 2.2‐ml cartridges.
Table 7.4 Articaine.
Pharmacological factor | Reference | ||
|
|||
|
Rahn and Ball (2001) Vogel (2007) |
||
|
|||
|
|||
|
Muschaweck and Rippel (1974)Winther and Patirupanusara (1974) | ||
|
Annex 11 | ||
|
Annex 11 | ||
|
Annex 11 | ||
Physical–chemical property | |||
|
Annex 6 | ||
|
Annex 7 | ||
|
Annex 9 | ||
|
Muschaweck and Rippel (1974) | ||
Clinical factor | Reference | ||
|
|||
|
Annex 8 | ||
|
Council on Clinical Affairs (2015) AAPD (2020) |
||
Denotes low risk |
Table 5.11 (Chapter 5) | ||
Denotes low risk |
Table 5.11 (Chapter 5) | ||
|
Malamed et al. (2000) Katyal (2010) |
||
Anesthetic parameter | Variable | A‐100 | A‐200 |
Buccal infiltration Superior lateral incisor 1.8 ml (Annex 21) |
|
98 60 190 |
92 45 190 |
Mandibular block 1.8 ml (Annex 27) |
|
260 | 260 |
Pharmacological, physical‐chemical, and clinical properties and anesthetic parameter.
Summary: (A‐100) 2% articaine with 1:100 000 (10 μg/ml) epinephrine: 98%‐60’/190’‐260′.
(A‐200) 2% articaine with 1:200 000 (5 μg/ml) epinephrine:92%‐45’/190’‐260′.
Metabolism
Much less articaine (5–15%) than other amide anesthetics is metabolized in the liver by the P450 microsomal enzyme system (Isen 2000; Rahn and Ball 2001). Therein lies the advantage of this solution, as the ester side chain on its thiophene ring is rapidly hydrolyzed by plasma pseudocholinesterases (Becker and Reed 2006), which catabolize 85–95% of the drug. As articainic acid, the compound formed has no anesthetic activity or effect on the central nervous system (Müller et al. 1991; Oertel et al. 1997), the half‐life of articaine is just 25 minutes. Nonetheless, in the 1 in 3000 individuals with deficient or altered plasma cholinesterases (Kalow and Gunn 1959) the drug is metabolized in the liver. Catabolism is a two‐stage process (Vree et al. 1988; Van Oss et al. 1989) accelerated by alkaline pH (Oertel et al. 1996).
Articaine is classified as an amide‐type local anesthetic, not an ester, and exhibits no cross‐allergenic activity with para‐aminobenzoic acid anesthetics, nor is allergic sensitization common (Becker and Reed 2006). The reason for the lack of allergic potential is that when articaine is metabolized to articanic acid, a metabolite with the structure similar to para‐aminobenzoic acid (PABA) is not formed. The formation of a PABA metabolite is the reason for cross‐allergenicity amongst ester anesthetics, not amides including articaine.
Articaine is eliminated primarily (85%) in the urine, 75% as articainic acid and only 3% as free and unaltered articaine (Annex 12). Two percent is also found in the feces (Hornke et al. 1984). This drug diffuses across the placenta passively, lodging in the umbilical vein at 32% of the concentration found in the maternal blood vessels (Strasser et al. 1977). Its possible biotransformation pathways are listed in Annex 12.
Remarks
Several features of articaine merit comment.
Maximum Dose and Toxicity
This issue was discussed in Chapter 5. By way of summary, its acute experimental toxicity is very similar to that of lidocaine (Annex 8; Albalawi et al. 2018), although the enormous advantage of articaine is its high clearance rate (4.17 l/minute) and short half‐life (25 minutes) (Annex 11). The dose allowed in dentistry is therefore higher than for lidocaine (300 mg = 4.3 mg/kg). The maximum absolute dose proposed for dentistry is 500 mg (7 mg/kg) (Council on Clinical Affairs 2015; AAPD 2020), equivalent at 4% to seven 1.8‐ml or seven and a half 1.7‐ml cartridges. See Annex 10 of doses of dental local anesthetic solutions.
The use of articiane is not recommended in children under 4 years of age as there is little data to support its safety and efficacy, although a few trials have shown it to be safe (Wright et al. 1989), thus more data and clinical studies are needed to verify those findings (Malamed et al. 2000; Malamed et al. 2001; Katyal 2010; AAPD 2020).
Anesthetic Potency
Articaine has been proven to carry higher anesthetic efficacy than lidocaine. Two recent meta‐analyses comparing 4% articaine to 2% lidocaine solutions, both with 1:100 000 epinephrine, showed articaine to be more effective, particularly in pulpal anesthesia in buccal infiltrations (Katyal 2010
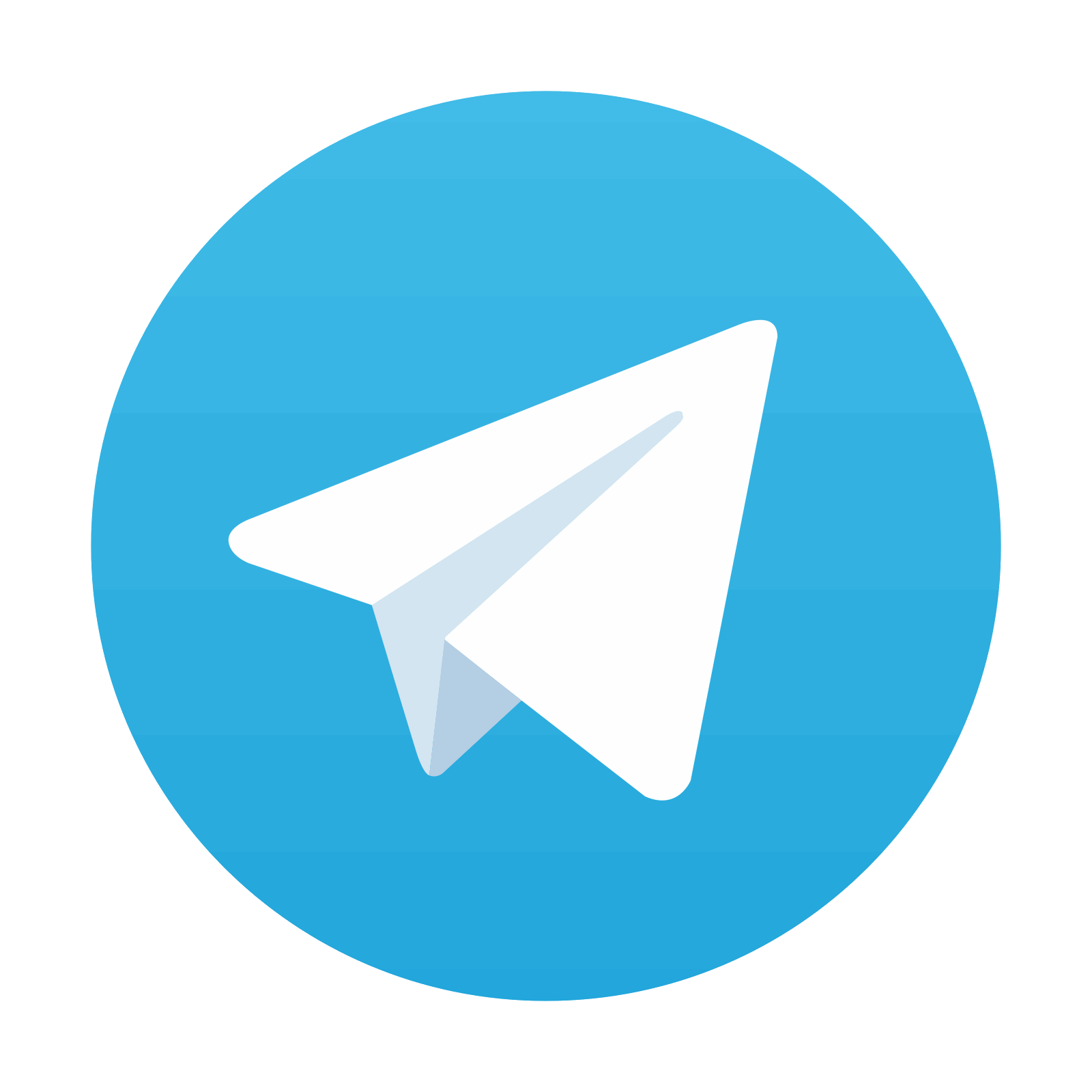
Stay updated, free dental videos. Join our Telegram channel

VIDEdental - Online dental courses
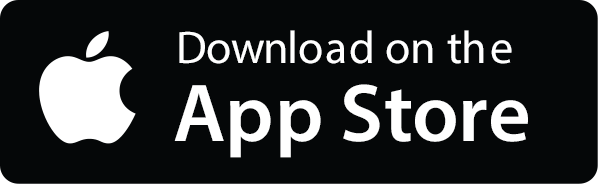
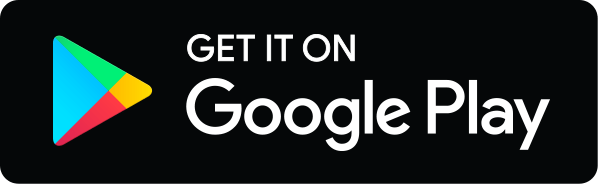