Abstract
Numerous previous studies have investigated the production of mineralised tissues by transplanting human dental pulp cells with calcium based scaffolds. The potential of alternative setups remains largely uninvestigated, therefore in this study, human dental pulp cells were encapsulated into non-calcium based biomaterial – self-assembling peptide nano-fibre hydrogel. The cell-gel constructs were cultured in full medium for 2 weeks. Then they were cultured in full medium supplemented with β-glycerophosphate, dexamethasone and l -ascorbic acid for 2 more weeks. These cell-gel constructs and plain-gel constructs (with no cells) were transplanted subcutaneously into five nude mice. The gel constructs were retrieved 4 weeks after surgery. The plain-gel constructs were all completely resorbed with no new tissue formation. The cell-gel constructs were transformed into tissue pieces that were mineralised and contained blood capillaries. Immunohistochemistry analysis confirmed the expression of multiple bone markers (osteopontin, osteocalcin, osteonectin and parathyroid hormone receptor) in these tissue pieces. Computerised analysis of the contact radiographs gave the mean radio-opaque area percentage as 78% ( N = 5, P < 0.001 compared with the 0% of the control). The results demonstrate good prospects for using human dental pulp cell plus self-assembling peptide nano-fibre hydrogel to produce mineralised tissue pieces for clinical use.
Bone grafting is commonly needed in the reconstruction of bone defects resulting from trauma or surgical procedures. Autologous bone grafting is the gold standard for clinical bone repair although its limitations have been noted by researchers who have been seeking better alternatives . Numerous in vitro studies have indicated that stem cells/progenitor cells isolated from human dental pulp are capable of producing mineralised extracellular matrix . Human dental pulp cell (HDPC) is a probable candidate cell type for bone tissue engineering although inconsistent results have been reported from previous in vivo studies with HDPCs. Some investigators have reported the successful formation of lamellar bone by transplanted HDPCs ; some have demonstrated that the capacity of different strains of HDPCs to produce mineralised tissue may vary significantly ; and others have reported the failure of HDPCs to produce mineralised tissue in their studies . Further studies on the in vivo behaviour of HDPCs are necessary before the engineering of mineralised tissue with these cells for clinical use can be considered.
As well as proper progenitor cells, appropriate scaffold material is another essential requirement for successful tissue engineering. The interactions between cells and their extracellular matrix play a crucial role in regulating cell functions . When human osteoblasts were cultured on biodegradable polymers, higher levels of osteopontin and osteonectin were produced . Despite these findings, porous biphasic hydroxyapatite/tricalcium phosphate (HA/TCP) is the predominant scaffold material used in human dental pulp cells studies, as it was in the in vivo studies mentioned above . The potential influence of other scaffold material on the production of mineralised tissues has not been fully investigated.
This pilot study tested the ability of a new combination of cell and biomaterial to produce mineralised tissue. HDPCs were encapsulated in a non-calcium based soft hydrogel material. The hydrogel scaffold being tested was a self-assembling peptide nano-fibre (SAPN) material. The authors hypothesised that this combination of cell and biomaterial could produce mineralised tissue in an in vivo environment.
Materials and methods
Isolation and culture of HDPCs
The project was ethically approved by the Institutional Review Board of the University of Hong Kong/Hospital Authority Hong Kong West Cluster. Extracted tooth samples were collected from private dental practitioners. The HDPCs were isolated as described elsewhere . Briefly, the pulp tissue obtained from tooth specimens were digested in type I collagenase (Sigma–Aldrich, USA) solution for 1 h at 37 °C. Single-cell suspensions were obtained by passing the cell extracts through 70-μm strainers. The cell suspensions were seeded into six-well plates with Dulbecco’s modified Eagle’s medium (DMEML) culture medium supplemented with 10% foetal bovine serum (FBS), 100 unit/ml penicillin plus 100 μg/ml streptomycin, and incubated at 37 °C in 5% CO 2 . The culture medium was changed twice a week. The cells were passaged to 25T and finally to 75T ( Fig. 1 A ) culture flasks once confluence was reached. Only those cell samples finishing the expansion process, reaching confluence in the 75T culture flask, within 30 days were considered to have a favourable proliferation rate, and were cryopreserved for later use. During passage 1, while the HDPCs were being expanded, a tiny portion of cells was simultaneously seeded onto cover slips for antibody testing. Two batches of HDPCs, collected from two patients, tested positive for STRO-1 and CD146 antibody ( Fig. 1 B and C), and showing a favourable proliferation rate were selected for the rest of this study.

Cell encapsulation and induction
The SAPN material was purchased from BD Biosciences (Bedford, USA) under the brand name PuraMatrix. Sucrose was added to the original (1%, w/v) BD PuraMatrix stock solution to make it 10% sucrose based. The HDPCs at passage 2 were collected and resuspended in 10% sucrose solution. Aliquots of 20 μl of the suspension, containing about 1 × 10 5 cells, were mixed with 20 μl of the PuraMatrix working solution. The mixtures were allowed to set into gel constructs in full culture medium. The medium was changed at 1, 10, and 30 min as recommended by the manufacturer. The medium was changed twice a week for 2 weeks.
To confirm the perpetuation of cells within the gel, two gel constructs were fixed with 4% paraformaldehyde in phosphate buffered saline. The gel constructs were fluorescent stained with Phalloidin, Alexa Fluor 488 Conjugate (Lonza Walkersville, Inc., Walkersville, USA) and examined under laser confocal microscope.
For osteogenic induction the full medium was further supplemented with 10 mM sodium β-glycerophosphate, 10 nM dexamethasone and 50 μg/ml l -ascorbic acid . This induction medium was changed twice a week for 2 more weeks.
Plain-gel constructs (with no cells) were prepared by incubating plain 0.5% PuraMatrix gel, of 40 μl each, in osteogenic induction medium for 2 weeks.
Transplantation study
Five 6-week-old immunocompromised nude mice were used because their immune system cannot recognise the transplanted cells as foreign bodies. Under anaesthesia, subcutaneous pockets were created on both sides of the animals in the flank region. The cell-gel constructs, two constructs with cells from patient 1 and three constructs with cells from patient 2, were placed into the pockets on the left side while the ones on the right received a plain-gel construct as the control. The animals were killed at week 4 post-transplantation. The transplanted sites were examined for remaining gel constructs and/or new tissue formation. Accordingly, tissue sample blocks were obtained with wide margins. All procedures were ethically approved by the University of Hong Kong Committee on the Use of Live Animals in Teaching and Research.
General observation
Retrieved samples were fixed with 4% paraformaldehyde in phosphate buffered saline for 2 days. To facilitate visual and radiographic examination, the tissue blocks were trimmed. Under microscopy, the retrieved gel constructs were located and excessive surrounding mouse tissue was removed. The general form and surface texture of the retrieved gel constructs was noted. Contact radiographs were taken to verify the extent of mineralisation in the tissue blocks.
Immunohistochemistry on tissue section
After gross examination, paraffin sections were prepared from the retrieved tissue blocks (with the transformed gel constructs in situ ). Routine haematoxylin and eosin (H–E) staining was applied to selected sections, saving the rest for immunohistochemical analysis. The presence of collagen type I, osteocalcin, osteopontin, osteonectin and parathyroid hormone receptor were evaluated by applying the corresponding anti-human antibody to the sections. Antigen–antibody bindings were visualised with 3,3′-diaminobenzidine (DAB) staining with avidin–biotin amplification. Briefly, antigens were retrieved by proteinase K treatment at 37 °C. Endogenous peroxidase was quenched by 3% H 2 O 2 for 20 min. Nonspecific binding was blocked by preincubation with 10% normal serum for 60 min. Sections were incubated with the unconjugated primary anti-human antibodies, mentioned above, in 2% blocking serum overnight. ABC Staining System (Santa Cruz Biotechnology, Inc.) was applied as recommended by the manufacturer for secondary antibody treatment and DAB staining. Sections were counterstained with haematoxylin, sealed, and analysed using light microscopy.
Results
When transferred into culture medium, the SAPN material set into a clear gel within 1 or 2 s. Initially, the encapsulated HDPCs were like tiny round peas evenly dispersed in the gel constructs. On the third day after encapsulation some HDPCs resumed their typical spindle-like appearance. By week 2 it was difficult to determine individual cells with phase contrast light microscopy. This was possibly due to increased cluttering or overshadowing of cells within the three-dimensional (3D) gel constructs. The built up of extracellular matrix might further jeopardise the optical performance of the phase contrast mechanism. Laser confocal images showed that the HDPCs were no longer homogenously distributed in the gel. The cells aligned and connected to form thread-like structures that were interwoven into a 3D configuration like a steel wool mop ( Fig. 2 A ).
By week 4, the appearance of the gel constructs under light microscopy was similar to looking up to see the sky from beneath a dense canopy. Adjacent to the gel constructs, large colonies of adherent cell spread out onto the culture-ware. Significant deposits had developed on these colonies. Positive Alizarin red staining ( Fig. 2 B) suggested these were calcium salt depositions.
Evidently the HDPCs survived encapsulation by the SAPN scaffolds. The HDPCs also migrated within, and out of, the SAPN scaffold without apparently damaging the scaffold.
In vivo observations
By postoperative week 4, no ulceration, no swelling, and no reddening or any superficial sign of adverse tissue reaction was observed at any transplanted site.
Transplant retrieval
Retrieval on the right side (the control group) revealed no sign of inflammation. The plain-gel constructs could not be found, it was presumed that they were all completely resorbed with no new tissue formation. As the location of the plain-gel constructs could not be determined ( Fig. 3 A ), no tissue sample was collected from the control group for further analysis.
On the left side (the experimental sites), the transplanted scaffolds were palpable through the animal’s skin. No sign of inflammation was noted during dissection. After trimming the tissue samples, the retrieved transplants showed up as small ivory coloured discoid structures. The original smooth surface of the cell-gel construct was transformed into a nodular surface. The overall appearance was like a small piece of ‘cookie’ wrapped in a thin translucent layer of loose connective tissue ( Fig. 3 B). A few blood vessels were observed on the outer layer of loose connective tissue. When all the soft tissues were rendered radiolucent on contact radiographs, by adjusting the X-ray intensity, multiple foci of mineralisation were seen ( Fig. 3 C).
On examining the H–E stained paraffin sections, no inflammatory infiltrate was observed in the tissues surrounding the retrieved transplanted scaffold. Regions of nuclear sparse extracellular matrix of lobular appearance were dispersed throughout the sections of the retrieved transplants ( Fig. 4 A ). These lobules of extracellular matrix seemed to coincide with the radio-opaque foci shown in the contact radiographs. The gaps between the lobules of extracellular matrix were filled by small amounts of tissue with higher nuclei density. At higher magnification, blood capillaries were readily found ( Fig. 4 B). The distinct outline of each extracellular matrix lobule was observable. The circumferential apposition of cells following the contour of the lobules was also noted ( Fig. 4 C). While the plain-gel constructs were completely resorbed, the HDPC seeded gel constructs were completely transformed into solid pieces of viable tissue packed with mineralised lobules.
Immunohistochemistry
Using immunostaining, positive expressions of parathyroid hormone receptor, osteopontin, osteocalcin, osteonectin and type I collagen were recorded. A large proportion of the tissues wedged between the lobules of the extracellular matrix were positively stained for parathyroid hormone receptor ( Fig. 5 ). Parathyroid hormone receptor was taken as a marker for osteoblast, which is responsible for matrix formation in bone.
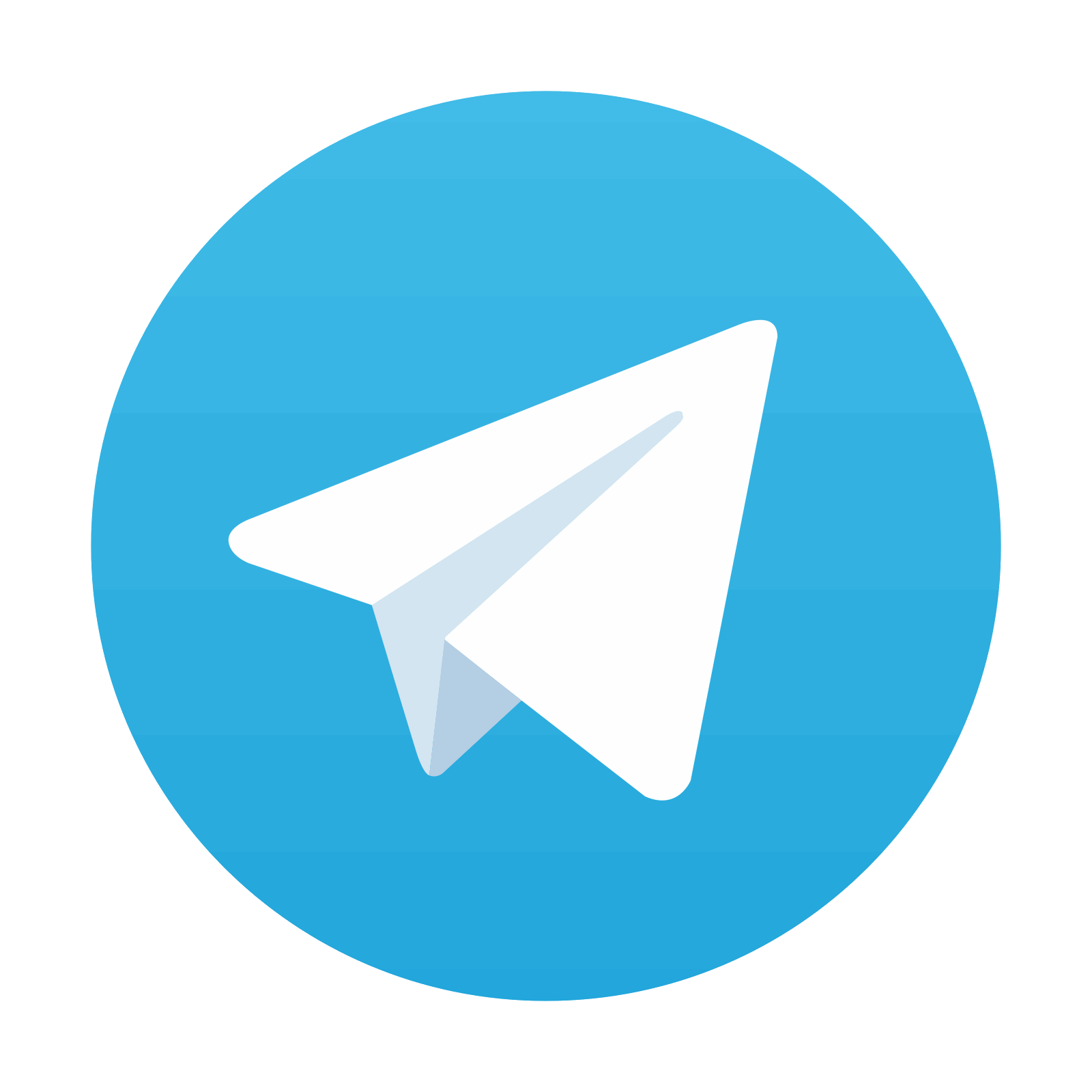
Stay updated, free dental videos. Join our Telegram channel

VIDEdental - Online dental courses
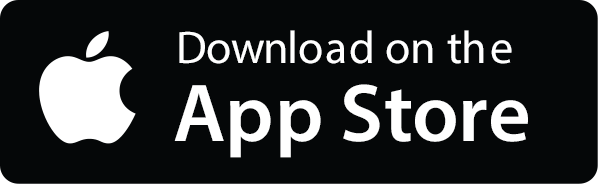
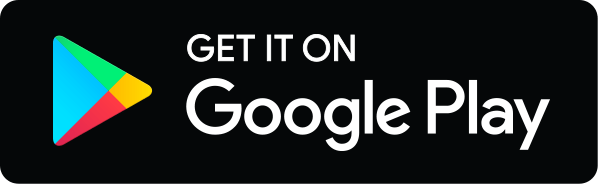
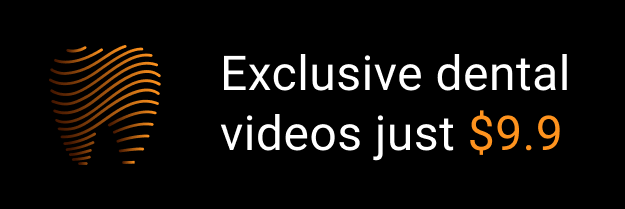