Introduction
Nickel-titanium closed-coil springs are purported to deliver constant forces over extended ranges of activation and working times. In-vivo studies supporting this claim are limited. The objective of this study was to evaluate changes in force-decay properties of nickel-titanium closed-coil springs after clinical use.
Methods
Pseudoelastic force-deflection curves for 30 nickel-titanium coil springs (used intraorally) and 15 matched laboratory control springs (simulated intraoral conditions: artificial saliva, 37°C) were tested before and after retrieval via dynamic mechanical analysis and a testing machine, respectively, to evaluate the amounts of force-loss and hysteresis change after 4, 8, or 12 weeks of working time (n = 10 per group). The effects of the oral environment and clinical use on force properties were evaluated by comparing in-vivo and in-vitro data.
Results
The springs studied showed a statistically significant decrease in force (approximately 12%) after 4 weeks of clinical use ( P <0.01), with a further significant decrease (approximately 7%) from 4 to 8 weeks ( P = 0.03), and force levels appearing to remain steady thereafter. Clinical space closure at an average rate of 0.91 mm per month was still observed despite this decrease in force. In-vivo and in-vitro force-loss data were not statistically different.
Conclusions
Nickel-titanium closed-coil springs do not deliver constant forces when used intraorally, but they still allow for space-closure rates of approximately 1 mm per month.
Space closure is an important aspect of orthodontic treatment. Using light, continuous forces over a relatively long activation range (eg, 5-10 mm of space closure) allows for more biologically favorable and clinically efficient tooth movement with fewer negative side effects. Some common orthodontic materials used for space closure deliver high initial forces that decay rapidly before reactivation. Nickel-titanium materials are purported to overcome this rapid force-decay problem and supply light, continuous forces over a long activation range. The claim that they can deliver these more biologically favorable forces, and thus potentially lead to more efficient tooth movement, is how many clinicians have justified their use, despite their relatively greater cost compared with other common space-closing materials such as elastomeric chains and closing loops.
Nickel-titanium alloys possess the unique properties of shape memory and superelasticity because of their capacity to alter their crystalline bonding patterns between the martensitic and austenitic phases as a function of temperature and applied stress without permanent dislocation of atoms. Thus, these materials exhibit a relatively flat (nonlinear) load-deflection curve signifying the superelastic characteristic of the nickel-titanium alloy in which it delivers a low, constant force over a long range of activation.
Unfortunately, several in-vitro laboratory studies and limited in-vivo studies have suggested that nickel-titanium closed-coil springs might not deliver constant forces. Maganzini et al examined 14 types of nickel-titanium closed-coil springs in vitro from 5 companies and concluded that most of the springs tested did not exhibit constant deactivation forces or physiologic peak load forces.
It is known that in-vivo clinical outcomes correlate poorly with in-vitro studies. Intraorally, plaque accumulation on appliances and the array of oral bacteria with their highly varied by-products create a unique environment that cannot be well simulated in the laboratory. Additionally, the mechanical properties of nickel-titanium products have been shown to highly depend on intraoral temperature changes. Eliades and Bourauel highlighted the need for postclinical retrieval analysis to fully understand the in-vivo material properties. Clinical data examining force losses in nickel-titanium coil springs are limited, but in-vivo results from Nightingale and Jones demonstrated an average force loss of 48% after 22 weeks of intraoral use. Our project aimed to evaluate the intrinsic force-decay properties of nickel-titanium closed-coil springs after clinical use, with the hypothesis that they will experience force decay proportional to stretch duration and thus affect the efficacy of space closure. Comparisons with matched laboratory analysis will elucidate the overall effect of the intraoral environment on spring properties.
Understanding the force-decay properties of nickel-titanium closed-coil springs during intraoral use will enable clinicians to make more cost-effective decisions and empower them to deliver more efficient and effective treatments.
Material and methods
Patients in active treatment at the University of North Carolina School of Dentistry graduate orthodontic clinic or the dental faculty practice who met the inclusion and exclusion criteria outlined below were eligible to participate in this study. After institutional review board approval (study10-1802) from the University of North Carolina, 11 patients were consecutively enrolled, and data were collected based on the following criteria: (1) they needed space-closure treatment, (2) space was closed with sliding mechanics using 150-g Sentalloy nickel-titanium coil springs (DENTSPLY GAC International, Bohemia, NY); and (3) they consented to participate in the study. The exclusion criterion was springs that showed permanent deformation before or at the removal stage.
All springs used in this study were Sentalloy closed-coil springs of medium grade that were advertised by the manufacturer to deliver a force of 150 g without deformation or force change when stretched in a range of 3 to 15 mm. These commercially available springs are approximately 9 mm long (eyelet to eyelet) and consist of a 3-mm length of coil with eyelets at each end. All springs used were from the same lot number (B3X0). A segment of coil spring was cut and used to run differential scanning calorimetric analysis (Q100; TA Instruments, New Castle, Del) to investigate the phase transformation of the nickel-titanium coil. The rate of temperature change was 1°C per minute.
Initial (before use) force levels for each spring were tested using dynamic mechanical analysis. It is a technique that incorporates stress-strain force measurements to study the mechanical properties of a material. Dynamic mechanical analysis was chosen for this study because it can accurately control temperature (±0.1°C) during force analysis and perform a load-controlled test. Ten springs were stretched during the preliminary testing, and pseudoelastic force-deflection curves were generated for each. Although there were small variations in force levels from each spring, the ideal testing force for the dynamic mechanical analysis was determined to be 300 g. At this force level, many preliminary springs were able to achieve almost the full 12-mm activation range recommended by the manufacturer; beyond this force level, many springs were stretched beyond the 12-mm guideline and possibly distorted. Although the manufacturer’s reported force level was 150 g, some springs reached 300 g at the stretch distance of clinical space closure. Testing the springs at 300 g allowed us to capture and characterize the entire range of loading and unloading force curves for these nickel-titanium coil springs. This amount of force did not introduce plastic deformation according to our force-deflection plots.
All 55 springs used in this study were tested on a dynamic mechanical analysis apparatus (model 2980, TA Instruments) at the constant force of 300 g ( Fig 1 , A ). Temperature was controlled using a combination of liquid nitrogen and thermal heating to maintain temperature at 37°C ± 0.1°C. Force was ramped up at a rate of 0.5 N per minute to 2.942 N (300 g) and then back down to zero at the same rate. Each spring was preloaded to 0.24 N (24 g) and maintained at the isothermal temperature of 37°C for 2 minutes before force ramping. Springs were attached to 0.032-in stainless steel hooks, which were gripped by the dynamic mechanical analysis film tension clamp ( Fig 1 , B ).
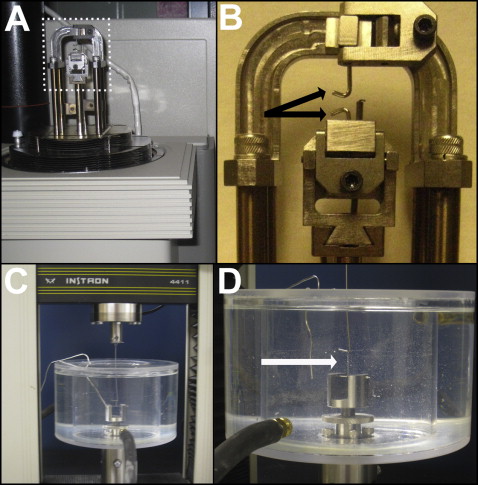
The final (after-use) testing of the springs was conducted on a universal testing machine (model 4411; Instron, Norwood, Mass) ( Fig 1 , C ), which uses a uniaxial load cell to measure forces and activations and generate load-deflection curves. The testing machine used displacement control rather than force control; it stretched each spring to the same length that it was activated in pretesting via the dynamic mechanical analysis. The springs were stretched to this length at a rate of 5 mm per minute and then allowed to return to their original relaxed state at the same rate. The testing machine was equipped with a ±500-N static load cell (serial number UK 27; Instron) attached to the crosshead. A 0.032-in stainless steel hook was attached to the load cell to hold the upper loop of the coil spring. The opposite end of the coil spring was held by a 0.032-in stainless steel rod, which was inserted in a special testing jig attached to the instrument base. The spring and the special jig were contained in a double-jacketed water chamber and submerged in water ( Fig 1 , D ). The temperature of the water in the inner bath (37°C ± 1°C) was maintained by circulating temperature-controlled water through the outer closed chamber. This temperature was carefully regulated by a water-circulating temperature controller (Haake; Thermo Electron, Karisruhe, Germany). The monitoring thermocouple for the water circulator was placed in the inner chamber. The springs were maintained in the water bath for 2 minutes before stretching.
After the dynamic mechanical analysis pretesting, the springs were randomly distributed to 3 groups: clinical, laboratory, and control.
For the clinical springs, 30 pretested springs were used during the treatment of patients in the orthodontic clinics at the University of North Carolina at Chapel Hill, from May to December 2011. Once a patient met the inclusion criteria, he or she was enrolled in the study, and initial data regarding space closure were gathered. The springs were attached from the canine hook to the molar hook and ligated to the canine hook to prevent loss ( Fig 2 ). Intraoral measurements were made for spring activation range and interdental spacing using a Boley gauge. In addition, bracket slot size, wire size, and wire material were recorded. Patients were seen on their normal recall schedule of 4 weeks. At each recall, the springs were checked for signs of obvious distortion and maintained undisturbed until collection if no distortion was noted. Ten springs were collected for each time point in the study (4, 8, and 12 weeks). Upon removal of the springs, final measurements regarding spring activation length and remaining space were recorded. The springs were cleaned in a 10% formalin solution for 10 minutes, rinsed with deionized water, and stored dry in plastic bags for final force testing on the Instron machine.
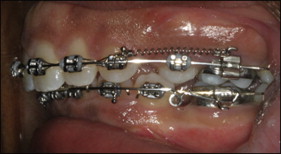
Depending on the patient’s space closure needs, up to 4 springs could be collected from the same patient at 1 time (1 per quadrant). If additional space closure was needed after retrieval of the springs, new springs could have been placed in the same patient and collected after another 4, 8, or 12 weeks. Overall, 11 patients (7 female, 4 male) participated in this prospective study (average age, 23.2 years; range, 13-43 years). The slot size and type of archwire used for each clinical spring are summarized in Table I .
Time (wk) | Mean force loss (g) | SD | Force loss (%) | P value | |
---|---|---|---|---|---|
Control | 0 (n = 10) | 5.12 | 3.53 | 1.71 | <0.01 |
Clinical | 4 (n = 10) | 34.69 | 19.99 | 11.57 | <0.01 |
8 (n = 10) | 56.59 | 18.93 | 18.88 | <0.01 | |
12 (n = 10) | 53.33 | 19.10 | 17.79 | <0.01 | |
Laboratory | 4 (n = 13) | 36.32 | 11.52 | 12.12 | <0.01 |
8 (n = 13) | 52.02 | 15.58 | 17.36 | <0.01 | |
12 (n = 13) | 58.27 | 23.60 | 19.44 | <0.01 |
In the laboratory springs, 15 pretested springs were placed on stainless steel plates with stainless steel attachment pegs set 20 mm apart; this correlates to 11 mm of coil activation. The overall distance between the 2 posts was 20 mm. The eyelets at the ends of the springs were 3 mm each; they consumed 6 mm of space. In addition, the diameter of the eyelets was 1.5 mm (ie, slop in the eyelet holes), which needs to be overcome before the springs are activated. This leads to 20 – 6 (eyelets) – 3 mm (slop in the eyelet holes) = 11 mm of activation. These springs were stored stretched in the laboratory in a salivary substitute material at 37°C and tested at time intervals of 4, 8, and 12 weeks on the Instron universal testing machine. The salivary substitute material used was Fusayama Meyer artificial saliva. The composition of this solution, which closely resembles natural saliva, is potassium chloride (0.4 g/L), sodium chloride (0.4 g/L), CaCl 2 ·2H 2 O (0.906 g/L), NaH 2 PO 4 ·2H 2 O (0.690 g/L), Na 2 S·9H 2 O (0.005 g/L), and urea (1 g/L). The solution was titrated to a pH of 6.5 using 5 mol/L of sodium hydroxide.
For the control springs, 10 pretested springs were analyzed on the Instron universal testing machine to identify the relationship between the 2 machines used for pretesting and posttesting.
Statistical analysis
Initial and final pseudoelastic force-deflection curves were generated for each spring in the clinical, laboratory, and control groups. For all mechanical testing, each spring was stretched to approximately the same length for its posttesting on the Instron machine as it was for its pretesting in the dynamic mechanical analysis. The maximum force attained by each spring at this length was used to compare the amount of force loss (force loss = maximum force reached by the spring at a given length before use – maximum force reached by the same spring at that same length after use).
At each time point, the loss in force was assessed using a 1-sample t test to determine whether the mean loss was statistically significant. Unpaired t tests were used to compare the control group’s force loss with the 4-week clinical data and separately to the 4-week laboratory data to evaluate for statistical significance beyond what was due to differences between the 2 machines used for testing. One-way analysis of variance (ANOVA) was used to evaluate force-loss differences between time points for the clinical springs, which had 3 mutually exclusive groups. Force-loss differences between time points for the laboratory springs were evaluated using repeated-measures ANOVA. Unpaired t tests were used to compare force losses for the clinical groups at each time period with the laboratory values over that same period. A linear regression model was performed to identify associations between the outcome variable of space closure per week and the predictor variables of sex, age, archwire type, slot size, initial coil stretch length, and individual coil stiffness (calculated by dividing the initial maximum force by the activation length of that spring).
Results
Space closure was carried out on 0.018-in slot appliances (6 springs) and 0.022-in slot appliances (24 springs) in addition to different wire dimensions: 18 stainless steel archwires (7 springs), 0.018 × 0.025-in stainless steel archwires (16 springs), and 0.016 × 0.022-in stainless steel archwires (7 springs).
No clinical spring showed signs of distortion upon retrieval, so all 30 (10 per time point) were included in the analysis. During testing with the Instron machine at 4 weeks, 2 laboratory springs became distorted because of machine malfunctions and were not used for analysis, leaving 13 laboratory springs for analysis. All 10 control springs were used for analysis.
The initial and final pseudoelastic force-deflection curves for representative springs from the control, 4-week, 8-week, and 12-week groups are shown in Figure 3 . The curves show a nearly flat plateau of constant force indicating a stress-induced martensite transformation during loading. Interestingly, the differential scanning calorimeter graph confirms that nickel-titanium springs have a phase transformation at oral temperatures ( Fig 4 ).
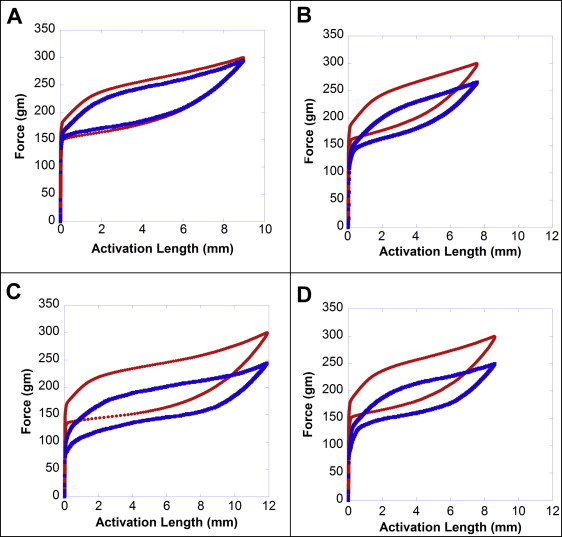
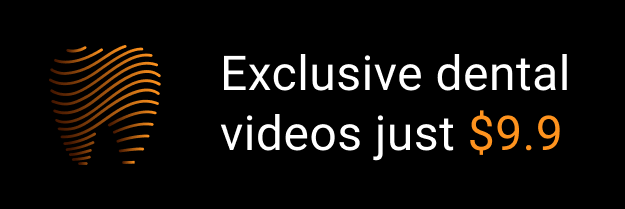