Introduction
The aim of this study was to assess the effect of intraoral aging on the setting status of a resin composite and a glass ionomer adhesive, relative to control specimens stored in water.
Methods
Metallic brackets were bonded with resin composite orthodontic adhesive (Transbond XT; 3M Unitek, Monrovia, Calif) or a glass ionomer cement (Fuji I; GC, Tokyo, Japan) to recently extracted premolars and kept in water for 6 months. The same materials were also bonded to the premolars of orthodontic patients. After 6 months, the teeth were carefully extracted, with the brackets intact on their buccal surfaces. All teeth were embedded in epoxy resin and sectioned buccolingually. Fourier transform infrared microscopy and Raman microscopy were used for the estimation of the degree of cure in the composite and the salt yield in the glass ionomer adhesives.
Results
The control samples of the composite showed significantly lower degrees of cure than did the retrieved specimens (52.40% ± 3.21% vs 57.62% ± 1.32% by Fourier transform infrared microscopy, and 61.40% ± 2.61% vs 67.40% ± 3.44% by Raman microscopy). Raman microscopy significantly overestimated the degree of cure and failed to provide reliable information for the salt yield in the glass ionomer cement. Fourier transform infrared microscopy showed increased, but no statistically significant difference in, aluminum-carboxylate salts in the retrieved specimens.
Conclusions
Enhanced oxidation of residual carbon-carbon bonds in the composite and slightly increased dissolution of the weaker calcium-salt phase in the glass ionomer cement were the main differences in the intraorally aged specimens in comparison with the specimens stored in water.
Despite many studies from orthodontic materials research, the laboratory configurations and setups used for exposing the materials to water and various electrolytes fail to simulate the actual oral environment, which includes saliva, pH fluctuation, microbial attack, complex cyclic loading, and enzymatic degradation. Since the intraoral environment is a complex milieu capable of affecting the physical and mechanical properties of an adhesive, laboratory data should be carefully interpreted. Thus, there is a notable lack of evidence regarding the intraoral aging pattern of orthodontic materials and the associated phenomena, such as surface alterations, structural changes, and variations of mechanical properties. The intraoral exposure might affect the longevity of the adhesive (eg, aging of polymeric adhesives) by modification of structural qualities.
The traditional orthodontic adhesives for bracket bonding are resin composite materials, introduced in the mid-1970s. These orthodontic adhesives have been used successfully on acid-etched enamel over the last 40 years. The development of visible light-cured materials offered additional advantages in the field—ie, high early bond strength, minimal oxygen inhibition, and prolonged working time for optimal bracket placement—which have brought innovation and practicality to modern orthodontic treatment. Disadvantages of the resin composite adhesives include loss of enamel during acid conditioning, loss of the superficial fluoride-rich enamel zone during debonding, and demineralization of enamel around the brackets because of poor patient compliance with respect to oral hygiene. The importance of the curing efficiency on the performance of resin composites has long been documented. It has been shown that the degree of cure (%DC, the amount of carbon-carbon bonds reacted after setting) of resin composites modulates the composite’s physical and mechanical properties, color stability, solubility and degradation, and biocompatibility.
Glass ionomer cements possess unique benefits that make them useful in clinical orthodontics. They chemically adhere to both enamel and metal, release fluoride with its known caries-inhibiting properties, and can absorb fluoride from sources such as fluoride solutions, thus recharging their fluoride reservoir. Furthermore, acid conditioning is not required, so that both bonding and debonding procedures can reduce iatrogenic damage to enamel, and the bonding field does not have to be dry, thus increasing the speed and ease of bracket placement. But it is a concern that bond strengths for glass ionomer cements are not of the same magnitude as those for resin composite adhesives; as a result, the failure rate of brackets attached to teeth is higher. To improve bracket cementation, resin-modified glass ionomers were introduced.
Because the phenomena accompanying intraoral aging of the material might not be reflected in short-term or long-term performance of the adhesives under laboratory conditions, intraoral aging might modify the critical properties of orthodontic adhesives. The purpose of this study was to assess the effect of 6 months of intraoral aging on the setting efficiency of resin composite and conventional glass ionomer orthodontic adhesives relative to control specimens stored in water. The null hypothesis tested was that there is no difference in the setting status between laboratory and intraorally aged resin composite and glass ionomer orthodontic adhesives.
Material and methods
The control sample consisted of 14 sound premolars, recently extracted for orthodontic reasons and kept for 1 week in distilled water with the addition of 0.5% sodium azide at 8°C.
The teeth were randomly classified into 2 groups of 7 specimens each, cleaned and polished with a nonfluoride paste (Clean Polish; Hawe-Neos Dental, Bioggio, Switzerland). Metallic brackets (Mini Sprint; Forestadent, Pforzheim, Germany) were bonded to the middle buccal enamel surfaces with a conventional glass ionomer cement (Fuji I; GC, Tokyo, Japan) or a composite resin adhesive (Transbond XT; 3M Unitek, Monrovia, Calif). Glass ionomer cement was placed directly on blot-dried enamel. The resin composite adhesive, used without primer or bonding resin, was applied on air-dried, acid-etched (for 30 seconds) enamel with a 35% phosphoric acid gel (Transbond XT etching gel, 3M Unitek) and photopolymerized from the incisal and cervical bracket edges (10 seconds each) with a halogen light-curing unit (850 mW/cm 2 standard light intensity, Trilight; 3M ESPE, Seefeld, Germany). The tip of the unit was placed in contact with the bracket edges at approximately a 45° angle. All teeth were stored in 200 mL of distilled water at 37°C for 6 months. The water was replaced once a month to prevent saturation from leachable components.
The test sample consisted of specimens obtained from patients recruited in the Graduate Orthodontic Clinic of the Aristotle University of Thessaloniki, Thessaloniki, Greece, with an age range of 13 to 36 years (mean, 22 years); 55% were male, and 45% were female. Briefly, the patients had been selected using the following selection criteria: need for extractions for orthodontic purposes of 2 or 4 premolars, intact premolar buccal surfaces, and no previous glass ionomer restorative fillings in the oral cavity. The original protocol had been approved by the ethical committee of the university (14/18.07.2005); after detailed explanations of the purpose of this research and the clinical procedures, consent had been obtained. Furthermore, no orthodontic treatment had been started before the extractions and 6 months after bonding with the adhesives to be tested. The patients and the buccal premolar surfaces had been randomly classified (numbers 1-7 for patients; letters C [composite] or G [glass ionomer] for tooth surfaces) in 7 contralateral pairs (total of 14 teeth) to receive sequentially alternating resin composite and glass ionomer adhesives. The tooth surfaces had been cleaned and polished with the nonfluoride paste. The same brand of metallic brackets had been bonded with either the conventional glass ionomer cement (Fuji I) or the composite resin (Transbond XT) as reported before. After 6 months, the teeth were carefully extracted, with the brackets intact on their buccal surfaces, and thoroughly rinsed with distilled water.
The roots of all teeth were removed by a horizontal section 1 mm below the cervical enamel margins, and the crowns were embedded in epoxy resin (CaldoFix; Struers A/S, Ballerup, Denmark) with the proximal surfaces parallel to the horizontal plane. The specimens were sectioned buccolingually using a microtome under continuous coolant (Isomet; Buehler, Lake Bluff, Ill). The sections were then polished with silicon carbide papers (600-1200 grit sizes) under water coolant and ultrasonicated in distilled water for 2 minutes to remove the surface smears ( Fig 1 ). The specimens were stored in a desiccator for 5 days to remove loosely bound water and then studied by Fourier transform infrared microscopy (FTIRM) and Raman microscopy (RM).
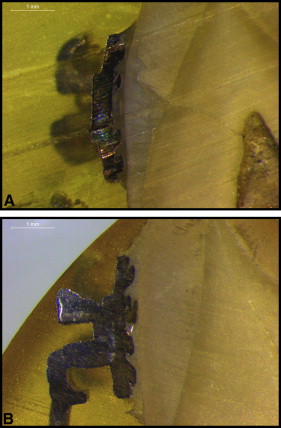
FTIRM was performed with a microscope (AutoImage; Perkin-Elmer, Bacon, United Kingdom) equipped with a germanium micro-attenuated total reflectance objective and attached to a Fourier transform infrared spectrometer (Spectrum GX; Perkin Elmer). Spectra were acquired at 4 points per specimen (2 in the bulk adhesive, 2 at the margins) under the following conditions: mercury cadmium telluride detector cooled by liquid nitrogen, 4000 to 750 cm −1 wave number range, 4 cm −1 resolution, 50-μm-diameter sampling window (attenuated total reflectance objective), 128 spectra coaddition, and 1-μm depth of analysis at 1000 cm −1 . All spectra were subjected to baseline and attenuated total reflectance corrections. Spectra of the resin composite paste and the glass ionomer mix before setting were used as references of the unset materials.
RM was performed by a Raman spectrometer (EZRaman I; Enwave Optronics, Irvine, Calif) attached to a microscope (MBE; Leica Microsystems, Wetzlar, Germany) under the following conditions: 785 nm laser excitation, 40-times magnification, 100 to 200 mW nominal power, 1.5-mm working distance, 2000 to 200 cm −1 wave number range, and 5 cm −1 resolution. Four regions were analyzed per specimen as above.
For the resin composite adhesive, the percentage degree of cure (%DC) after storage was assessed on a relative basis by the 2-band method and the tangent baseline technique. The aliphatic (C = C) bond stretching vibrations at 1638 cm −1 were chosen as the analytical band, whereas the aromatic (C…C) bond-stretching vibrations at 1605 cm −1 , which are unaffected by the polymerization reaction, were chosen as the reference band. The %DC was calculated according to the equation: %DC = 100 × [1 − (A/B × C/D)], where A is the net peak absorbance height of the polymerized material at 1638 cm −1 , B is the absorbance height of the unpolymerized material at 1638 cm −1 , C is the absorbance height of the unpolymerized material at 1605 cm −1 , and D is the absorbance height of the polymerized material at 1605 cm −1 . The %DC values for each specimen were the averages of the 4 measurements.
For the glass ionomer cement, the following peaks were used to monitor the acid-base reaction: the carboxyl peaks of polyacrylic acid (1740 cm −1 ) and tartaric acid (1730 cm −1 ), and the peaks of calcium polyacrylate (1540 cm −1 ), aluminum polyacrylate (1610 cm −1 ), calcium tartrate (1595 cm −1 ), and aluminum tartrate (1670 cm −1 ). Because the carboxyl groups are completely neutralized during the early setting stages of conventional glass ionomers, the salt yield was calculated by measuring the ratio of the peak areas of aluminum salts (1700-1600 cm −1 ) to calcium salts (1600-1500 cm −1 ) using the tangent baseline technique and the same analysis mode as above (4 regions per specimen).
The results of the %DC were statistically analyzed with 2-way analysis of variance (ANOVA) (α = 0.05) with analytical method and storage conditions as independent parameters. Statistical analysis of the salt ratio values was assessed by a t test.
Results
Representative FTIRM spectra of the resin composite adhesive are shown in Figure 2 . All the set specimens demonstrated a reduction in the peak high at the 1638 cm −1 band (C = C stretching vibrations) because of the conversion (C = C → C − C) after light curing. The spectra profiles were similar, except for a slight reduction of the C = C peak in intraorally exposed specimens. The RM spectra of the resin composite adhesive clearly demonstrated the characteristic bands (C = C stretching vibrations 1638 cm −1 and aromatic C…C stretching vibrations 1605 cm −1 ) used for the calculation of the %DC as well ( Fig 3 ).
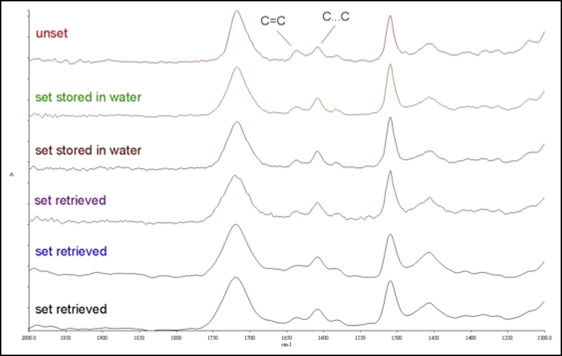
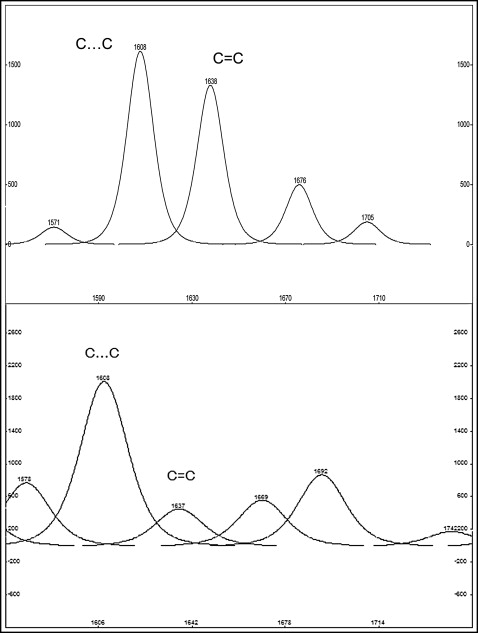
The results of the %DC after the FTIRM and RM analyses are presented in Table I . Statistical analysis of the %DC results by 2-way ANOVA showed significant differences in analytical method and storage conditions ( P <0.001), with no interaction between them ( P = 0.757). RM showed significantly higher %DC values for both storage conditions than did FTIRM ( P <0.001). Within the same analytical technique, the retrieved specimens showed significantly higher %DC than did those stored in water ( P = 0.009 for FTIRM; P = 0.004 for RM). In 2 of the 7 retrieved specimens, FTIRM analysis of the adhesive at the outer 50-μm regions showed the maximum %DC values.
%DC, FTIRM | %DC, RM | |
---|---|---|
Stored in water (6 mo, 37°C) |
52.4 (3.2) 1,a 42.0 (min)-57.1 (max) |
61.4 (2.6) 3,b 59.9 (min)-64.1 (max) |
Retrieved | 57.6 (1.3) 2,c 56.2 (min)-59.4 (max) |
67.4 (3.4) 4,d 64.0 (min)-72.3 (max) |
FTIRM spectra of the glass ionomer cement are illustrated in Figure 4 . The characteristic broad carboxylic peak of the unset material (1730 cm −1 ) disappeared after setting, and new peaks appeared assigned to calcium polyacrylate (1550-1520 cm −1 and 1410-1390 cm −1 ), aluminum polyacrylate (1620-1580 cm −1 and 1470-1430 cm −1 ), calcium tartrate (1630-1600 cm −1 ), and aluminum tartrate (1670-1650 cm −1 ). The contribution of water (1642 cm −1 ) was greater in some water-stored specimens in comparison with the retrieved ones. Because of the shape complexity and partial overlapping of the peak regions, the peak area ratios (aluminum salts/calcium salts) instead of the sum of the absolute area values were used.
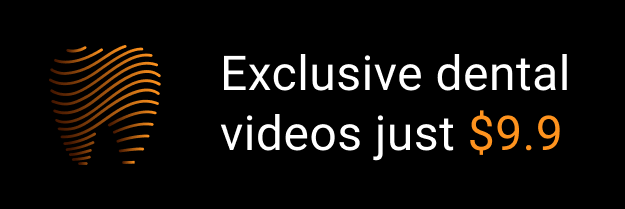