Introduction
White-spot lesions (WSL) might be susceptible to mechanical damage during orthodontic bracket and adhesive removal. The aims of this in-vitro study were to investigate enamel loss on bracket and adhesive removal when the brackets were surrounded by WSL and to determine the effect of remineralizing these lesions with a 1% (w/v) casein phosphopeptide amorphous calcium fluoride phosphate (CPP-ACFP) solution before bracket and adhesive removal.
Methods
Precoated metal mandibular incisor brackets were centrally bonded onto polished third molars and WSL produced by exposure to a demineralization buffer for 4, 12, and 30 days (n = 20 per group). Half of the demineralized window was covered with acid-resistant nail varnish, and the specimens were then subjected to remineralization with 1% CPP-ACFP. Brackets and residual adhesive were removed, and enamel damage was assessed by digital photography, profilometry, and scanning electron microscopy. Lesion depth, mineral loss, and remineralization were measured by transverse microradiography.
Results
WSL enamel around the bracket was more susceptible to iatrogenic damage at adhesive removal compared with sound enamel. Remineralization of lesions with 1% CPP-ACFP before adhesive removal significantly ( P <0.002) reduced the area and depth of damage.
Conclusions
Remineralizing WSLs with CPP-ACFP before adhesive removal reduced iatrogenic enamel damage.
Enamel mineral loss occurs over a continuum of severity with early clinical presentation as a white-spot lesion (WSL). It is termed WSL because of the change in the refractive index of the enamel, causing scattering of light and giving the enamel a chalky, opaque appearance. It has been reported that between 2% and 96% of all orthodontic patients develop WSLs during their treatment, and their presence can be detected as early as 4 weeks into orthodontic treatment.
The increased prevalence of WSLs in orthodontic patients can be attributed to the following factors. First, fixed orthodontic appliances form a niche for plaque to inhabit, evading regular oral hygiene practices. Second, residual adhesive surrounding orthodontic brackets provides a roughened surface that allows bacterial adherence. Third, the newly erupted teeth seen in typical orthodontic patients might also be more susceptible to acid attack.
With every incidence of pumicing, acid etching, debonding, or remnant cleanup, a small amount of enamel is likely to be removed. This depends on many factors: type of bonding system, adhesive material, debonding technique, and presence of enamel demineralization. Researchers have found that removing residual adhesive with a slow-speed tungsten carbide bur can produce an average defect from 3.0 to 50.5 μm deep in sound enamel. Limited research has investigated the effect of adhesive removal from demineralized enamel. No study to date has examined the impact of WSL severity on iatrogenic enamel damage during removal of orthodontic brackets and adhesive, nor has the effect of remineralizing these lesions before adhesive removal been studied.
During orthodontic treatment, if caries-active patients are identified, the aim should always be to institute preventive measures to interrupt the dental caries process. However, practitioners often find themselves actively trying to remineralize WSLs after orthodontic treatment. Although natural lesion regression has been shown by quantitative light-induced fluorescence 6 months after debanding, the overall regressions were small, and some lesions still progressed to cavitation. Casein phosphopeptide stabilized amorphous calcium phosphate complexes (CPP-ACP), the remineralizing agents in Tooth Mousse (also known as MI Paste) (GC Corporation, Tokyo, Japan), have been shown to enhance WSL regressions in postorthodontic patients. The main benefits of CPP-ACP are their ability to localize at the tooth’s surface and incorporate into supragingival plaque to provide bioavailable calcium and phosphate ions where they are most needed.
Recently, fluoride has been incorporated into the CPP-ACP complex (CPP-ACFP) and was shown to promote remineralization; the deposited mineral is consistent with fluorapatite. This produced levels of remineralization of enamel subsurface lesions of up to 57%. This level of remineralization was able to return translucency to the treated enamel to that of sound enamel, in agreement with the clinical findings of others.
The aims of this in-vitro study were to investigate enamel loss at bracket and adhesive removal when the orthodontic brackets were surrounded by WSLs of varying depths and degrees of mineral loss, and to determine the effect on enamel loss when these lesions where remineralized with 1% (w/v) CPP-ACFP solution before bracket and adhesive removal. The hypothesis was that white spots would be more susceptible than sound enamel to damage during bracket and adhesive removal, and that remineralization would reduce this damage.
Material and methods
Ethics approval for this study was received from the Departmental Human Ethics Advisory Group of the University of Melbourne (DHEAG no: 0602917). Seventy-five third molars from a community with fluoridated water were collected. All teeth were stored in 10% neutral (pH, 7.0) phosphate-buffered formalin solution for a minimum of 2 weeks. The enamel specimens were scrutinized at 3 times for exclusion criteria: dental caries, dental fluorosis or other hypomineralized lesions, extraction damage, or pitting and cracks. All suitable teeth were rinsed and stored in double deionized water (MilliQ, Millipore, Billerica, Mass) before experimental use. The outer enamel surface was polished wet to an optical luster and mirror finish by using Sof-Lex discs (3M, St Paul, Minn) attached to a slow-speed contra-angle dental hand piece to remove the potentially fluoride-rich layer while maintaining adequate enamel thickness for WSL formation. Sixty-five teeth were hemi-sected into buccal or lingual enamel specimens with a water-cooled diamond blade saw (Minitom, Struers, Copenhagen, Denmark). The coronal pulp chambers were extirpated with a slow-speed bur in a contra-angle hand piece. Each enamel specimen had a precoated orthodontic mandibular incisor bracket (APC II, Victory Series, 3M Unitek, Monrovia, Calif) bonded in the center of the crown according to the manufacturer’s instructions by using Transbond Plus Self Etching primer (3M Unitek). Any visible excess adhesive was removed before light curing for 40 seconds with an Ortholux LED curing light (3M Unitek). Care was taken to review the surrounding enamel for bond or adhesive flash, which, if present, was removed with Sof-Lex discs. Acid-resistant red nail varnish (Revlon, New York, NY) was applied to the whole tooth, leaving a 1-mm window of exposed enamel surrounding the orthodontic bracket, and allowed to dry overnight. The specimens were randomly assigned to 4 groups. Group A was the control (n = 5). Groups B, C, and D were the experimental groups with 20 teeth in each. The 10 remaining teeth were further prepared into enamel slabs suitable for transverse microradiography analysis (TMR) as described by Cochrane et al and assigned to group B (n = 4), group C (n = 3), and group D (n = 3). A strip of Transbond adhesive (3M Unitek) was bonded across the enamel slab in a mesiodistal direction. All experimental specimens were then mounted inverted on sticky wax stubs and secured to the internal surface of plastic specimen jar lid for subsurface lesion formation.
Subsurface lesions were created in the exposed enamel windows according to the modified method of White. The demineralization solution contained 80 mL per liter of Good-Rite K-702 polyacrylate solution (Lubrizol Advanced Materials, Cleveland, Ohio), 500 mg per liter of hydroxyapatite (Bio-Gel, HTP, Bio-Rad Laboratories, Richmond, Calif), and 0.1 mole per liter of lactic acid (Ajax Chemicals, Auburn, New South Wales, Australia) at pH 4.8. All specimens from groups B through D were immersed in 40 mL of the demineralization solution for 4, 12, and 30 days, respectively, at 37°C, with the solution changed every 48 hours. Fresh demineralization buffer was made every 5 days. After subsurface lesion formation, all enamel specimens were rinsed with Milli-Q water and stored in a humidified environment. Nail varnish was applied to a randomly assigned side (left or right) to isolate the underlying demineralized enamel. The specimens of groups B through D were then immersed in 2 mL of 1% (w/v) CPP-ACFP with 0.05% sodium azide remineralizing solution at pH 5.5 for 30 days at 37°C. The remineralizing solution was changed every 4 days, with fresh solution prepared every 10 days. After the remineralization phase was completed, the red nail varnish was carefully removed with a scalpel blade (#23 carbon steel surgical blade, Swann-Morton, Sheffield, England), and each specimen was dried for 5 seconds with a triplex air syringe and placed on a standardized incandescent lighting stage for photography (number 1 PF207E 275W 240-250V photoflood lamps, Philips, Eindhoven, the Netherlands). A digital image was taken of each sample (EOS-20D [Canon, Tokyo, Japan], 1:1 magnification, ISO 200, tungsten setting, aperture f-stop 14:8) with a ruler and saved as a 72-pixel-per-in resolution JPEG file. The orthodontic bracket was debonded according to the manufacturer’s instructions with the recommended debonding pliers (3M Unitek) and photographed. A new slow-speed dome fissure tungsten carbide bur (Dental Logistics, Melbourne, Australia) in a contra-angle hand piece was used to remove residual orthodontic adhesive after randomization and blinding in 1 session and photographed.
A macro was created (Adobe Photoshop CS3, version 10.0.1, Adobe Systems, San Jose, Calif) to crop and adjust each image in a standardized fashion. Brightness (–15) and contrast (+100) were altered to enhance the contrast of the image. The images’ histogram profiles were “clipped” (70 to 210) to reduce glare and then viewed with magnification. The adhesive remnant index (ARI) and the area of damage were calculated after bracket and adhesive removal by using imaging software (Image J freeware). The scale was calibrated for each image by using the ruler, and damage was circumscribed and measured. All teeth underwent a white-light profilometry line scan through areas of enamel damage to obtain a depth measurement (300-μm z-axis depth probe, AltiSurf 500, Cotec-CCA, Thonon-les-bains, France; 30 Hz speed scan at 50 μm/s). Each line scan was leveled, and the step distance measurement was recorded (PaperMap 3.1, Cotec-CCA). A representative tooth from each group was gold sputter-coated (DynaVac Mini Sputter coater, Melbourne, Australia) and examined by using a scanning electron microscope (FEI Quanta 200 FEG, Brno, Czech Republic; 5.0-10.0 kV), under low (40 times) and high (600 and 3000 times) magnification to obtain secondary electron images.
Ten slabs prepared for TMR underwent the same demineralization regimen as did their parent group, after which they were hemi-sected. The left half was stored in an Eppendorf tube in a humidified environment, and the right half was subjected to the remineralizing regimen as above. The TMR enamel slabs were then dehydrated and embedded in methylmethacrylate resin (Paladur, Kulzer, Hanau, Germany). Four 200 to 300 μm sections perpendicular to the window surface were sectioned by using an internal annulus saw microtome (1600, Ernst Leitz, Wetzlar, Germany), lapped to 100 μm and radiographed along with an aluminum step wedge of 10 × 14 μm thick increments by using high-resolution glass plates (Microchrome, San Jose, Calif) and nickel-filtered copper Kα radiation (20 kV, 10 mA). After image analysis, the lesion depth was measured (μm), and the mineral content (vol %) profile of the demineralized and remineralized lesions were compared with the median sound enamel profile of the same section. The difference between the areas under the densitometric profile of the demineralized lesion and the median sound enamel, calculated by trapezoidal integration, is represented by ΔZd.The difference between the areas under the densitometric profile of the remineralized lesion and the median sound enamel, calculated by trapezoidal integration, is represented by ΔZr. The amount of mineral deposited in the lesion by remineralization is ΔZd-ΔZr is vol % mineral μm. These parameters were also converted to percentage change values after remineralization; percent remineralization ( %R ) represents the percentage of change in ΔZ values:
Statistical analysis
A power calculation was used to determine group sizes, by using values of demineralized enamel damage and levels of enamel remineralization obtained previously with a CPP-ACFP solution. Descriptive statistics including means and standard deviations were calculated for each group by using a statistical software package (version 16.0 for Windows, SPSS, Chicago, Ill). All data were examined for normality by using the Kolmogorov-Smirnov test. Nonnormal profilometry and damage area data were analyzed by using nonparametric Kruskal-Wallis and Mann-Whitney U tests. For comparisons between groups, a Bonferroni adjustment was made (α = 0.008). The TMR data were normally distributed and analyzed by using a 1-way classification analysis of variance (ANOVA) with the Tukey post-hoc test (α = 0.05). A paired t test was used to compare remineralization with demineralization areas and depth damage in each group.
Results
Five types of quantitative data were obtained from the experiment: ARI, area of damage from photographic analysis (% of window), depth of damage from white-light profilometry (μm), %R, and lesion depth (μm) from TMR. The study results are presented in Tables I to III .
Group | Lesion depth (μm) | ΔZd | ΔZd-ΔZr | ||
---|---|---|---|---|---|
Demin | Remin | (vol % min.μm) | (vol % min.μm) | %R | |
A | 0 ± 0 a | 0 ± 0 a | 0 ± 0 a | 0 ± 0 a | 0.0 ± 0.0 b,c,d |
B | 118 ± 14 a | 117 ± 12 a | 4839 ± 854 a | 2907 ± 580 a | 60.1 ± 5.8 b,d |
C | 226 ± 13 a | 214 ± 11 a | 10796 ± 1179 a | 6664 ± 1066 a | 61.7 ± 5.6 c |
D | 432 ± 22 a | 422 ± 24 a | 22272 ± 2145 a | 9589 ± 1898 a | 43.0 ± 6.5 b,c,d |
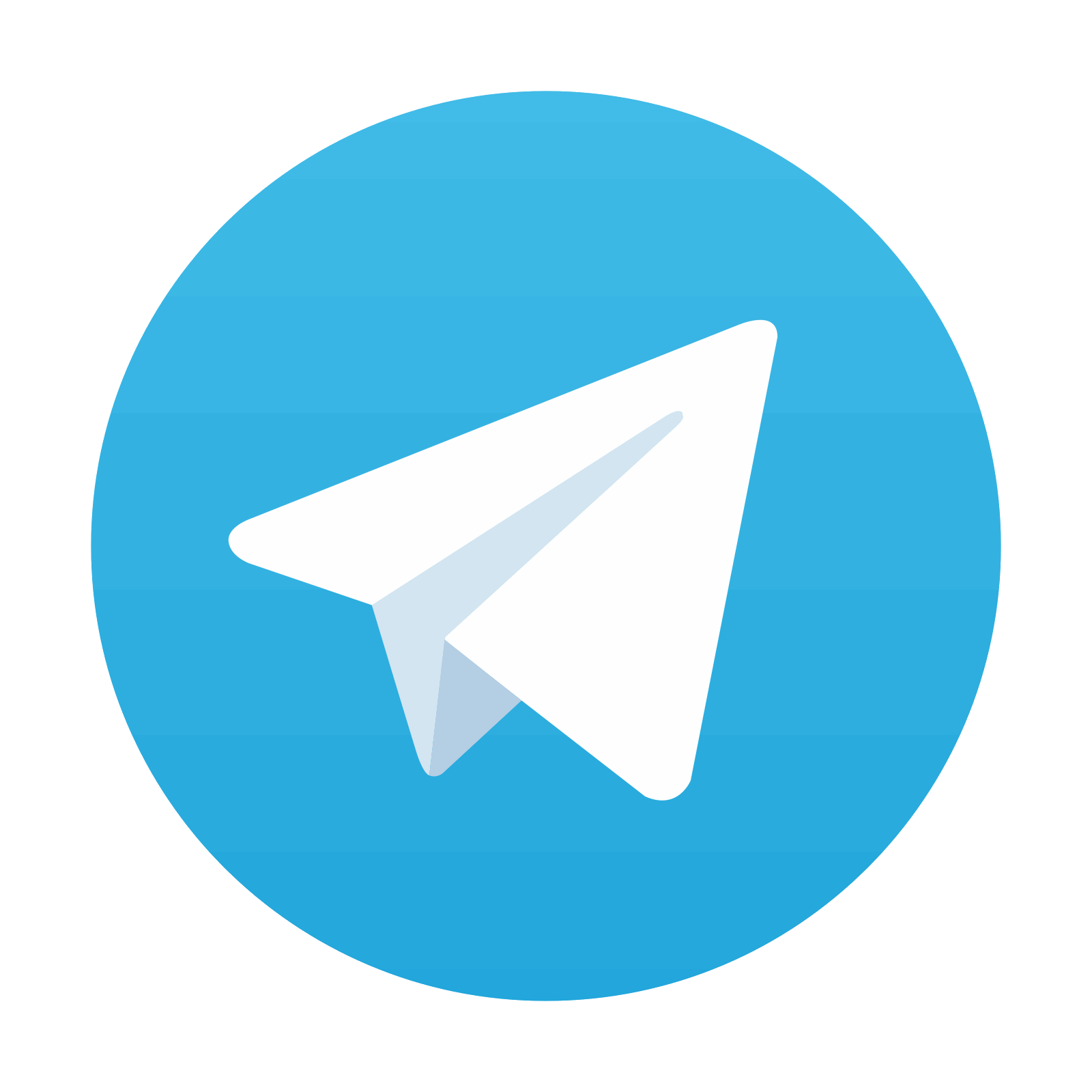
Stay updated, free dental videos. Join our Telegram channel

VIDEdental - Online dental courses
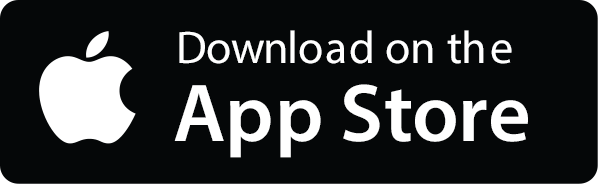
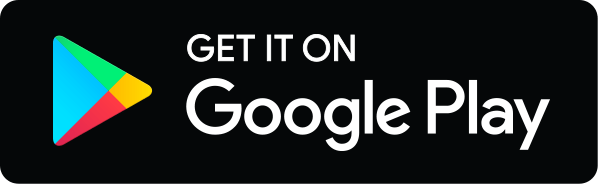
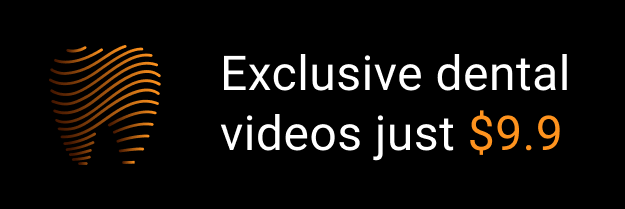