Introduction
Several metals undergo redox cycling, producing free radicals and generating oxidative stress. The purpose of this study was to investigate in-vitro oxidative stress of orthodontic archwires made of various alloys.
Methods
Mouse fibroblast cells L929 were exposed to 6 types of archwires, and the concentration of the oxidative stress marker 8-hydroxy-2′-deoxyguanosine in DNA was evaluated. Trypan blue dye was used in the determination of cell viability and numbers.
Results
Standard nickel-titanium archwires generated the highest oxidative stress, significantly higher than all other wires and the controls ( P <0.05), and coated nickel-titanium, copper-nickel-titanium, and cobalt-chromium were lower than nickel-titanium ( P <0.05), but higher than titanium-molybdenum and the negative and absolute controls ( P <0.05). Titanium-molybdenum and stainless steel generated the lowest stress. Nickel-titanium induced the lowest viability, lower than the negative and absolute controls and all other wires ( P <0.05) except titanium-molybdenum. Stainless steel showed the highest viability. Nickel-titanium produced the highest inhibition of cell growth, higher than all samples ( P <0.05) except the positive control and cobalt-chromium. The lowest inhibition was observed in stainless steel and titanium-molybdenum, lower than nickel-titanium, cobalt-chromium, and the positive control ( P <0.05).
Conclusions
All orthodontic archwires generate oxidative stress in vitro. Stainless steel archwires have the highest and nickel-titanium the lowest biocompatibility.
Orthodontic appliances and their corrosion products are in a prolonged, 1- to 2-year-long, contact with the oral mucosa during orthodontic treatment; this sets a high requirement for biocompatibility and stability. The role of orthodontic archwire alloys as mediators in the production of free radicals and oxidative stress has not been widely evaluated. Some heavy metals used in the fabrication of archwires, such as iron, chromium, copper, and cobalt undergo redox cycling, therefore directly producing free radicals, whereas nickel produces free radicals indirectly. Reactive oxygen species are forms of free radicals omnipresent in living cells as a result of either cell metabolism or the action of exogenous physical or chemical sources. DNA damage induced by reactive oxygen species is counteracted by cellular DNA repair mechanisms. Once these defense capacities are surpassed by an increased production of reactive oxygen species or their decreased elimination, oxidative stress linked to the mechanism of carcinogenesis develops. The detection of free radicals is complicated because of their short half-life and is therefore directed toward the products of oxidative stress. These are modified nucleosides, among which the most interesting is 8-hydroxy-2′-deoxyguanosine (8-OHdG), which is biologically highly relevant because it can induce the transversion of guanine into thymine, and this mutation is among the most frequently found in cancers. Previous studies of the effects of chronic exposure to various environmental factors on the concentration of 8-OHdG in leukocytes and urine have given mixed results. Studies financed by the industry often deny damage, whereas independent studies largely confirm DNA damage. Chronic exposure to nanoparticles, nicotine, metals, asbestos, azo dyes, benzene, polycyclic aromatic hydrocarbons, silicates, styrene, toluene, xylene, and rubber manufacturing has been studied so far. The available data suggest that dose-response relationships between chronic exposure and the induction of 8-OHdG have not yet been established.
The purpose of this study was to investigate the differences in the degree of in-vitro generated oxidative stress among different types of archwires.
Material and methods
Six types of orthodontic archwires were tested: (1) stainless steel (steel archwires; Forestadent, Pforzheim, Germany), (2) nickel-titanium (Titanol; Forestadent), (3) copper-nickel-titanium (Copper Ni-Ti; Ormco, Orange, Calif), (4) rhodium-coated nickel-titanium (BioForce High Aesthetic; Dentsply GAC, Bohemia, NY), (5) cobalt-chromium Blue Elgiloy (Coballoy; Dentsply GAC), and (6) titanium-molybdenum (TMA; Ormco) ( Table I ). To simulate conditions in the oral cavity, commercial artificial saliva (Glandosane; Stada Arzneimittel, Vienna, Austria) was used, and the pH of 6.75 ± 0.15 was adjusted with 10 mol/L of sodium hydroxide buffer. The samples contained 1 archwire of each type, and all tests were set up in triplicate and repeated twice. Each archwire was cut in pieces 1 cm long, weighed, autoclaved (at 121°C and 1 bar for 35 minutes, and placed in a sealed bag), immersed in artificial saliva of adjusted pH in sterile hermetic polyethylene tubes containing 1 mL of saliva per 0.2 g of archwire, and stored at 37°C under stationary conditions for 30 days. The artificial saliva with sodium hydroxide buffer, used as the negative control, was stored under the same conditions. After 30 days, the archwire pieces were removed, and the saliva samples were stored at 4°C until further analysis.
Iron | Nickel | Titanium | Chromium | Cobalt | Molybdenum | Copper | Manganese | Tin | Silicon | Other | |
---|---|---|---|---|---|---|---|---|---|---|---|
Stainless steel | 68.5-73.5 | 6-9 | 16-18 | <0.8 | <2 | <1.5 | Carbon, <0.12 | ||||
Nickel-titanium (Titanol) | <0.5 | 50-60 | 39.2-49.2 | Carbon, <0.1 Aluminum, <0.1 Oxygen, <0.1 |
|||||||
Copper-nickel-titanium (Copper Ni-Ti) | 49.1 | 45.6 | 0.2 | 5 | |||||||
Coated nickel-titanium (BioForce High Aesthetic) | 50-55 | 45-50 | Rhodium coating | ||||||||
Cobalt-chromium (Coballoy) | 15.3 | 15.4 | 19.9 | 39.8 | 7.1 | 1.97 | 0.48 | Carbon, <0.1 Phosphorus, <0.1 Sulfur, <0.1 Beryllium, <0.1 |
|||
Titanium-molydenum (TMA) | 80-85 | 10-13 | 3.8-5.3 |
The study was performed on cell cultures of mouse fibroblasts L929. All cells were grown in a culture in Dulbecco’s modified eagle medium (Sigma Aldrich, St Louis, Mo), supplemented with 10% fetal bovine serum (Gibco BRL, Grand Island, NY), 11 mmol/L sodium bicarbonate, 2 mmol/L L-glutamine, 100 μg/mL streptomycin, and 100 U/mL penicillin. Cells were cultivated in 100-mm cell culture dishes (Sigma Aldrich) in an amount of 2 × 10 6 cells in a humidified atmosphere containing 5% carbon dioxide at 37°C for 24 hours to obtain a cell monolayer.
We added 200 μL of each saliva sample to previously prepared samples of mouse fibroblast cultures in cell culture dishes (each, 2 × 10 6 cells). For the positive control, 200 μL of 0.1 mmol/L of hydrogen peroxide was added to the fibroblasts. The cell culture medium without the test material was used as the absolute control. The samples were incubated for 48 hours.
To determine the number of cells and their viability, 20 μL of cell suspension was mixed with 80 μL of 10% trypan blue solution (Sigma Aldrich), which specifically dyes dead cells blue. The cells were counted by using a hemocytometer (Neubauer chamber; Brand GMBH, Wertheim, Germany) on an optical microscope (Olympus BM-2, Olympus, Center Valley, Pa).
A commercial kit (GenElute Mammalian Genomic DNA kit; Sigma Aldrich) was used to isolate genomic DNA from the cells. Since the number of cells had increased from 2 to more than 5 × 10 6 within 2 days, 5 × 10 6 of cells were taken from each sample. The cells were then trypsinized and centrifuged at 300 times gravity for 5 minutes. The cells were resuspended in 200 μL of resuspension solution (PBS) in which 20 μL of RNase A solution had been added for elimination of RNA, and then incubated at room temperature for 2 minutes. According to the manufacturer’s instructions, the cells were resuspended and lysed. The addition of 95% ethanol (Kemika, Zagreb, Croatia) enabled the binding of DNA to the silicate membrane of the column. The remaining contamination was rinsed, and the DNA was eluted with a tris-EDTA buffer.
The quality and the amount of the extracted DNA were determined at wave lengths of 260 and 280 nm by using the spectrophotometer (NanoVue, GE Healthcare Bio-Sciences AB, Uppsala, Sweden).
The extracted DNA at 0.1 mg per milliliter was used for further analysis. The sample was converted into single-stranded DNA by incubation at 95°C for 5 minutes and rapid chilling on ice. The DNA was then digested into nucleosides by incubation with 10 U of nuclease P1 (Sigma Aldrich) for 2 hours at 37°C in 20 mmol/L of sodium hydroxie at a pH of 5.2, followed by treatment of 5 U of alkaline phosphatase (Sigma Aldrich) for 1 hour at 37°C in 100 mmol/L of tris buffer at a pH of 7.5. The reaction mixture was centrifuged at 6000 times gravity for 5 minutes, and the supernatant was used to assay the concentration of the oxidative stress marker 8-OHdG.
The oxidative DNA damage was determined with a competitive enzyme immunoassay (OxiSelect Oxidative DNA Damage ELISA Kit [8-OHdG quantitation]; Cell Biolabs, San Diego, Calif) following the manufacturer’s instructions. The absorbance readings were done immediately after that with a microplate reader (Start Fax 2010; Awareness Technology, Palm City, Fla) with a 620-nm filter. The quantity of 8-OHdG in each sample was determined by the comparison of its absorbance with the standard curve that had previously been made with series of dilutions of standards in the concentration span of 0 to 20 ng per milliliter.
Statistical analysis
Analysis of variance (ANOVA) with the Student-Newman-Keuls post-hoc test was used in statistical analysis, and the effect size was assessed by η 2 in statistical software (version 10.0; SPSS, Chicago, Ill).
Results
All samples showed high viability, and no acute toxicity was shown. The viability of the standard nickel-titanium archwire (94.13 ± 1.23) was closest to the positive control (89.93 ± 3.07) and was significantly lower than the negative and absolute controls and all wires ( P <0.05) except the titanium-molybdenum ( Table II ; Fig 1 ). Stainless steel showed the highest viability (98.97 ± 1.09), higher than nickel-titanium and the positive control ( P <0.05). The effect size was 75%, which implies that a great proportion of variability in viability was explained by differences in the tested groups.
Group | Viability index (%) ∗ | Cell growth (N-fold increase) † | OHdG (ng/mL) ‡ |
---|---|---|---|
Stainless steel | 98.97 ± 1.09 a | 7.25 ± 1.14 d | 1.25 ± 0.56 gh |
Titanium-molybdenum | 96.02 ± 2.35 ab | 7.58 ± 0.56 d | 0.74 ± 0.20 g |
Cobalt-chromium | 96.64 ± 1.42 a | 4.76 ± 1.07 ef | 1.53 ± 0.25 h |
Nickel-titanium | 94.13 ± 1.23 b | 3.75 ± 1.14 f | 2.39 ± 0.82 i |
Copper-nickel-titanium | 98.55 ± 1.48 a | 5.68 ± 0.83 de | 1.73 ± 0.21 h |
Coated nickel-titanium | 97.55 ± 1.41 a | 5.82 ± 0.84 de | 1.63 ± 0.08 h |
Control positive | 89.93 ± 3.07 c | 4.57 ± 0.10 ef | 1.10 ± 0.50 gh |
Control negative | 97.75 ± 1.94 a | 6.17 ± 2.90 de | 0.65 ± 0.22 g |
Control absolute | 97.65 ± 0.63 a | 6.28 ± 1.06 de | 0.87 ± 0.26 g |
∗ ANOVA, P <0.001; η 2 = 0.750.
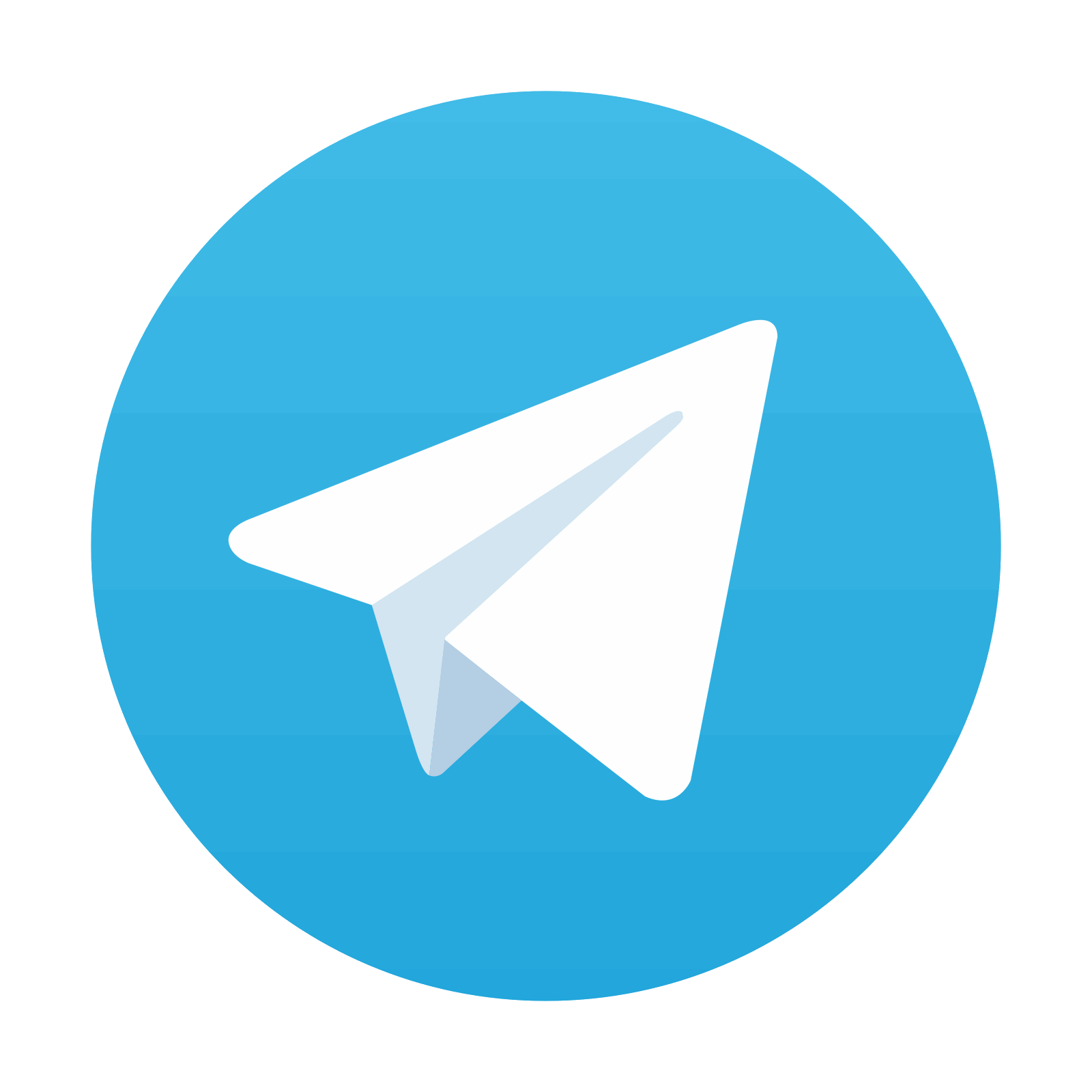
Stay updated, free dental videos. Join our Telegram channel

VIDEdental - Online dental courses
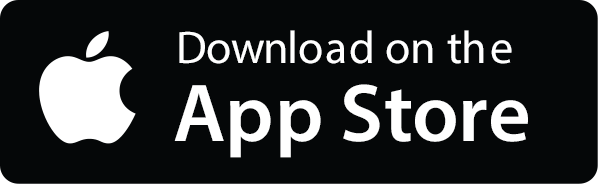
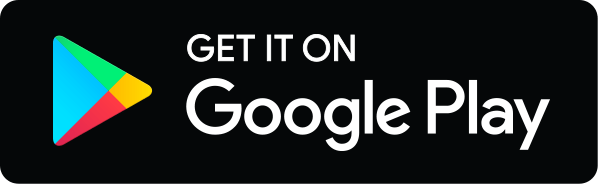