Highlights
- •
Cryo-FIB specimen preparation for TEM imaging of resin–dentin interfaces is proposed.
- •
We report step by step FIB sample preparations in details.
- •
Minimizing the milling temperature improve collagen fibrils visibility.
Abstract
Objective
The introduction of focused ion beam (FIB) milling has facilitated preparation of hard tissue samples for transmission electron microscope (TEM). However, this technique generates high temperature that may alter or damage morphological features in biological tissue. Therefore, the aim of this study was to determine the effects of cryogenic cooling on the morphological features of dentin interfaces with dental restorative materials in samples prepared by FIB for TEM examination.
Methods
After preparation of a cylindrical-shaped cavities in extracted, non-carious premolar teeth, the specimens were restored with dental adhesive/composite and categorized into two restorative materials groups; (PB) a combination of Clearfil Protect Bond (Kuraray Noritake Dental, Japan)/Estelite Sigma Quick composite (Tokuyama Dental, Japan), and (SB) Filtek Silorane restorative system (3M ESPE, USA). The specimens were subjected to interfacial cross-sectioning, followed by observation and area selection using confocal laser microscopy. Later, ultrathin sections were prepared using FIB with cryogenic cooling (PB-C) and (SB-C), or without cooling (PB-NC) and (SB-NC) that all were examined under TEM.
Results
Resulting TEM images of the ultra-morphological features at the resin–dentin nano-interaction zone were improved when FIB preparation was conducted in the cryogenic condition and no sign of artifacts were detected.
Significance
Conducting ion beam milling with cryogenic cooling was advantageous in minimizing the elevation in specimen temperature. This led to preservation of dentin microstructures that revealed additional information about substrates that are necessary for advanced characterization of tooth–biomaterial interactions.
1
Introduction
The introduction of nanometer-scale technology has attracted great attention and has advanced the science of nanostructural observation. In the biomedical field, nano-characterization has enhanced the development, synthesis and optimization of biomaterials, and helped in understating of the mechanisms of tissue–biomaterial interactions.
Dentin is the most abundant hard tissue in human teeth and has been extensively studied with different techniques and under several conditions in the past several decades . The tissue is composed of 50 vol.% mineral, 30 vol.% organic matrix and 20 vol.% fluid . This tissue is anisotropic in nature and contains dentinal tubules, which are cylindrical in shape ranging 1–2 μm in diameter, extending from dentinoenamel junction and cemintoenamel junction toward the pulp chamber. Each dentinal tubule is surrounded by a thin, highly mineralized cuff of peritubular dentin . In addition, the complexity of dentin microstructure is attributed to its mineralized collagen fibrils; mainly type-I collagen, ranging from 50 to 100 nm in diameter, forming the structural matrix of dentin. The fibrils are oriented orthogonal to the dentinal tubules to create the intertubular dentin matrix .
Achieving satisfactory biomechanical adhesion between resin polymers and dentin structure is still one of the major demands in restorative dentistry . Resin–dentin bonding has evolved in an effort to obtain an intimate interfacial adhesion between monomer-impregnated dentin matrices containing both mineral-apatite (Ap) crystallites and demineralized collagen fibrils. The interfacial interactions between dental adhesives and dentin structure have been researched using various microscopic techniques . Transmission electron microscopy (TEM) is a well-established and valuable methodology in evaluation of dental interfaces . TEM requires preparation of very fine sections of the tissue that are sufficiently thin (70–90 nm) to allow the incident electron beam to pass through. Ultramicrotomy with a thin diamond blade has been conventionally used for preparation of the thin sections for TEM . Nevertheless, this sectioning technique has several limitations, which include the non-uniform slice thickness and the inability to choose specific sites of interest . Moreover, the method is extremely difficult when hard or brittle materials such as dental enamel, ceramics and dental composites are involved. The mechanical stress induced during sample preparation can possibly lead to the loss of integrated chemical and morphological structures . Cryogenic ultramicrotomy was introduced to limit the need for sample fixation .
Recently, focused ion beam (FIB) milling has been introduced as an alternative method to conventional specimen preparation using microtome, which overcame many technical problems associated with conventional ultramicrotomy . FIB works by ablation of a small amount of material when a primary ion beam hits the sample surface. Removal of material by ablation allows precision milling of the specimen down to a nano-scale. This site-specific milling tool enables unique capabilities ranging from top-down structuring in nanotechnology to tomographic characterization, which can be conducted on a wide array of materials including semiconductors, metals, ceramics, polymers and biological tissues . This technology can be implemented in conjugation with scanning electron microscopy (SEM) and TEM to evaluate tooth-resin interfaces at the nano-scale. However, FIB may cause local heat production along the beam path that could alter or damage the ultramorphological features of the biological tissue at room temperature . It was supposed that the beam damage would be minimized if the thinning was conducted under cryogenic conditions . While dental research is gradually adopting this milling technology as a new tool in characterization of the tissue, the effect of the beam on dentin structure has only been researched to a limited extent. Therefore, the purpose of this study was to assess the ultramorphological structures at the nano-interaction zone of the restored dentin cavities obtained by Cryo-FIB/TEM technique in comparison to conventional FIB/TEM.
2
Materials and methods
2.1
Materials used
The materials used in this study are listed in ( Table 1 ); the lot numbers and chemical composition of each material are according to the information provided by the manufacturers. Two self-etch adhesive systems and two resin composites were used in this study; the two-step self-etch adhesive Clearfil Protect Bond (PB; Kuraray Noritake Dental, Tokyo, Japan), was used with a light-cured universal composite resin with sub-micron spherical fillers Estelite Sigma Quick (shade A2, Tokuyama Dental, Tokyo, Japan), and the Silorane system adhesive (SB; (3M ESPE, St. Paul, MN, USA) was used with Filtek Silorane composite system (Shade A2, 3 M ESPE, St. Paul, MN, USA).
Material (manufacturer) CODE | Composition | Lot number | Fillers % |
---|---|---|---|
Clearfil Protect Bond Adhesive Two-Step Self-Etch (Kuraray Noritake Dental, Japan) | Primer: MDPB, MDP, HEMA, hydrophilic aliphatic methacrylate, water, CQ, dyes, initiators, accelerators, others (pH 2.0) | 00082B | – |
PB | Bond: MDP, Bis-GMA, HEMA, hydrophobic aliphatic methacrylate, sodium fluoride, colloidal silica, CQ, initiators, accelerators, others | 00137A | – |
Estelite sigma Quick Universal Composite (Tokuyama Dental, Japan) | Composite: Bis-GMA, TEGDMA, silica–zirconia fillers, silica–titania fillers, photoinitiators | J018 | 82% (wt.) 71% (vol) |
Silorane System Adhesive Two-Step Self-Etch (3M ESPE, USA) SB | Primer: phosphorylated methacrylates, vitrebond copolymer, Bis-GMA, HEMA, water, ethanol, silane-treated silica filler, initiators, stabilizers (pH 2.7) | N289224 | – |
Bond: hydrophobic dimethacrylate, phosphorylated methacrylates, TEGDMA, silane-treated silica filler, Initiators, stabilizers | N209848 | – | |
Filter Silorane Low Shrinkage Posterior Composite (3 M ESPE, USA) | Composite: silorane resin, CQ, iodonium salt, electron donor, quartz filler, yttrium fluoride, stabilizers, pigments | N204592 | 76% (wt.) 55% (vol) |
2.2
Restorative technique
The experiment design adopted in this study, in particular for use on caries-free, extracted human premolars complies with the guiding principles for experimental procedures found in the Declaration of Helsinki of the World Medical Association, and has been duly cleared by the Institutional Review Board and institutional Ethics Committee of King Abdulaziz University and Tokyo Medical and Dental University.
A schematic illustration of the restorative technique is shown in Fig. 1 a . After random specimen selection and soft tissue removal, the roots were removed by grinding and the occlusal cusps were flattened. Standard cylindrical-shaped class-I cavities were prepared on the occlusal surface (3 mm in diameter and 1.5 mm in depth) using a flat-end long tapered diamond bur (ISO #021, Shofu, Kyoto, Japan) and finished with superfine flat-end diamond bur (ISO #013, Shofu) mounted on an air turbine headpiece (Ti-Max X, NSK, Kanuma, Japan) and under copious cooling water to minimize the micro-gap formation at the interface and to improve the marginal integrity of the resin restoration . Afterward, the specimens were categorized into two restorative materials groups; (PB) where a combination of Clearfil Protect Bond/Estelite Sigma Quick composite was used, or (SB) in which the teeth were restored with Filtek Silorane adhesive/composite system. Each group were restored in bulk filling technique and light-cured in accordance with the manufacturer instructions using a halogen curing unit (Optilux 501, Kerr, CA, USA; 500 mW/cm 2 intensity) that was tested for consistent output before each use to avoid halogen aging that could interfere with adequate polymerization . All specimens were stored in 25 °C water for 24 h.
2.3
Cross-sectional observation using confocal laser scanning microscope (CLSM)
After the specimens were stored for 24 h to permit complete resin polymerization, they were trimmed from the buccal surface, using silicon carbide papers ranging from 280-grit up to 2000-grit in an ascending sequence under running water, and then further polished with diamond pastes with particle sizes of 6, 3, 1 and finally 0.25 μm in circular motion under copious cooling water. Then, direct observation of the flattened tooth at the tooth-restoration interface in each sample was done using CLSM (1LM21H/W, Lasertec, Yokohama, Japan) ( Fig. 1 a–c). The interfaces were imaged at (1250×) and (2500×) magnifications in order to ensure good initial adaptation at the base of the cavity before selecting the target area.
2.4
Focused ion beam (FIB)
Generally, when FIB is used as an imaging tool, known as scanning ion microscopy (SIM), it operates in a similar manner to a SEM, with the major difference being that ions (rather than electrons), usually gallium (Ga), interact with the surface to produce a secondary electron signal. Moreover, since the ions have a much larger mass than the electrons, the ion beam has the capability of ablating away atoms interacting with the beam, allowing the FIB to be used as a milling tool if high ion currents are selected. Moreover, FIB has the ability to use gas chemistries to induce ion beam-assisted chemical vapor deposition (FIB-AD). This system utilizes tungsten, platinum and amorphous carbon deposition as a protective layer for the sample surface when milling near sensitive areas, and where ion beam overspray could affect the sample.
In this study, specimen preparations were conducted using the FIB system (FB2200, Hitachi, Tokyo, Japan) followed by TEM observations (HD-2700, Hitachi, Tokyo, Japan). This site-specific milling tool equipped with microsampling system, consisted of a vacuum chamber, a liquid metal ion source (LMIS), an ion column, a sample stage, detectors, probes, gas delivery system, and a computer to control the device.
2.5
Cryogenic preparation
The FIB system is capable of operating under cryogenic conditions. In this case, liquid nitrogen gas (LN) is used for cooling of the sample stage (−100 °C) that assisted in stabilizing of the hydrated specimens by keeping them at cryogenic conditions during FIB milling and metal deposition process.
2.6
FIB specimen preparation
Before FIB application, cross-sectioned restorative material groups (PB, SB) were divided into four subgroups according to the FIB specimens preparation conditions; (PB-NC, SB-NC) as control groups that were prepared at normal chamber temperature (25 °C), and test groups (PB-C, SB-C) that were prepared in cryogenic condition (about −100 °C). The preparatory steps for FIB/TEM are described in Fig. 2 . All the steps were accomplished under vacuum in the FIB system and imaged under SIM to assist the operator in visualization of sample after each step.
In control groups (PB-NC, SB-NC) and after selection of the target area (5 μm length × 4 μm width), a protective layer of tungsten was deposited on the exposed target surface using FIB-AD technique. Then the target area was FIB ablated (40 kV) into a prism-shaped configuration (or micro-sample) (5 μm length × 4 μm width × 5 μm depth), leaving a solid-bridge on one side to support the micro-sample. Thereafter, the micro-sample and a built-in metal needle (micro-manipulator) were fixed together by means of tungsten deposition using FIB-AD. Further FIB milling was completed all around the tungsten area until the micro-sample could be removed from the specimen. Each extracted micro-sample was transferred and fixed on a compatible FIB/TEM stage using FIB-AD. Then, a final round of tungsten deposition over the extracted micro-sample was carried out to protect the surface from FIB excessive charging during the thinning procedure. Later, the micro-sample was ultrathinned into 100 nm thickness (down to 5 kV beam) using H-bar/microsampling technique followed by TEM observation ( Fig. 3 ).
In contrast, the test groups (PB-C, SB-C) were positioned and secured on the FIB cryo-stage, and then subjected to similar preparation, metal deposition, thinning parameters and microsampling procedure.
2.7
Transmission electron microscopy examination
After FIB milling, the sections were transferred using molybdenum mesh to a scanning transmission electron microscope (STEM) HD-2700 for ultramorphological examination. STEM was conducted at 80 kV and the imaging was carried out under several magnifications (30,000× to 300,000×). In order to find differences among the groups, a visual qualitative analysis was performed by comparing the contrast of the resin–dentin ultrastructure, structural details and signs of artifacts or damage.
2
Materials and methods
2.1
Materials used
The materials used in this study are listed in ( Table 1 ); the lot numbers and chemical composition of each material are according to the information provided by the manufacturers. Two self-etch adhesive systems and two resin composites were used in this study; the two-step self-etch adhesive Clearfil Protect Bond (PB; Kuraray Noritake Dental, Tokyo, Japan), was used with a light-cured universal composite resin with sub-micron spherical fillers Estelite Sigma Quick (shade A2, Tokuyama Dental, Tokyo, Japan), and the Silorane system adhesive (SB; (3M ESPE, St. Paul, MN, USA) was used with Filtek Silorane composite system (Shade A2, 3 M ESPE, St. Paul, MN, USA).
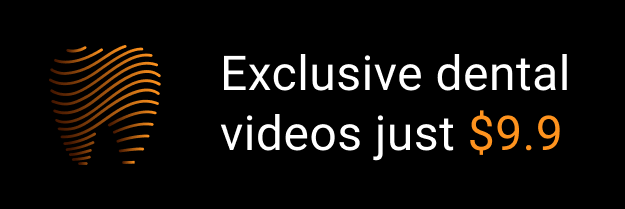