Highlights
- •
The new adhesive containing 7.5% MPC + 5% DMAHDM had protein adsorption that was nearly 20-fold less than that of commercial SBMP control.
- •
Adding MPC or DMAHDM each alone greatly decreased the biofilm viability, CFU and lactic acid production, compared to SBMP control.
- •
Adding MPC and DMAHDM together in adhesive achieved antibacterial effects much stronger than each alone, reducing biofilm CFU by four orders of magnitude.
- •
Incorporation of 7.5% MPC and 5% DMAHDM into primer and adhesive to obtain protein-repellent and antibacterial activities did not compromise dentin bond strength.
- •
The use of double agents (protein-repellent MPC + antibacterial DMAHDM) in dental adhesive achieved much stronger inhibition of biofilms than using each agent alone.
- •
The novel protein-repellent and antibacterial bonding agent is promising to reduce biofilm/plaque buildup and reduce recurrent caries at the tooth-restoration margins.
Abstract
Objectives
Secondary caries at the tooth-restoration margins remains a main reason for restoration failure. The objectives of this study were to: (1) combine protein-repellent 2-methacryloyloxyethyl phosphorylcholine (MPC) with quaternary ammonium dimethylaminohexadecyl methacrylate (DMAHDM) to develop a new dental adhesive with double benefits of protein-repellent and antibacterial capabilities for the first time; and (2) investigate the effects on protein adsorption, anti-biofilm activity, and dentin bond strength.
Methods
MPC and DMAHDM were incorporated into Scotchbond Multi-Purpose (SBMP) primer and adhesive. Dentin shear bond strengths were measured using extracted human molars. Protein adsorption onto the adhesive resin surfaces was determined by the micro bicinchoninic acid (BCA) method. A dental plaque microcosm biofilm model with human saliva as inoculum was used to investigate biofilm metabolic activity, colony-forming unit (CFU) counts, lactic acid production and live/dead staining of biofilms on resins.
Results
Incorporation of 7.5% MPC and 5% DMAHDM into primer and adhesive did not adversely affect the dentin shear bond strength ( p > 0.1). The resin with 7.5% MPC + 5% DMAHDM had protein adsorption that was nearly 20-fold less than SBMP control ( p < 0.05). The resin with 7.5% MPC + 5% DMAHDM had much stronger antibacterial effects than using MPC or DMAHDM alone ( p < 0.05). Biofilm CFU counts on the resin with 7.5% MPC + 5% DMAHDM were reduced by more than 4 orders of magnitude, compared to SBMP control.
Significance
The use of double agents (protein-repellent MPC + antibacterial DMAHDM) in dental adhesive achieved much stronger inhibition of biofilms than using each agent alone. The novel protein-repellent and antibacterial bonding agent is promising to reduce biofilm/plaque buildup and reduce recurrent caries at the tooth-restoration margins.
1
Introduction
A primary reason for tooth cavity restoration failure is secondary caries at the tooth-restoration margins, caused by acid production by biofilms . There are usually residual bacteria in the prepared tooth cavity, and during service in vivo there are invading bacteria along the tooth-restoration margins due to bacterial leakage . Resin composites are popular filling materials due to their esthetics and direct-filling capabilities . Extensive studies have resulted in substantial improvements in composite fillers, polymer compositions, and handling and polymerization properties . However, previous studies showed that resin composites in vivo had more biofilm accumulation than other restorative materials . Composite restorations are bonded to the tooth structures via bonding agents . Adhesive compositions and bonding procedures have been improved, and the tooth-restoration bond strength has been enhanced . It is desirable to use an antibacterial bonding agent to combat biofilms at the tooth-restoration margins. Indeed, novel quaternary ammonium methacrylates (QAMs) were synthesized and incorporated into resins . Antibacterial adhesives containing QAMs substantially reduced biofilm growth and viability . For example, 12-methacryloyloxydodecylpyridinium bromide (MDPB) was incorporated into primer and adhesive with strong antibacterial properties . Methacryloxylethyl cetyl dimethyl ammonium chloride (DMAE-CB) was also used to develop antibacterial bonding agents . Recently, a quaternary ammonium dimethacrylate (QADM) was synthesized and incorporated into primer and adhesive . It was shown that the antibacterial potency of quaternary ammonium compounds increased with an increase in the alkyl chain length (CL) for the ammonium groups . More recently, a new quaternary ammonium monomer, dimethylaminohexadecyl methacrylate (DMAHDM, CL = 16) was synthesized and incorporated into dental adhesive, which showed a strong antibacterial activity .
A perfect seal at the margins is an important goal, but it is often difficult to achieve. Microgaps were often observed between the adhesive resin and the primed dentin, or between the adhesive resin and the hybrid layer . These microgaps could further deteriorate due to cyclic fatigue and wear actions . These microgaps could harbor biofilms, with acid production leading to secondary caries. Biofilm formation on tooth-restoration interfaces is a source of infection and is a prerequisite for secondary caries . A large portion of these marginal gaps would be surrounded by the cured adhesive resin, hence the invading bacteria would come into contact with the adhesive surface. Therefore, it is beneficial to have an adhesive that can inhibit biofilms.
Protein adsorption from physiological fluids such as saliva-derived protein film on a surface is an initial required step in bacteria attachment on the surface . Hence, it would be desirable to develop an adhesive resin that repels protein adsorption, thereby to repel bacteria attachment. A protein-repellent monomer, 2-methacryloyloxyethyl phosphorylcholine (MPC), is a methacrylate with a phospholipid polar group in the side chain . MPC is a common biocompatible and hydrophilic biomedical polymer . The excellent biocompatibility of MPC-containing biomaterials has been confirmed by their various characteristics such as being inert for biological systems, and with reduced levels of protein absorption, bacterial adhesion and cellular attachment . Several medical devices with MPC have been developed and used clinically . Recently, MPC was incorporated into dentin bonding agents, achieving strong protein-repellent ability . However, to date there has been no report on adhesives that possess both protein-repellent and antibacterial capabilities.
Accordingly, the objectives of this study were to incorporate MPC and DMAHDM to develop a dental adhesive with a combination of protein-repellent and antibacterial capabilities for the first time. The following hypotheses were tested: (1) Incorporating MPC and DMAHDM into primer and adhesive would not compromise the dentin bond strength; (2) primer and adhesive containing MPC and DMAHDM would have much less protein adsorption than a commercial bonding agent control; and (3) the dual agents of MPC and DMAHDM would yield protein-repellent and antibacterial primer and adhesive with much greater anti-biofilm potency than using MPC or DMAHDM alone.
2
Materials and methods
2.1
Incorporation of MPC into bonding agent
Scotchbond Multi-Purpose Adhesive and Primer (referred as “SBMP”) (3 M, St. Paul, MN) were used as the parent bonding system. According to the manufacturer, SBMP adhesive contained 60–70% of bisphenol A diglycidyl methacrylate (BisGMA) and 30–40% of 2-hydroxyethyl methacrylate (HEMA), tertiary amines and photo-initiator. SBMP primer contained 35–45% of HEMA, 10–20% of a copolymer of acrylic and itaconic acids, and 40–50% water. The protein-repellent monomer, 2-methacryloyloxyethyl phosphorylcholine (MPC), was synthesized via a method reported by Ishihara et al. and commercially available (Sigma-Aldrich, St. Louis, MO). The MPC powder was mixed with SBMP primer at MPC/(SBMP primer + MPC) of 7.5% by mass. The 7.5% mass fraction was selected following a previous study , which showed that 7.5% MPC in adhesive resin yielded the strongest protein-repellent property without compromising the dentin shear bond strength. The primer with MPC was magnetically-stirred with a bar at a speed of 150 rpm (Bellco Glass, Vineland, NJ) for 24 h to completely dissolve MPC in the SBMP primer. Similarly, 7.5% MPC by mass was also mixed into the SBMP adhesive.
2.2
Incorporation of DMAHDM into bonding agent
Dimethylaminohexadecyl methacrylate (DMAHDM) with an alkyl chain length of 16 was synthesized using a modified Menschutkin reaction following previous studies . In this method, a tertiary amine group was reacted with an organo-halide. A benefit of this reaction is that the reaction products are generated at virtually quantitative amounts and require minimal purification. Briefly, 10 mmol of 2-(dimethylamino) ethyl methacrylate (DMAEMA, Sigma-Aldrich, St. Louis MO) and 10 mmol of 1-bromohexadecane (BHD, TCI America, Port-land, OR) were combined with 3 g of ethanol in a 20 mL scintillation vial. The vial was stirred at 70 °C for 24 h. The solvent was then removed via evaporation, yielding DMAHDM as a clear, colorless, and viscous liquid .
SBMP primer was first mixed with MPC as described above. Then, the DMAHDM was mixed into the SBMP-MPC primer, at DMAHDM/(primer + DMAHDM) mass fractions of 5%, 7.5%, and 10%, to determine the optimal DMAHDM mass fraction when combined with 7.5% MPC. These DMAHDM mass fractions followed previous studies which had no MPC . Similarly, DMAHDM was incorporated into the SBMP-MPC adhesive at the same mass fractions. The following eight bonding systems were investigated:
- (1)
SBMP primer and adhesive control (termed “SBMP control”);
- (2)
SBMP primer + 7.5% MPC, SBMP adhesive + 7.5% MPC (“7.5% MPC”);
- (3)
SBMP primer + 5% DMAHDM, SBMP adhesive + 5% DMAHDM (“5% DMAHDM”);
- (4)
SBMP primer + 7.5% DMAHDM, SBMP adhesive + 7.5% DMAHDM (“7.5% DMAHDM”);
- (5)
SBMP primer + 10% DMAHDM, SBMP adhesive + 10% DMAHDM (“10% DMAHDM”);
- (6)
SBMP primer + 7.5% MPC + 5% DMAHDM, SBMP adhesive + 7.5% MPC + 5% DMAHDM (“7.5% MPC + 5% DMAHDM”);
- (7)
SBMP primer + 7.5% MPC + 7.5% DMAHDM, SBMP adhesive + 7.5% MPC + 7.5% DMAHDM (“7.5% MPC + 7.5% DMAHDM”);
- (8)
SBMP primer + 7.5% MPC + 10% DMAHDM, SBMP adhesive + 7.5% MPC + 10% DMAHDM (“7.5% MPC + 10% DMAHDM”).
2.3
Dentin shear bond testing
The use of extracted human teeth was approved by the University of Maryland. Extracted caries-free molars were first sawed to remove the crowns (Isomet, Buehler, Lake Bluff, IL), then ground perpendicularly to their longitudinal axes on 320 grit SiC paper until occlusal enamel was completely removed . The dentin surface was etched with etchant for 15 s and rinsed with water . A primer was applied, and the solvent was removed with an air stream. The adhesive was applied and light-cured for 10 s (Optilux-VCL401, Demetron, Danbury, CT). A stainless-steel iris, having a central opening with a diameter of 4 mm and a thickness of 1.5 mm, was held against the adhesive-treated dentin surface. The central opening was filled with a composite (TPH, Caulk/Dentsply, Milford, DE), and light-cured for 60 s . The bonded specimens were stored in distilled water at 37 °C for 24 h . The dentin shear bond strength, S D , was measured as previously described . A chisel was held parallel to the composite-dentin interface and loaded via a Universal Testing Machine (MTS, Eden Prairie, MN) at 0.5 mm/min until the composite-dentin bond failed. S D = 4 P /( πd 2 ), where P is the load at failure, and d is the diameter of the composite . Ten teeth were tested for each group, requiring a total of 80 teeth.
2.4
Degree of vinyl conversion
The degree of conversion (DC) of SBMP adhesive control and SBMP containing MPC and DMAHDM at the concentrations described above were measured by near-infrared (NIR) spectroscopy (Nicolet 6700, Thermo Scientific, Waltham, MA) . Specimens were photo-cured in a 4 mm diameter glass sample holder for 60 s. NIR spectra (3 specimens/group) were acquired before photo-cure and 24 h post-cure. DC was calculated from the percentage of change in the integrated peak area of the 6165 cm −1 methacrylate CH 2 absorption band normalized to thickness of the specimen before and after cure, following a previous study .
2.5
Specimen fabrication for protein adsorption and biofilm experiments
The dentin shear bond strength results showed that the 7.5% MPC + 7.5% DMAHDM group and the 7.5% MPC + 10% DMAHDM group had significantly lower dentin bond strengths than SBMP control. Therefore, 7.5% DMAHDM and 10% DMAHDM mass fractions were not used in the subsequent protein adsorption and biofilm experiments. The following four groups were used for the protein and biofilm tests:
- (1)
SBMP primer and adhesive control (termed “SBMP control”);
- (2)
SBMP primer + 7.5% MPC, SBMP adhesive + 7.5% MPC (“7.5% MPC”);
- (3)
SBMP primer + 5% DMAHDM, SBMP adhesive + 5% DMAHDM (“5% DMAHDM”);
- (4)
SBMP primer + 7.5% MPC + 5% DMAHDM, SBMP adhesive + 7.5% MPC + 5% DMAHDM (“7.5% MPC + 5% DMAHDM”).
The cover of a sterile 96-well plate (Costar, Corning Inc., Corning, NY) was used for fabricating disk specimens following previous studies . Briefly, 10 μL of a primer was placed in the bottom of each dent. After drying with a stream of air, 20 μL of adhesive was applied to the dent and photo-polymerized for 30 s, using a mylar strip covering to obtain a disk of approximately 8 mm in diameter and 0.5 mm in thickness. The cured disks were immersed in 200 mL of distilled water and magnetically-stirred with a bar at a speed of 100 rpm for 1 h to remove any uncured monomers . The disks were then sterilized with ethylene oxide (Anprolene AN 74i, Andersen, Haw River, NC) and de-gassed for 3 days .
2.6
Measurement of protein adsorption by micro bicinchoninic acid method
The amount of protein adsorbed on resin disks was determined by the micro bicinchoninic acid (BCA) method . Each disk was immersed in phosphate buffered saline (PBS) for 2 h. The disks then were immersed in bovine serum albumin (BSA) (Sigma-Aldrich) solutions at 37 °C for 2 h. The protein solutions contained BSA at a concentration of 4.5 g/L following previous studies . The disks then were rinsed with fresh PBS by stirring at a speed of 300 rpm for 5 min (Bellco Glass, Vineland, NJ), immersed in sodium dodecyl sulfate (SDS) at 1 wt% in PBS, and sonicated at room temperature for 20 min to completely detach the BSA from disk surfaces. A protein analysis kit (micro BCA protein assay kit, Fisher Scientific, Pittsburgh, PA) was used to determine the BSA concentration in the SDS solution. From the concentration of protein, the amount of protein adsorbed on the resin disk was calculated . Six disks were evaluated for each group.
2.7
Saliva collection for biofilm inoculum
The biofilm viability was investigated using a dental plaque microcosm model following previous studies . Saliva was collected from ten healthy adult donors having natural dentition without active caries or periopathology, and without the use of antibiotics within the last 3 months, following previous studies . The donors did not brush teeth for 24 h and abstained from food and drink intake for 2 h prior to donating saliva. Stimulated saliva was collected during parafilm chewing and was kept on ice. An equal volume of saliva from each of the ten donors was combined to form the saliva sample. The saliva was diluted in sterile glycerol to a concentration of 70%, and stored at −80 °C .
2.8
Dental plaque microcosm biofilm formation and live/dead assay
The saliva-glycerol stock was added, with 1:50 final dilution, to a growth medium as inoculum. The growth medium contained mucin (type II, porcine, gastric) at a concentration of 2.5 g/L; bacteriological peptone, 2.0 g/L; tryptone, 2.0 g/L; yeast extract, 1.0 g/L; NaCl, 0.35 g/L, KCl, 0.2 g/L; CaCl 2 , 0.2 g/L; cysteine hydrochloride, 0.1 g/L; hemin, 0.001 g/L; vitamin K1, 0.0002 g/L, at pH 7 . 1.5 mL of inoculum was added to each well of 24-well plates containing a resin disk, and incubated at 37 °C in 5% CO 2 for 8 h. Then, the disks were transferred to new 24-well plates filled with fresh medium and incubated. After 16 h, the disks were transferred to new 24-well plates with fresh medium and incubated for 24 h. This totaled two days of culture, which formed microcosm biofilms as shown previously .
Disks with 2-day biofilms were washed with PBS and stained using the BacLight live/dead kit (Molecular Probes, Eugene, OR) . Live bacteria were stained with Syto 9 to produce a green fluorescence, and bacteria with compromised membranes were stained with propidium iodide to produce a red fluorescence. The stained disks were examined using an inverted epifluorescence microscope (Eclipse TE2000-S, Nikon, Melville, NY). Six specimens were evaluated for each group. Three randomly chosen fields of view were photographed for each disk, yielding a total of 18 images for each group.
2.9
Lactic acid production and colony-forming unit (CFU) counts
Resin disks with 2-day biofilms were rinsed with cysteine peptone water (CPW) to remove loose bacteria . The disks were transferred to 24-well plates containing buffered peptone water (BPW) plus 0.2% sucrose. The disks were incubated in 5% CO 2 at 37 °C for 3 h to allow the biofilms to produce acid . The BPW solutions were then stored for lactate analysis. Lactate concentrations in the BPW solutions were determined using an enzymatic (lactate dehydrogenase) method, following a previous study . A microplate reader (SpectraMax M5, Molecular Devices, Sunnyvale, CA) was used to measure the absorbance at 340 nm (optical density OD340) for the collected BPW solutions. Standard curves were prepared using a lactic acid standard (Supelco, Bellefonte, PA) .
Disks with 2-day biofilms were transferred into tubes with 2 mL CPW, and the biofilms were harvested by sonication and vortexing (Fisher, Pittsburgh, PA) . Three types of agar plates were used to measure the CFU counts to assess the microorganism viability. First, tryptic soy blood agar culture plates were used to determine total microorganisms . Second, mitis salivarius agar (MSA) culture plates containing 15% sucrose were used to determine total streptococci . Third, MSA agar culture plates plus 0.2 units of bacitracin per mL was used to determine mutans streptococci . The bacterial suspensions were serially diluted, spread onto agar plates and incubated at 37 °C in 5% CO 2 for 24 h . The number of colonies that grew were counted and used, along with the dilution factor, to calculate the CFU on each resin disk .
2.10
MTT assay of metabolic activity of biofilms
A MTT (3-[4,5-dimethylthiazol-2-yl]-2,5-diphenyltetrazolium bromide) assay was used to examine the metabolic activity of biofilms . MTT is a colorimetric assay that measures the enzymatic reduction of MTT, a yellow tetrazole, to formazan. Disks with 2-day biofilms were transferred to a new 24-well plate, then 1 mL of MTT dye (0.5 mg/mL MTT in PBS) was added to each well and incubated at 37 °C in 5% CO 2 for 1 h. During this process, metabolically active bacteria reduced the MTT to purple formazan. After 1 h, the disks were transferred to a new 24-well plate, 1 mL of dimethyl sulfoxide (DMSO) was added to solubilize the formazan crystals, and the plate was incubated for 20 min at room temperature in the dark. After mixing via pipetting, 200 mL of the DMSO solution from each well was transferred to a 96-well plate, and the absorbance at 540 nm was measured via the microplate reader (SpectraMax M5) . A higher absorbance is related to a higher formazan concentration, which indicates a higher metabolic activity in the biofilm on the disk .
2.11
Statistical analysis
One-way and two-way analyses of variance (ANOVA) were performed to detect the significant effects of the variables. Tukey’s multiple comparison test was used to compare the data at a p value of 0.05.
2
Materials and methods
2.1
Incorporation of MPC into bonding agent
Scotchbond Multi-Purpose Adhesive and Primer (referred as “SBMP”) (3 M, St. Paul, MN) were used as the parent bonding system. According to the manufacturer, SBMP adhesive contained 60–70% of bisphenol A diglycidyl methacrylate (BisGMA) and 30–40% of 2-hydroxyethyl methacrylate (HEMA), tertiary amines and photo-initiator. SBMP primer contained 35–45% of HEMA, 10–20% of a copolymer of acrylic and itaconic acids, and 40–50% water. The protein-repellent monomer, 2-methacryloyloxyethyl phosphorylcholine (MPC), was synthesized via a method reported by Ishihara et al. and commercially available (Sigma-Aldrich, St. Louis, MO). The MPC powder was mixed with SBMP primer at MPC/(SBMP primer + MPC) of 7.5% by mass. The 7.5% mass fraction was selected following a previous study , which showed that 7.5% MPC in adhesive resin yielded the strongest protein-repellent property without compromising the dentin shear bond strength. The primer with MPC was magnetically-stirred with a bar at a speed of 150 rpm (Bellco Glass, Vineland, NJ) for 24 h to completely dissolve MPC in the SBMP primer. Similarly, 7.5% MPC by mass was also mixed into the SBMP adhesive.
2.2
Incorporation of DMAHDM into bonding agent
Dimethylaminohexadecyl methacrylate (DMAHDM) with an alkyl chain length of 16 was synthesized using a modified Menschutkin reaction following previous studies . In this method, a tertiary amine group was reacted with an organo-halide. A benefit of this reaction is that the reaction products are generated at virtually quantitative amounts and require minimal purification. Briefly, 10 mmol of 2-(dimethylamino) ethyl methacrylate (DMAEMA, Sigma-Aldrich, St. Louis MO) and 10 mmol of 1-bromohexadecane (BHD, TCI America, Port-land, OR) were combined with 3 g of ethanol in a 20 mL scintillation vial. The vial was stirred at 70 °C for 24 h. The solvent was then removed via evaporation, yielding DMAHDM as a clear, colorless, and viscous liquid .
SBMP primer was first mixed with MPC as described above. Then, the DMAHDM was mixed into the SBMP-MPC primer, at DMAHDM/(primer + DMAHDM) mass fractions of 5%, 7.5%, and 10%, to determine the optimal DMAHDM mass fraction when combined with 7.5% MPC. These DMAHDM mass fractions followed previous studies which had no MPC . Similarly, DMAHDM was incorporated into the SBMP-MPC adhesive at the same mass fractions. The following eight bonding systems were investigated:
- (1)
SBMP primer and adhesive control (termed “SBMP control”);
- (2)
SBMP primer + 7.5% MPC, SBMP adhesive + 7.5% MPC (“7.5% MPC”);
- (3)
SBMP primer + 5% DMAHDM, SBMP adhesive + 5% DMAHDM (“5% DMAHDM”);
- (4)
SBMP primer + 7.5% DMAHDM, SBMP adhesive + 7.5% DMAHDM (“7.5% DMAHDM”);
- (5)
SBMP primer + 10% DMAHDM, SBMP adhesive + 10% DMAHDM (“10% DMAHDM”);
- (6)
SBMP primer + 7.5% MPC + 5% DMAHDM, SBMP adhesive + 7.5% MPC + 5% DMAHDM (“7.5% MPC + 5% DMAHDM”);
- (7)
SBMP primer + 7.5% MPC + 7.5% DMAHDM, SBMP adhesive + 7.5% MPC + 7.5% DMAHDM (“7.5% MPC + 7.5% DMAHDM”);
- (8)
SBMP primer + 7.5% MPC + 10% DMAHDM, SBMP adhesive + 7.5% MPC + 10% DMAHDM (“7.5% MPC + 10% DMAHDM”).
2.3
Dentin shear bond testing
The use of extracted human teeth was approved by the University of Maryland. Extracted caries-free molars were first sawed to remove the crowns (Isomet, Buehler, Lake Bluff, IL), then ground perpendicularly to their longitudinal axes on 320 grit SiC paper until occlusal enamel was completely removed . The dentin surface was etched with etchant for 15 s and rinsed with water . A primer was applied, and the solvent was removed with an air stream. The adhesive was applied and light-cured for 10 s (Optilux-VCL401, Demetron, Danbury, CT). A stainless-steel iris, having a central opening with a diameter of 4 mm and a thickness of 1.5 mm, was held against the adhesive-treated dentin surface. The central opening was filled with a composite (TPH, Caulk/Dentsply, Milford, DE), and light-cured for 60 s . The bonded specimens were stored in distilled water at 37 °C for 24 h . The dentin shear bond strength, S D , was measured as previously described . A chisel was held parallel to the composite-dentin interface and loaded via a Universal Testing Machine (MTS, Eden Prairie, MN) at 0.5 mm/min until the composite-dentin bond failed. S D = 4 P /( πd 2 ), where P is the load at failure, and d is the diameter of the composite . Ten teeth were tested for each group, requiring a total of 80 teeth.
2.4
Degree of vinyl conversion
The degree of conversion (DC) of SBMP adhesive control and SBMP containing MPC and DMAHDM at the concentrations described above were measured by near-infrared (NIR) spectroscopy (Nicolet 6700, Thermo Scientific, Waltham, MA) . Specimens were photo-cured in a 4 mm diameter glass sample holder for 60 s. NIR spectra (3 specimens/group) were acquired before photo-cure and 24 h post-cure. DC was calculated from the percentage of change in the integrated peak area of the 6165 cm −1 methacrylate CH 2 absorption band normalized to thickness of the specimen before and after cure, following a previous study .
2.5
Specimen fabrication for protein adsorption and biofilm experiments
The dentin shear bond strength results showed that the 7.5% MPC + 7.5% DMAHDM group and the 7.5% MPC + 10% DMAHDM group had significantly lower dentin bond strengths than SBMP control. Therefore, 7.5% DMAHDM and 10% DMAHDM mass fractions were not used in the subsequent protein adsorption and biofilm experiments. The following four groups were used for the protein and biofilm tests:
- (1)
SBMP primer and adhesive control (termed “SBMP control”);
- (2)
SBMP primer + 7.5% MPC, SBMP adhesive + 7.5% MPC (“7.5% MPC”);
- (3)
SBMP primer + 5% DMAHDM, SBMP adhesive + 5% DMAHDM (“5% DMAHDM”);
- (4)
SBMP primer + 7.5% MPC + 5% DMAHDM, SBMP adhesive + 7.5% MPC + 5% DMAHDM (“7.5% MPC + 5% DMAHDM”).
The cover of a sterile 96-well plate (Costar, Corning Inc., Corning, NY) was used for fabricating disk specimens following previous studies . Briefly, 10 μL of a primer was placed in the bottom of each dent. After drying with a stream of air, 20 μL of adhesive was applied to the dent and photo-polymerized for 30 s, using a mylar strip covering to obtain a disk of approximately 8 mm in diameter and 0.5 mm in thickness. The cured disks were immersed in 200 mL of distilled water and magnetically-stirred with a bar at a speed of 100 rpm for 1 h to remove any uncured monomers . The disks were then sterilized with ethylene oxide (Anprolene AN 74i, Andersen, Haw River, NC) and de-gassed for 3 days .
2.6
Measurement of protein adsorption by micro bicinchoninic acid method
The amount of protein adsorbed on resin disks was determined by the micro bicinchoninic acid (BCA) method . Each disk was immersed in phosphate buffered saline (PBS) for 2 h. The disks then were immersed in bovine serum albumin (BSA) (Sigma-Aldrich) solutions at 37 °C for 2 h. The protein solutions contained BSA at a concentration of 4.5 g/L following previous studies . The disks then were rinsed with fresh PBS by stirring at a speed of 300 rpm for 5 min (Bellco Glass, Vineland, NJ), immersed in sodium dodecyl sulfate (SDS) at 1 wt% in PBS, and sonicated at room temperature for 20 min to completely detach the BSA from disk surfaces. A protein analysis kit (micro BCA protein assay kit, Fisher Scientific, Pittsburgh, PA) was used to determine the BSA concentration in the SDS solution. From the concentration of protein, the amount of protein adsorbed on the resin disk was calculated . Six disks were evaluated for each group.
2.7
Saliva collection for biofilm inoculum
The biofilm viability was investigated using a dental plaque microcosm model following previous studies . Saliva was collected from ten healthy adult donors having natural dentition without active caries or periopathology, and without the use of antibiotics within the last 3 months, following previous studies . The donors did not brush teeth for 24 h and abstained from food and drink intake for 2 h prior to donating saliva. Stimulated saliva was collected during parafilm chewing and was kept on ice. An equal volume of saliva from each of the ten donors was combined to form the saliva sample. The saliva was diluted in sterile glycerol to a concentration of 70%, and stored at −80 °C .
2.8
Dental plaque microcosm biofilm formation and live/dead assay
The saliva-glycerol stock was added, with 1:50 final dilution, to a growth medium as inoculum. The growth medium contained mucin (type II, porcine, gastric) at a concentration of 2.5 g/L; bacteriological peptone, 2.0 g/L; tryptone, 2.0 g/L; yeast extract, 1.0 g/L; NaCl, 0.35 g/L, KCl, 0.2 g/L; CaCl 2 , 0.2 g/L; cysteine hydrochloride, 0.1 g/L; hemin, 0.001 g/L; vitamin K1, 0.0002 g/L, at pH 7 . 1.5 mL of inoculum was added to each well of 24-well plates containing a resin disk, and incubated at 37 °C in 5% CO 2 for 8 h. Then, the disks were transferred to new 24-well plates filled with fresh medium and incubated. After 16 h, the disks were transferred to new 24-well plates with fresh medium and incubated for 24 h. This totaled two days of culture, which formed microcosm biofilms as shown previously .
Disks with 2-day biofilms were washed with PBS and stained using the BacLight live/dead kit (Molecular Probes, Eugene, OR) . Live bacteria were stained with Syto 9 to produce a green fluorescence, and bacteria with compromised membranes were stained with propidium iodide to produce a red fluorescence. The stained disks were examined using an inverted epifluorescence microscope (Eclipse TE2000-S, Nikon, Melville, NY). Six specimens were evaluated for each group. Three randomly chosen fields of view were photographed for each disk, yielding a total of 18 images for each group.
2.9
Lactic acid production and colony-forming unit (CFU) counts
Resin disks with 2-day biofilms were rinsed with cysteine peptone water (CPW) to remove loose bacteria . The disks were transferred to 24-well plates containing buffered peptone water (BPW) plus 0.2% sucrose. The disks were incubated in 5% CO 2 at 37 °C for 3 h to allow the biofilms to produce acid . The BPW solutions were then stored for lactate analysis. Lactate concentrations in the BPW solutions were determined using an enzymatic (lactate dehydrogenase) method, following a previous study . A microplate reader (SpectraMax M5, Molecular Devices, Sunnyvale, CA) was used to measure the absorbance at 340 nm (optical density OD340) for the collected BPW solutions. Standard curves were prepared using a lactic acid standard (Supelco, Bellefonte, PA) .
Disks with 2-day biofilms were transferred into tubes with 2 mL CPW, and the biofilms were harvested by sonication and vortexing (Fisher, Pittsburgh, PA) . Three types of agar plates were used to measure the CFU counts to assess the microorganism viability. First, tryptic soy blood agar culture plates were used to determine total microorganisms . Second, mitis salivarius agar (MSA) culture plates containing 15% sucrose were used to determine total streptococci . Third, MSA agar culture plates plus 0.2 units of bacitracin per mL was used to determine mutans streptococci . The bacterial suspensions were serially diluted, spread onto agar plates and incubated at 37 °C in 5% CO 2 for 24 h . The number of colonies that grew were counted and used, along with the dilution factor, to calculate the CFU on each resin disk .
2.10
MTT assay of metabolic activity of biofilms
A MTT (3-[4,5-dimethylthiazol-2-yl]-2,5-diphenyltetrazolium bromide) assay was used to examine the metabolic activity of biofilms . MTT is a colorimetric assay that measures the enzymatic reduction of MTT, a yellow tetrazole, to formazan. Disks with 2-day biofilms were transferred to a new 24-well plate, then 1 mL of MTT dye (0.5 mg/mL MTT in PBS) was added to each well and incubated at 37 °C in 5% CO 2 for 1 h. During this process, metabolically active bacteria reduced the MTT to purple formazan. After 1 h, the disks were transferred to a new 24-well plate, 1 mL of dimethyl sulfoxide (DMSO) was added to solubilize the formazan crystals, and the plate was incubated for 20 min at room temperature in the dark. After mixing via pipetting, 200 mL of the DMSO solution from each well was transferred to a 96-well plate, and the absorbance at 540 nm was measured via the microplate reader (SpectraMax M5) . A higher absorbance is related to a higher formazan concentration, which indicates a higher metabolic activity in the biofilm on the disk .
2.11
Statistical analysis
One-way and two-way analyses of variance (ANOVA) were performed to detect the significant effects of the variables. Tukey’s multiple comparison test was used to compare the data at a p value of 0.05.
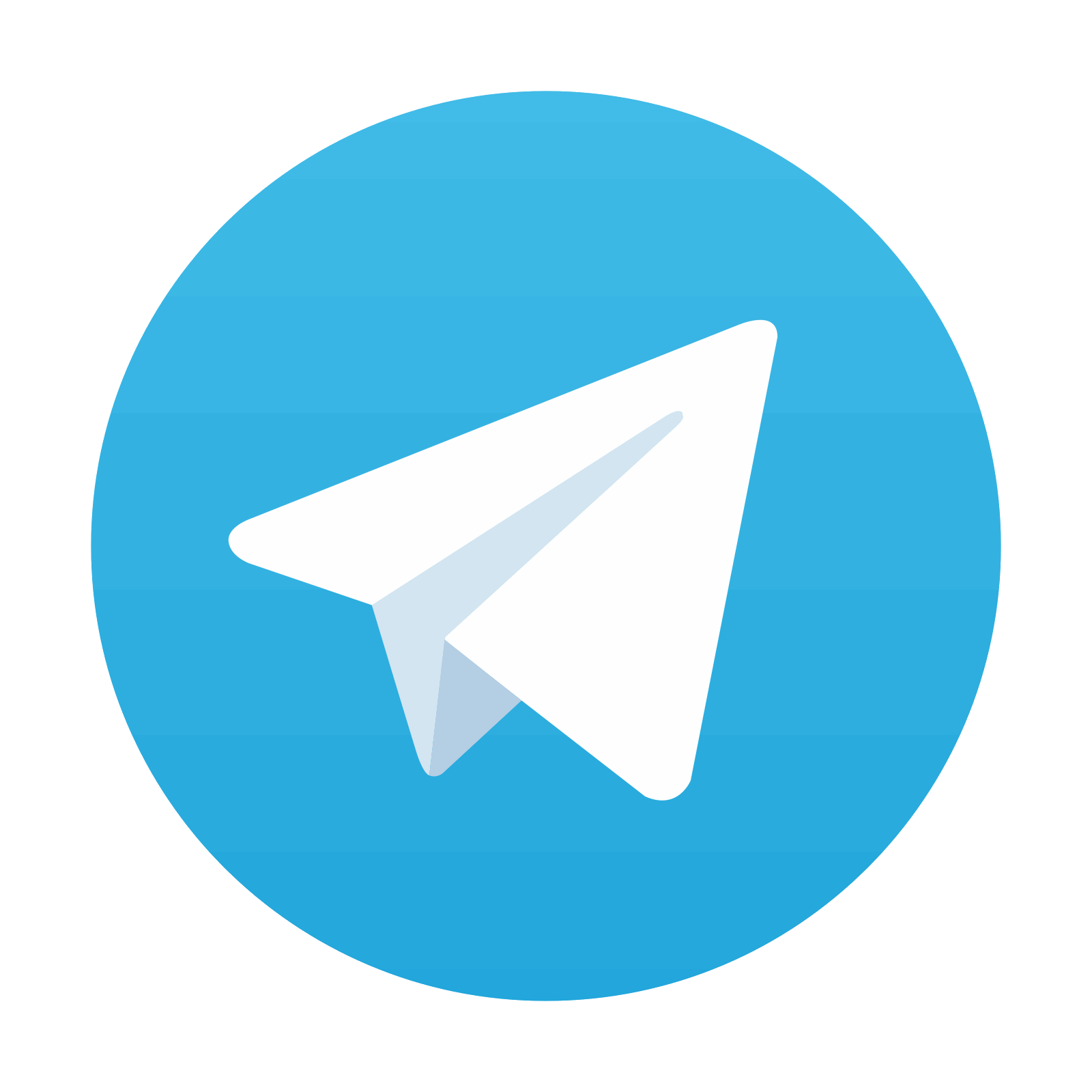
Stay updated, free dental videos. Join our Telegram channel

VIDEdental - Online dental courses
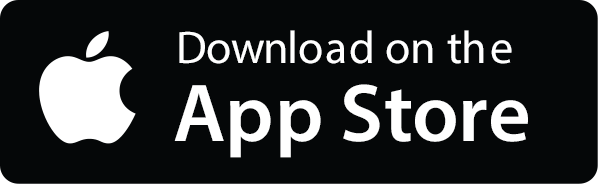
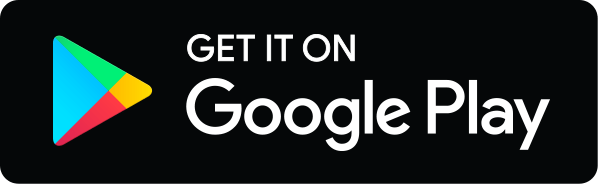