5
Lasers in Periodontology
Georgios E. Romanos
Stony Brook University, School of Dental Medicine, Stony Brook, NY, USA
5.1 Introduction
The main goal in the treatment of periodontitis is the removal of subgingival biofilm, bacterial endotoxins, and calculus, which are localized in the hard tissues, such as cementum, enamel, and exposed dentin. The main aim of the treatment is entire removal of calculus (scaling) and planing of the root surface (root planing) without unnecessary removal of healthy tooth structures. In addition, the removal of periodonto‐pathogenic bacteria, especially in areas not easily accessible to hand instruments, should be one of the main goals in the treatment of periodontal pockets. Biofilm penetrates the epithelium and the underlying connective tissue to a depth of 500 μm (Manor et al. 1984), releasing pro‐inflammatory cytokines, collagenases, elastases, and others, destroying the foundation of the periodontal support and leading to a continuous loss of attachment.
Deep periodontal pockets cannot be cleaned sufficiently; periodonto‐pathogenic bacteria support tissue destruction and, therefore, as a rationale of periodontal therapy a reduction of probing depth as well as gain of attachment should be considered. A sulcus with 1–3 mm probing depth controls the disease progression (Claffey and Egelberg 1995).
Nonsurgical and surgical periodontal therapy provide reconstruction of the periodontal sulcus (repair or regeneration), from the periodontal lesion having an inflamed pocket epithelium and connective tissue to a healthy periodontal support with a reattachment (reunion of the epithelium and connective tissue adherence with the root surface) and establishment of a long junctional epithelium or a new attachment (establishment of new stable connective tissue, which may include new cementum). Different studies in Rhesus monkeys performed by Caton and Nyman (1980) and Caton et al. (1980) at Eastman Dental Center in Rochester, New York, showed that different periodontal treatment modalities, such as periodic root planing in conjunction with soft tissue curettage and Widman flap procedure with and without bone grafts, resulted in the reformation of an epithelial lining along the treated root surfaces (long junctional epithelium), with absence of new connective tissue attachment. In any case, the formation of a long junctional epithelium was achieved at the same level as before treatment. Further studies showed also that the Widman flap surgical procedure does not increase the bone fill in infrabony defects, and no connective tissue attachment has been proven after this procedure in monkeys (Caton and Nyman 1980). A long junctional epithelium was established after this procedure as well.
Further analysis of the literature shows evidence that healing without or minimal connective tissue attachment but migration of a long junctional epithelium is possible in humans today (Cobb 1996).
The ideal concept might be the retardation of the long junctional epithelium in the defect after scaling and root planing (SRP), providing a new connective tissue attachment with adaptation of dense collagen fibers (Caton and Zander 1979) for a better periodontal support without gingival recessions and improvement of esthetics.
From the biological standpoint the removal of inflamed tissues, such as pocket epithelium and connective tissue, allows the host to better eliminate bacterial toxins and regenerate lost tissues in the periodontal apparatus. Therefore, many studies have tried to prove the importance of removal of the inflamed epithelium in the pocket using excision with a hand instrument (curettage), a scalpel (excision new attachment procedure, ENAP), or flap elevation (Widman surgical procedure) (Yukna et al. 1976; Ramfjord et al. 1987). The main objective in all these approaches is the reduction of probing pocket depth and bleeding on probing, bacteria elimination, and the improvement of connective tissue attachment.
Other surgical approaches to improve connective tissue attachment have been developed with high sensitivity and costs using barriers (Guided Tissue Regeneration, GTR) with or without bone grafting materials. The exclusion of the migration of the epithelium and connective tissue fibers in the periodontal lesion will be attempted, promoting new cementum with inserting collagen (Sharpey’s) fibers and also new bone formation. Even though GTR has a greater effect on probing reduction than open flap debridement, there is marked variability between studies, and the clinical relevance of these changes is unknown. Therefore, it is difficult to draw general conclusions about the clinical benefit of GTR and the factors affecting outcomes. There is a need to consider the predictability of this technique compared with other methods of treatment before making final decisions on use (Needleman et al. 2006).
However, a significant body of information has been produced in several clinical research centers evaluating the therapeutic responses of the periodontium to different treatment modalities. These studies provide evidence that periodontal therapy is effective in controlling periodontal inflammation, and that dentitions can be preserved for extended periods of time following treatments and proper maintenance. In general, there are no significant differences between the periodontal treatments evaluated. Therefore, the trend is from a resective to a more conservative, anti‐infective therapeutic approach and evaluation of the patient (Caffesse and Quiñones 1993).
Dental lasers may contribute to the field of periodontology, where other types of therapy and protocols cannot achieve the best clinical outcome. Even though there is limited evidence supporting laser‐mediated periodontal therapy over traditional treatment (Cobb 2006), there are reasons to include lasers in periodontal therapy as an adjunctive tool or as an alternative to conventional methods.
Due to the benefits of lasers, such as ablation or vaporization of the inflamed tissues, hemostasis and bactericidal effects may improve the periodontal condition and clinical outcomes. Less treatment time, less vibration and noise, as well as less operative pain, reduce the physical and mental stress of the patient and dentist (Ishikawa and Sculean 2007). Certainly, disadvantages like the costs of the equipment and the thermal side effects in conjunction with high risk of tissue destruction have to be considered for clinical application in daily routine practice.
There are two terms important for laser‐assisted periodontal therapy:
- Laser decontamination, which defines bacteria reduction within the inflamed periodontium, implant surfaces, and the beneficial effects in the biofilm and
- Laser coagulation, which means sealing of the blood vessels, capillaries, and lymphatic vessels in the inflamed tissues after laser decontamination.
Specifically, the following aims should be considered in the application of lasers during periodontal therapy:
- Bacteria reduction in the periodontal pockets
- Removal of dental calculus and subgingival hard deposits
- Root planing
- Removal of periodontal pocket epithelium and inflamed granulation tissue
- Retardation of the epithelial downgrowth in the defect
5.2 Laser‐Assisted Bacteria Reduction in Periodontal Tissues
The current treatment concept for the therapy of periodontal disease focuses on the control of supragingival plaque and subgingival microflora. The standard therapy is to achieve supragingival plaque and calculus removal and reduction (and if possible elimination) of the attached plaque and “swimmers” in the biofilm with curettes, as well as special modified ultrasonic scalers. A complete removal of the pathogenic bacteria is currently not possible. For about 95% of patients, it is sufficient if plaque is reduced to under a certain value, which, in conjunction with the host response, corresponds with a clinically healthy periodontium. The host is, in general, able to maintain a good periodontal clinical condition independent of the bacteria presence. For most patients, the progress of the disease is under control only through local bacteria and endotoxin reduction.
Lasers, whose irradiation is transferred by glass fibers or other delivery systems, could be therefore used in order to support therapy by decontaminating root surfaces by means of their photoablation and thermal effects. In addition, laser irradiation (specifically the Er:YAG laser) in conjunction with scaling and root planing (SRP) may not only control the microorganisms in the periodontal pockets but also may be considered as a prophylactic method against transient bacteremia (Komatsu et al. 2012).
There is a plethora of in vitro studies demonstrating bacteria reduction using lasers, and these studies define the radiation parameters (Table 5.1) (Tseng et al. 1991b; White et al. 1991; Cobb et al. 1992; Ando et al. 1996; Coffelt et al. 1997; Gokhale et al. 2012; Komatsu et al. 2012).
The bactericidal effect is explained primarily by the photothermal effects of the laser irradiation on the tissues. For instance, the irradiation with the excimer laser is absorbed mainly from the bacterial DNA. In this case, substantially low energy density is necessary in comparison to other lasers to achieve a bactericidal effect (Purucker et al. 1994).
Encouraged by these positive in vitro results, single in vivo studies were carried out. According to studies performed by Liu et al. (1999) with a Nd:YAG laser as an adjunctive tool (mechanical + laser), a further bacteria reduction of 25–40% is possible. This effect becomes particularly obvious on black‐pigmented microorganisms, because the laser irradiation is absorbed specifically by their melanin particles.
Working with high energies, which are necessary for the antibacterial effects, and without water‐cooling produces an undesirable thermal effect of the laser radiation. In experiments with rabbits, it was proven that the critical temperature for the bone is only 10 °C above the body temperature within a period of 1 minute (Eriksson and Albrektsson 1983). Another in vivo study, in which gutta‐percha was condensed in the root canal thermomechanically, showed that, in 28% of the cases cementum resorption was caused as a result of a temperature rise of about 18 °C (Saunders 1990). The study by Wilder‐Smith et al. (1995) proved that this destruction of periodontal structures also happens in treatment with the Nd:YAG laser (5 W power, 1100 J/cm2 energy density), in which a temperature rise was measured on the root surface of up to 22 °C after a treatment duration of 5 minutes with the Nd:YAG laser.
In vital teeth the applied thermal energy also affects the pulp tissue. According to Wilder‐Smith et al. (1995), temperature rises up to 36 °C were measured in the pulp with Nd:YAG laser. These temperature rises are strongly dependent on the thickness of the dentin at the area of the laser application. Studies with Nd:YAG lasers have yielded temperature rises of 27 °C, with only 100 J/cm2 (duration 30 seconds), if the dentin thickness is only 0.5 mm (White et al. 1994). A study with the Er:YAG laser revealed that an applied energy density of 2000 J/cm2 (duration 20 seconds) caused a temperature rise of 18° C in the pulp (Aoki et al. 1994). This is due to the lower penetration depth of this laser radiation due to the tooth’s hard substance (Table 5.2). The high temperatures resulting from the treatment with the laser are not acceptable. The corresponding water‐cooling is therefore absolutely necessary, and similarly also in the use of ultrasonic scalers.
Table 5.1 Bactericidal effect of laser irradiation in vitro.
Study | Laser Wavelength | Bacteria | Power Density |
---|---|---|---|
Tseng et al. (1991b) | Nd:YAG | no defined | No defined |
White et al. (1991) | Nd:YAG | B. subt., E. coli | No defined |
Cobb et al. (1992) | Nd:YAG | A.a., P.g., P.i. | No defined |
Purucker et al. (1994) | Excimer | A.a., P.g., P.i. | 13 mJ/cm2 |
Ando et al. (1996) | Er:YAG | A.a., P.g. | 0.3 J/cm2 |
Coffelt et al. (1997) | CO2 | E. coli | 11 J/cm2 |
Gokhale et al. (2012) | Er:YAG | Anaerobes | No defined |
Komatsu et al. (2012) | Er:YAG | P.i., P.g., T.f., T.d.,F.n. | No defined |
Table 5.2 Temperature increase during laser irradiation in vitro.
Study | Laser Wavelength | Power Density | Time | Pulp | Root |
---|---|---|---|---|---|
Aoki et al. (1994) | Er:YAG | 2000 J/cm2 | 20 sec | 18 °C | 39 °C |
White et al. (1994) | Nd:YAG | 100 J/cm2 | 30 sec | 6–43 °C | – |
Wilder‐Smith et al. (1995) | Nd:YAG | 1100 J/cm2 | 300 sec | 36 °C | 22 °C |
The bactericidal effects of the Nd:YAG laser (in vivo) on root surfaces and on the subgingival flora have been studied very early (Cobb et al. 1992; Ben Hatit et al. 1996). Other studies present contradictory results of the use of the Nd:YAG laser in periodontal therapy with no benefits to traditional SRP (Liu et al. 1999). However, a relatively recent review of the literature has been conducted to evaluate the evidence of the use of the Nd:YAG laser, supporting this treatment modality as mono‐therapy or in conjunction with conventional nonsurgical therapy (SRP). The review showed poor evidence of the benefits of the pulsed Nd:YAG laser in the treatment of periodontal disease (Thomas and Shafer 2011).
In contrast to the pulsed Nd:YAG laser, the bactericidal effects of the 980 nm diode laser (2.5 W, continuous wave in contact with the pocket epithelium in the pocket for subgingival curettage) as an adjunct to mechanical debridement were compared to conventional debridement and periodontal flap procedure and showed a greater reduction of the anaerobic bacteria in comparison to the control group (Gokhale et al. 2012).
Besides this thermal effect, changes on the root surfaces are also caused when using the Nd:YAG and CO2 laser. When using the CO2 laser for bacteria reduction in the periodontal tissues, damages, such as craters and microcracking, but also melting of the surface with increased porosity, can also occur with energy density levels of 41 J/cm2 (Coffelt et al. 1997). Other studies have revealed that these damages lead to a bioincompatibility of the root surface for fibroblasts (Table 5.3) (Morlock et al. 1992; Trylovich et al. 1992; Thomas et al. 1994; Radvar et al. 1995; Wilder‐Smith et al. 1995; Coffelt et al. 1997). In contrast to these studies Crespi et al. (2002) showed an increased fibroblast attachment when root surfaces were irradiated with a defocused CO2 laser, in pulsed mode with a low power of 2 W combined with mechanical instrumentation (ultrasonics) and was suggested as a useful tool for root conditioning.
Table 5.3 Root surface changes due to laser irradiation in vitro.
Study | Laser Wavelength | Power Density | Changes |
---|---|---|---|
Morlock et al. (1992) | Nd:YAG | No defined | Craters, melting |
Trylovich et al. (1992) | Nd:YAG | No defined | Craters, melting, Lack of biocompatibility |
Thomas et al. (1994) | Nd:YAG | No defined | Lack of biocompatibility |
Radvar et al. (1995) | Nd:YAG | No defined | Melting, porosity |
Wilder‐Wilder‐Smith et al. (1995) | Q‐switched Nd:YAG | 922 J/cm2 | Craters, melting, substrate destruction |
Coffelt et al. (1997) | CO2 | 41 J/cm2 | Craters, melting, porosity |
With the evidence yielded by these studies, there is doubt whether treatment of the root surface with the currently available laser technologies in comparison to conventional therapy facilitates an improvement of the clinical outcome or leads to a more intense bacteria reduction.
5.3 Removal of Subgingival Calculus
The removal of calculus with hand instruments is a difficult technique, which requires advanced training and can be performed correctly only by well‐trained, experienced clinicians. In addition to that, it is a procedure which cannot be tolerated by high number of patients due to discomfort. In contrast to that, the use of lasers to remove calculus seems to be a relatively simple technique with many advantages.
Tseng et al. (1991a) carried out the first studies with an Nd:YAG laser on freshly extracted molars. Although no better dental calculus removal was proven, there was a significant reduction of the processing steps which are necessary for its removal.
Selective dental calculus removal is in vitro possible with a Q‐switched Nd:YAG laser using a certain energy density. Nevertheless, this laser is not yet available for dental applications. Furthermore, the removal rates, with the necessary settings for selective work, are very low. The excimer laser with wavelength of 193 nm also may be used for selective calculus removal without causing damage in the root structure (Frentzen et al. 1992).
Additional studies also showed the positive effects in the removal of calculus using the frequency‐doubled alexandrite laser (Rechmann and Henning 1995). The solid‐state laser with a wavelength 337 nm, pulse duration 100 ns, removes dental calculus without damage to the cementum. However, recent experimental studies provide further information that a specific laser wavelength of 400 nm may be more efficient for calculus removal (Schoenly et al. 2010). Based on these studies with a scanning electron microscope (SEM), the 400 nm laser appears to be efficient for calculus removal resulting in a root surface more suitable for repair (Figure 5.1) in comparison to the conventional methods, such as use of ultrasonics and hand instruments (Schoenly et al. 2013).

Figure 5.1 Removal of calculus in vitro using a new laser with wavelength of 400 nm.
Source: (IPG Photonics, Oxford, MA.), J. Schoenly, PhD (IPG Photonics, Oxford, MA).
All above laser wavelengths have no clinical applications, since they do not exist in the dental market today.
The only clinically used lasers for calculus removal are the Er:YAG laser (2940 nm) or the Er,Cr:YSGG laser (2790 nm) and the more recent CO2 laser SOLEA (9300 nm). In vitro studies of Aoki et al. (1994) in Japan have shown the effective removal of calculus with an Er:YAG laser (Figure 5.2). The pulsed Er:YAG laser used with water irrigation was able to remove subgingival calculus from the tooth root effectively at an energy level of about 30 mJ/pulse (energy density: 10.6 J/cm2/pulse) and 10 pps, under in vitro conditions. Ablation of the tooth substance on laser‐assisted scaling was generally observed within the cementum. However, the angle of the working tip of the Er:YAG laser seems to influence the amount of root substance removal and determines the clinical outcome. Angles of the working tip less than 30° are beneficial without damage to the root surface (Folwaczny et al. 2001).

Figure 5.2 Calculus removal using the Er:YAG laser on an extracted tooth.
Source: Dr. Georgios E Romanos.
On the other hand, root surfaces temperatures rise to 39 °C, without cooling. On the pulp wall, there is a temperature rise of 18.4 °C. In order to avoid damage to the surrounding periodontal tissues and the pulp, water‐cooling is of importance. Also problematic is the control of the substance removal, because not only hard deposits, but also dental hard tissue (cementum, dentin) is removed. Depending on the pulse energy (30–120 mJ/pulse, 10 pps frequency), the ablation depth of the dentin can be 74–136 μm (Aoki et al. 1994). This was found in studies of teeth of Asian patients. Because a potential complete removal of the cementum in the root surface cannot be avoided, an electronic control of lasers would be a possible solution for future clinical practice.
Other studies on calculus removal were performed by German groups of scientists and demonstrated the advantages of the Er:YAG laser for removal of subgingival calculus (Keller and Hibst 1995). Studies also showed increased loss of cementum and dentin, demonstrating roughened surfaces, which should be taken into account in clinical conditions (Frentzen et al. 2002). The used parameters were set at 160 mJ/pulse and frequency of 10 Hz. It has to be noted that there is a possible genetic difference in the cementum thickness between Caucasian and Asian teeth.
In an in vivo application of the laser, a sapphire tip (for the erbium family lasers) was used to remove calculus, which is in contact with the root surface or on an angle less than 30° in nonsurgical or surgical therapy to avoid deep crater formation (Folwaczny et al. 2001). Nevertheless, on the interproximal surfaces, there is no possibility of direct irradiation, in spite of the sufficient access, because the “side‐firing fibers,” which occur at the tip of the beam applicator with a deflection mirror, are not yet available.
Initial clinical studies presented an increase in attachment after nonsurgical periodontal therapy with an Er:YAG laser and maintenance of the clinical parameters for up to two years and is comparable to conventional scaling (Schwarz et al. 2003).
However, clinical studies by Sculean et al. (2004a) with nonsurgical periodontal therapy showed that sites treated with an Er:YAG laser demonstrated a mean gain of clinical attachment similar to a group where ultrasonics were used at three and six months. No statistically significant differences were observed between the groups of treatment, and both therapies led to significant improvements of the investigated clinical parameters.
In contrast to these studies, a flap surgery and use of an Er:YAG laser (160 mJ, 10 Hz) in comparison to the use of ultrasonics in conjunction with surgical treatment showed at six months after treatment that both therapies led to significant improvements of the clinical parameters. The Er:YAG laser may represent a suitable alternative for root surface and defect debridement in conjunction with periodontal surgery (Sculean et al. 2004b).
Additional studies showed positive effect using the Er,Cr:YSGG laser in the treatment of intrabony defects associated with chronic periodontitis and minimal invasive nonsurgical (closed) procedure. Specifically, the study was performed by Al‐Falaki et al. (2016) and showed significant reduction in intrabony defect depth but no change in suprabony bone height. More than half (9 of 15) of the examined defects presented more than 50% bone fill. The Er,Cr:YSGG laser was used with a 14 mm 500 μm radial firing periodontal tip (RFTP5, Biolase, Irvine, CA, USA) and 1.5 W power, 30 Hz frequency, and 20 : 11% (water: air) ratio. The tip was placed parallel to the long axis of the tooth into the base of the pocket, moving in vertical and horizontal movements as long as granulation tissue was debrided from the pocket. Deepithelization occurred using a 50 Hz frequency outside the periodontal pocket to the level of the mucogingival junction to be able to eliminate the epithelial cells and control the epithelial proliferation (downgrowth).
In contrast to the previous laser wavelengths, studies showed that the conventional CO2 laser in the periodontal pocket may lead to alterations in the root surfaces and charring or carbonization. Modifying the pulse duration and using microsecond pulse lengths, Vaderhobli et al. (2010) were able to show histologically in porcine oral mucosa and periodontal tissues no damage of the hard tissues, such as root surface, enamel, and bone during sulcular debridement. In addition, the coagulation zone was less than 100 nm. With the clinically effective ablation of the pocket epithelium, there was not damage of the periodontium or the pulp. This effect is due to the increased tissue relaxation time with the use of microsecond pulses during the laser irradiation.
5.4 Removal of Pocket Epithelium
For about 5% of periodontitis patients it is advisable to make the elimination of plaque easier by removal of the pocket epithelium and the inflamed connective tissue to prevent a recurrence of the lesion. This inflamed tissue can be removed by irradiation of the periodontal pocket walls with an Nd:YAG laser.
Successful results in intraoral soft tissue applications were reported by White et al. (1991), which showed specifically pocket depth reduction, and less postoperative bleeding, inflammation, and pain after the use of the Nd:YAG laser compared to scalpel surgery. Similarly, we demonstrated positive effects of the Nd:YAG laser use in oral soft tissue and periodontal applications (Romanos 1994a). We were able to observe relatively early a slower epithelial migration and proliferation in the periodontal sites in a patient with acute necrotized ulcerative gingivitis (1.75 W and 20 pps on the contact probe) (Romanos 1994b). In comparison to the conventional gingivectomy with the gingivectomy knife, the laser glass fiber removes the sulcular and oral epithelium of the pocket presenting a slower formation and apical migration of the epithelial layers compared to the conventional excision (Romanos 1994b). This was explained due to the delayed wound healing associated with the laser, due to heat transfer. After this observation was made in more clinical cases, we were able to evaluate in vitro the impact of the laser on the epithelial proliferation.
The lack of pocket epithelium immediately after laser curettage was also histologically proven in a study by Gold and Vilardi (1994). Specifically, in patients with moderate periodontal pockets, a Nd:YAG laser was used with a frequency of 20 Hz, pulse duration of 150 μs, power of 1.25, and 1.75 W (representing the energy density of 70 and 120 J/cm2 with the use of a fiber 320 μm in diameter) and treatment duration of two to three minutes per tooth. With both implemented powers, biopsies of the soft tissues (taken immediately after irradiation) showed that the pocket epithelium could be completely removed without causing detectable damage (at the light microscopical level) of the underlying connective tissues.
New studies using an Nd:YAG laser with water‐cooling compared short‐term effects of the pulsed Nd:YAG laser as an adjunct to SRP in the treatment of periodontitis. The clinical improvement was significantly higher in the lased group, and samples of gingival crevicular fluid (GCF) showed specifically a significant reduction of IL‐1b and MMP‐8 after the use of the pulsed Nd:YAG laser (average power: 4 W; energy: 80 mJ/pulse; frequency: 50 Hz; fiber diameter: 600 μm) compared to SRP alone (Qadri et al. 2010). The authors cannot easily explain the reason for improvement of the periodontal status after the use of the laser glass fiber in the periodontal tissues but discuss the significance of the partial removal of the pocket epithelial lining as a contributing factor for the decrease of periodontal inflammation and less discomfort for patients in these sites (Qadri et al. 2010). In addition, the long‐term effects (after 20 months) of the single application of an Nd:YAG laser in combination with SRP had positive effects on periodontal health compared to treatment by SRP alone (Qadri et al. 2011).
Additional studies in animal in vitro models using the glass fiber (300 μm) of a 980 nm diode laser for soft tissue curettage have been used in comparison to conventional curettes (Figure 5.3). The study showed a complete removal of the epithelium (Figure 5.3a–c) within the pocket after use of a 980 nm diode laser (2 W, CW) but no epithelial removal after the use of hand instruments (Figure 5.3d). This epithelial removal was achieved in all cases with the laser and independent of the experience level of the clinicians (Romanos et al. 2004).
![Photos depict the removal of the long junctional epithelium in ex vivo conditions in the bovine periodontium (treatment provided by [a] a clinician with complete training in periodontology and oral surgery; [b] an oral surgeon; [c] a general dentist); (d) control debridement with a curette shows still presence of the long junctional epithelium.](https://i0.wp.com/pocketdentistry.com/wp-content/uploads/2022/11/c05f003.jpg?w=960)
Figure 5.3 Removal of the long junctional epithelium in ex vivo conditions in the bovine periodontium (treatment provided by [a] a clinician with complete training in periodontology and oral surgery; [b] an oral surgeon; [c] a general dentist); (d) control debridement with a curette shows still presence of the long junctional epithelium.
Source: Dr. Georgios E Romanos.

Figure 5.4 Mean PPD over time and mean CAL over time. Clinical parameters after the use of a 980 nm diode laser in the periodontal pocket in aggressive periodontitis patients. The laser‐assisted SRP reduced significantly the PPD and the loss of attachment (Kamma et al. 2009).
Clinical studies using a 980 nm diode laser as an adjunct tool to SRP showed a moderate qualitative improvement over traditional treatment in the clinical indices with significant reduction of sensitivity and less discomfort during treatment (Borrajo et al. 2004). In addition, no detectable surface alterations and no signs of thermal side effects in any of the treated teeth were observed (Castro et al. 2006).
A significant contribution in the literature is the clinical and microbiological study performed by Kamma et al. (2009), who showed a significant reduction of periodonto‐pathogenic bacteria in patients with aggressive periodontitis using a 980 nm continuous wave laser and 2 W average power (Figure 5.4).
Due to the effects of laser decontamination and laser coagulation, the vascularization of the periodontal tissues will be reduced, the water content will be reduced, and the tissue becomes firm and less vascularized. Like the Nd:YAG laser, the diode lasers have a relatively high affinity for chromophores in pigmented tissues, which results in good hemostasis. In addition, there is higher penetration in the tissue and higher resorption by black‐pigmented bacteria compared to the Er:YAG and CO2 lasers. Therefore, irradiation using these wavelengths is more selective to the periodonto‐pathogenic bacteria and removal of the epithelial lining in the pocket.
Retrospective analysis of clinical cases after the use of laser‐assisted nonsurgical periodontal therapy after 10 years in deep periodontal pockets with more than 6 mm PPD was published by Roncati et al. (2017). The application of an 810 nm pulsed diode laser with 1 W power (mean: 0.5 W; 10 Hz; fiber 320 μm) or a 980 nm pulsed diode laser with 2.5 W power (mean: 0.75 W; 15 kHz; fiber 400 μm) had a significant improvement of the periodontal clinical parameters over the long term.
Also, in a high number of clinical cases (2683 patients in a multicenter study), the microbial profiling of the periodontal pockets was evaluated after the use of an Nd:YAG laser. This recent study demonstrates for the first time the efficacy of the laser‐assisted periodontal therapy based on the microbial response of the tissue to the laser therapy (Martelli et al. 2016).
Nevertheless, more research is necessary to prove that the removal of inflamed granulation tissue from osseous periodontal defects is also possible without simultaneous damage to the surrounding healthy periodontal structures due to the deep tissue penetration. This applies particularly to the treatment of deep vertical and furcation defects.
The following protocol is suggested for removal of pocket epithelium and inflamed periodontal tissues:
After the initial phase of periodontal therapy (hygiene phase) and evaluation of the clinical condition, scaling and root planing will be performed to establish a smooth cementum, free of biofilm and calculus, in order to achieve a new attachment. Laser‐assisted SRP will be performed according to the following protocol:
- Topical anesthesia, low power laser irradiation (1 W for pulsed Nd:YAG and maximum 2 W for diode lasers in a continuous mode) or pulsed mode using the 300–400 μm glass fiber in order to enlarge the pocket for better access. An initiated tip allows excision of the soft tissue (ablation of the inner part of the pocket epithelium will be accomplished).
- The next step includes corono‐apical movements within the pocket in a depth of approximately 3 mm. Laser coagulation during irradiation and decontamination can be achieved at the same time. In case of sensitivity or pain, local anesthesia is indicated. A noninitiated tip allows diffused energy transfer into the connective tissue to reduce bacteria and exudation.
Figure 5.5 Schematic illustration of the laser fiber movement within the pocket for coagulation and decontamination.
- Removal of calculus with an Er:YAG laser or ultrasonics in conjunction with hand instruments (scalers and curettes) for root planing. Due to instrumentation, spontaneous bleeding is initiated.
- The final step of the treatment is irradiation and coagulation (bleeding control) with the fiber using corono‐apical movements in simultaneous mesio‐distal orientation (Figure 5.5) using 1.5–2 W average power with a frequency of 50–70 Hz for the pulsed Nd:YAG (fiber 300–400 μm), or 2–3 W (CW) or 4 W (pulsed mode, 50 Hz) for the diode laser (fiber 400 μm), after initiation (with an articulating paper). If the fiber is coated with debris, the fiber has to be cleaved and recalibrated. This laser procedure will be done in the maximum depth (based on PPD measurement), reduced 1–2 mm with the glass fiber in the pocket.
At this final step, removal of the epithelium migrating into the pocket, the so‐called sulcular epithelium, will be performed, as well as stabilization of the blood clot within the pocket using the laser. This clot represents a biological barrier in the wound, which does not allow migration of the epithelium in the wound (Figure 5.6). For pockets more than 4–5 mm deep, a removal of the oral epithelium (outer surface of the pocket is also recommended (deepithelialization). This will be done only in deep pockets and should not be utilized in every pocket.
- During laser irradiation the fiber will be kept in contact with soft tissues (laser curettage) and in parallel position to the root surface. In case of the CO2 laser application, the perio‐tip will be positioned in a similar way within the pocket (Figure 5.7).

Figure 5.6 Schematic illustration of the periodontal wound healing after laser irradiation of the pocket.
The Er:YAG laser sapphire, chisel‐type tip is used in contact with the root surface also performing calculus removal and simultaneously root planing (Figure 5.8).
For localized deep pockets (advanced periodontal lesions) a deepithelialization should be performed every 7–10 days (see also Section 5.5) or a flap surgery in conjunction with laser treatment may be indicated. In case of nonsurgical laser‐assisted therapy, the deepithelialization should be performed without inserting the fiber in the newly formed sulcus. Only the oral epithelium should be ablated with the laser at this time.

Figure 5.7 CO2 laser irradiation in the pocket as well as deepithelialization technique.
Source: Dr. Georgios E Romanos.
- Routine postoperative instructions, such as rinsing with salt water as well as careful toothbrushing with a soft toothbrush using gentle pressure, are recommended. Interdental flossing is indicated with special care to avoid tissue irritation for the first four to seven days of healing. Analgesics will be used as needed. Avoidance of crunchy food that could irritate the tissue and jeopardize the healing is advised. Mouthwashes may be used as well.
The role of the initiated and noninitiated tips is fundamental, every clinician must have a clear understanding of the laser effects and must be proficient in reading laser‐tissue interactions (Smith 2011).
Modification of this technique was described by Gregg and McCarthy (1998) using the PerioLase pulsed Nd:YAG dental laser for laser curettage with the name laser periodontal therapy (LPT). This technique was developed based on the master’s thesis of M. E. Neill (1997) and a previous study published by Neill and Mellonig (1997) from University of San Antonio, TX, who evaluated the effects of the pulsed Nd:YAG laser using the PulseMaster 1000 Dental Laser. This study showed improvement of the clinical parameters after the use of a low power Nd:YAG laser in conjunction with scaling root planing and 2 W power (80 mJ energy and 25 Hz frequency) for an average of two minutes of tooth irradiation. In addition, the impact of this laser irradiation was positive on the bacteria (specifically on the Porphyromonas gingivalis and Prevotella intermedia) reduction.
Modifying this method, using the LPT with the PerioLase digital laser, the initial pocket debridement occurs with a 6 W true pulse free running (FR) Nd:YAG laser (PerioLase MVP‐7) and 100–150 μs pulse duration to remove the pocket epithelium and periodontal pathogens. After use of ultrasonics and hand instruments for removal of calculus the pulse duration will be increased from 450 to 650 μs in order to create a blood clot and clot stability in the pocket. The promoters of the technique recommend occlusal adjustment after laser therapy.

Figure 5.8 Schematic illustration of the laser tip within the pocket for removal of calculus.
Further data from the use of this technique seem to show no significant differences between the surgical periodontal therapy and the LPT in private practice, providing benefits for patients who do not like to undergo surgical treatment (Harris et al. 2002).
The first report presenting histologic results in humans following a laser‐assisted new attachment procedure (LANAP) for the treatment of periodontal pockets was published by Yukna et al. (2007). All teeth were scaled and root planed with ultrasonic and hand scalers. One of each pair of teeth received treatment of the inner pocket wall with a free‐running pulsed Nd:YAG‐laser to remove the pocket epithelium, and the test pockets were lased a second time to seal the pocket. The clinical parameters showed better PPD and CAL compared to the control teeth. After three months, all treated teeth were removed en bloc for histologic processing. All laser‐assisted treated specimens showed formation of new cementum and connective tissue attachment and occasionally coronal to the notch, whereas five of the six control teeth had a long junctional epithelium with no evidence of new attachment or regeneration.
Recent studies also showed that this technique may induce periodontal regeneration, but there is need for further investigation with long‐term clinical trials to compare the stability of clinical results to conventional therapy (Nevins et al. 2012). In this histological report five from ten examined teeth were evaluated histologically presenting evidence of some degree of periodontal regeneration with new cementum, periodontal ligament, and alveolar bone.
5.5 Retardation of the Epithelial Downgrowth
The retardation, or delay, of epithelial downgrowth is a basic concept for potential gain of new connective tissue attachment in periodontal tissues. With the use of the CO2 laser a removal of pocket epithelium is possible without damage of the underlying connective tissue (Rossmann et al. 1987). In experimental studies with monkeys, it was proved that using the CO2 laser inside and outside the pocket, the sulcular and oral epithelium was removed to the level of the mucogingival junction, and therefore epithelial cells were absent in the periodontal apparatus. Since epithelium was absent, a long junctional epithelium formation within the pocket is impossible if ablation of the newly formed epithelium (out of the pocket) was removed every 7–10 days. Therefore, a delay of the epithelial downgrowth of approximately 14 days was achieved (Istael et al. 1992). Another study with two patients having hopeless teeth also showed a delay of epithelial migration according to formation of a new connective tissue attachment instead of long junctional epithelium.
Based on this concept, the oral epithelium was removed with a CO2 laser on the day of the surgical intervention as well as 10, 20, and 30 days postsurgically. In order to confirm the complete removal of the epithelial cells, a wet gauze soaked with saline will be used (Figure 5.9) in a similar manner as previously described in the ablation of leukoplakia (see also Chapter 3). Clinical case series with two‐ to three‐wall intrabony defects were published, presenting an improvement of bone fill and the clinical parameters (with or without osseous grafting) in conjunction with the deepithelialization technique (Rossmann and Israel 2000).
Further studies showed that direct irradiation of root surfaces using a defocused CO2 laser induces fibroblast attachment without melting and deleterious changes of the root surface. This is the result of in vitro studies (Barone et al. 2002; Crespi et al. 2002) based on animal preclinical experiments demonstrating the positive effects of the CO2 laser irradiation in periodontal tissues and the improvement of periodontal regeneration after irradiation (Crespi et al. 1997). Specifically, class III furcation defects in beagle dogs were treated with a defocused, pulsed CO2 laser (with 2 W power). This treatment induced the formation of new periodontal ligament, cementum, and bone.
Recent clinical case series demonstrated the clinical protocol of the CO2 laser irradiation on the root surface in conjunction with the deepithelialization technique to improve bone regeneration (Crespi et al. 2004). The predictability of the CO2 laser‐assisted technique in comparison to the traditional Widman flap procedure was published recently after 15 years of follow‐up (Crespi et al. 2011).

Figure 5.9 Removal of the oral epithelium after CO2 laser irradiation (a) using a wet gauze (b) in order to produce bleeding from the underlying connective tissue and final hemostasis (c).
Source: Dr. Georgios E Romanos.
Since this laser‐assisted procedure aims to remove specific types of cells, control cell proliferation, and promote the healing of other types of cells, we named this surgical treatment concept laser‐assisted guided tissue regeneration (LA‐GTR), which can be used within the periodontal pocket (Figure 5.10) but also in other areas and indications in surgical procedures. Specifically, this technique has been applied to increase the width of keratinized tissues instead of harvest a gingival graft from the palate (FGG), with predictable long‐term results (see also Figure 5.17).
The glass fiber during irradiation has to be inspected carefully to avoid side effects, such as breakage and undetected exposures of energy to the surrounding tissues.
A cleaving of the fiber means a 90‐degree surface at the terminal end of the fiber which can be achieved with special cleaving tools, such as ceramic scissors or glass‐cutting pens. An optimal cleave with a well‐defined solid circular appearance of the laser light is a prerequisite for a high‐quality laser irradiation with maximum energy delivery in the target tissues.

VIDEdental - Online dental courses
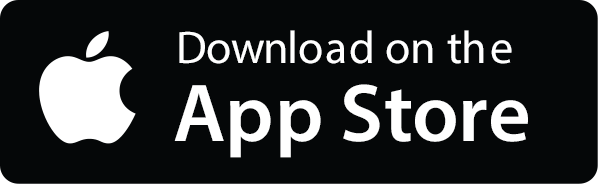
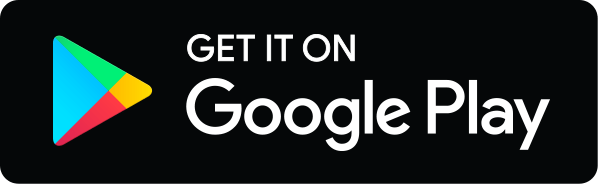