4
Lasers and Bone Surgery
Georgios E. Romanos
Stony Brook University, School of Dental Medicine, Stony Brook, NY, USA
4.1 Introduction
Medical advances for bone surgical procedures are, among others, facilitated by suitable and practice‐oriented drilling and bone‐cutting techniques. A promising alternative to drills, microsaws, trephines, and piezosurgery for osteotomy procedures (Al Asseri and Swennen 2018) is bone osteotomy using lasers.
Particularly in the oral cavity, the use of lasers is expected to result in a better quality of osteotomies due to the accessibility of the relatively small size of handpieces and laser delivery systems in intraoral conditions. The requirements of lasers for applications in the bone are much higher than of those for the soft tissues since complete bone evaporation is possible only with very high temperatures, because of the high mineral content of the bone.
In vitro osteotomies using human bone initially used industrial lasers, such as a 250‐watt (W) carbon dioxide (CO2) CW laser (Engelhardt and Bimberg 1972). Later on, experimental medical CO2 CW systems were introduced as well as pulsed (superpulsed) CO2 lasers in the ms range. Some studies were focused on the differences in the healing process among different CO2 lasers, as well as comparisons to conventional osteotomies (Moore 1973; Gertzbein et al. 1981; Walsh et al. 1989). It has been reported that an intense carbonized zone at the osteotomy margins using CO2 CW lasers is associated with the delay of wound healing. Therefore, mathematical models have been developed to control temperature rise and keep the temperature in constant conditions under CO2 laser application (Lévesque et al. 2015; Levesque and Robaczewski 2017). Here, we demonstrate advances of laser‐assisted bone osteotomies using different wavelengths or using shorter pulse widths, particularly in the μs‐range.
4.2 CO2 Laser
Clayman et al. (1978) demonstrated the influence of the CW and pulsed (superpulsed) CO2 laser on bone healing in rabbits. In both groups, bone formation could be detected after four weeks, which was completed after two months with simultaneous formation of lamellar bone. The only significant clinical difference between the two laser systems is the much higher average speed of the osteotomy with the superpulsed CO2 laser, with a pulse energy of 5 J, at an average power of 7 W, a pulse width of 50–200 ms, and a repetition rate of 25–250 Hz, compared to a CO2 CW laser with energy of 10 J. The results of this study were compared to the wound healing processes with the use of high‐speed drills on rabbit bone. It was found that the average quality of the incision with laser application was much better, and effective hemostasis could be achieved; yet the healing of the bone was significantly affected (Clayman 1976).
Studies performed by Small et al. (1979) in the rabbit tibia compared CO2 laser osteotomies (both in the continuous and in superpulsed mode) with conventional drilling methods and concluded that an intense carbonized zone was formed at the laser‐osteotomy, which during the laser use should be removed in order to allow better quality of the cut. The superpulsed lasers (with a maximum average output power of 6 W) showed a delay in wound healing by two to four weeks, in comparison to the CW laser systems with a maximum output power of 10 W. Significantly less lamellar bone formation and lower carbonization zone were shown. Cutting of the bone with 10 W CO2 CW lasers was not possible (Small et al. 1979).
The bone healing processes were found to be fastest after conventional osteotomy, due to the reduced necrosis and lack of carbonization. A possible reason for these delayed healing processes after laser application is the strong thermal damage of the bone with the use of CW systems or systems that are pulsed in milliseconds. Another disadvantage of CO2 lasers (CW or pulsed) is the limited visibility during the osteotomy due to the carbonization zone.
In summary, it has been proven that neither industrial nor medical CW and superpulsed CO2 lasers for processing of bone have brought the desired results. The much‐cited CW or superpulsed CO2 laser cannot be used clinically, due to the thermal stressing of bone. Therefore, different laser systems, in particular with shorter pulse lengths, should be evaluated that would cause lower thermal damage on the bone during the osteotomy process.
Recent studies by Augello et al. (2018) showed that in adult sheep, using micro‐CT and histological analysis, more mineralized bone was formed in the laser group compared to the piezoelectric group.
Also, Kuttenberger et al. (2008) used a technically demanding procedure to perform laser osteotomies in sheep tibia. The osteotomy depth was possible at a depth of 20 mm without visible thermal damage to the bone. The computer‐guided CO2 laser osteotomy demonstrated clinical and radiological healing patterns comparable to those seen with osteotomy done by standard mechanical instruments. The authors concluded that through the use of computer guidance, extremely precise osteotomies and sophisticated cut geometries are possible using this technique. For practical applications, precise control of the depth of laser cutting and easier manipulation of the osteotome are required.
Studies using transversaly excited atmospheric (TEA) pressure CO2 laser systems operating at 9300 nm with pulse durations of 10–20 μs suggested that high repetition rates are well suited for dental applications. Specifically, these systems were used for dentin and bone ablation in vitro (Fan et al. 2006).
In contrast, TEA 9600 nm CO2 lasers operating at the peak absorption wavelength of bone and with pulse durations of 5–8 μs showed approximately the same thermal relaxation rate as in hard tissue, produced high ablation rates, and minimal peripheral thermal damage. There was no discernible thermal damage and no need for water irrigation during ablation. In comparison, using a free‐running Er:YAG laser the peripheral thermal damage measured 25–40 μm (Fried and Fried 2001). However, none of these systems have wide applications in clinical practice.
4.3 Excimer Laser
Due to the effect that CO2 lasers are not routinely efficient without carbonization and thermal damage, high expectations were placed on the group of the excimer lasers (UV light). These lasers emit short pulses with durations in the nanosecond range in the wavelength range of 193–351 nm.
Comparative studies between the excimer laser (wavelength: 308 nm), the CO2 CW laser, and an oscillating saw on rabbit bones showed that the excimer laser caused only a small delay in healing compared to the saw (Nelson et al. 1989a, b; Yow et al. 1989). Also, the cutting quality on the bone and cartilage was significantly improved (Nelson et al. 1988, 1989a,b; Walsh and Deutsch 1989; Walsh et al. 1989; Gonzales et al. 1990; Meyer et al. 1990). The healing rate of an excimer laser cut was not slower than mechanical treatments, and the quality of the osteotomy was comparable to mechanical treatment (Dressel et al. 1991).
The ablation rates of excimer lasers in their present form are too low to meet clinical requirements. However, increasing the pulse repetition rate, there is risk of thermal damage stress of the bone (Doerschel 1995), but there are no clinical applications of these systems today.
4.4 Er:YAG and Ho:YAG Lasers
An in vivo animal model osteotomy with an Er:YAG laser (full width half maximum [FWHM] 180 μs, 5 Hz) showed after eight weeks complete bone fill of the osteotomy gap (Scholz 1992). It showed no crystallization products and a moderate brown color of the crater rim, although no spray‐cooling was used. The osseous structure and healing process were similar to conventional osteotomy methods and the application of the Er:YAG laser. Even after four weeks, the percentage of osseous construction in the osteotomy was more than 90%.
In contrast, the use of Ho:YAG laser (λ = 2.1 μm, FWHM 250 μs, repetition rate 5–10 Hz, pulse energy 400 mJ) showed a very strong destruction zone. When using a short‐pulsed CO2 laser (9.6 μm, ca. 65 μs FWHM and pulse energy 450 mJ, repetition rate 5 Hz) without spray‐cooling, an approximately 5 μm thick carbonization zone was produced, forming a healing barrier, which was not reduced even eight weeks after surgery (Table 4.1).
Since the absorption coefficient of bone at the wavelength of Ho:YAG lasers is low, high‐energy densities are necessary, but even then, a cut through the bone with this laser is very difficult. Moreover, complete bone healing was described only after a period of more than eight weeks (Nuss et al. 1988; Charlton et al. 1990).
However, recent studies were performed to explain the dynamic process of bubble evolution induced by Ho:YAG lasers underwater without and with bone tissue and its effects on hard tissue ablation. The results showed that the Ho:YAG laser was capable of ablating hard bone tissue effectively in under water irrigation conditions. The penetration of the Ho:YAG laser can be significantly increased up to about 4 mm with the assistance of the bubble. The hydrokinetic forces associated with the bubble not only contributed to reducing the thermal injury to peripheral tissues but also enhanced the ablation efficiency and improved the ablation crater morphology (Zhang et al. 2016).
Table 4.1 Bone healing in the osteotomies using different laser systems compared to conventional osteotomy. Quality assessment based on new osteon formation.
Bone Formation | After 4 Weeks | After 8 Weeks |
---|---|---|
Saw | +++ | +++ |
Excimer laser | ++ (+) | +++ |
Er:YAG laser | ++ (+) | +++ |
< ?sub Start?>Short pulsed CO2 laser< ?sub End?> | ++ | +++ |
< ?sub Start?>CW CO2 laser< ?sub End?> | + | + |
Ho:YAG laser | + | ++ |
Bone formation (optimal: +++) (according to Scholz 1992).
Results from studies in which ridge split was performed in pig mandible with Er:YAG laser, piezosurgery, and conventional drills showed that the maximum bone temperature of 7.3 °C was noted for a specimen prepared using piezosurgery devices. Furthermore, the bone temperature after irradiation with an Er:YAG laser with energy of 400 mJ increased more quickly (but less than the critical threshold) in comparison with the cases of energy equal to 200 mJ (Matys et al. 2016).
Besides, decortication for orthodontic implant placement with an Er:YAG laser compared to conventional osteotomy and piezosurgery methods was recently evaluated. The small diameter decortication by the Er:YAG laser appeared to provide better implant primary stability as compared to drill and piezosurgery (Matys et al. 2018).
4.5 Laser Systems for Clinical Dentistry
The currently published data and clinical practice have shown that bone removal and bone osteotomy are only possible with the Er:YAG or the Er,Cr:YSGG lasers. With the currently available applicators, the interventions on the bone, and with the necessary for the practice laser parameters, it is possible to use these laser wavelengths in clinical settings.
The use of special lenses (Zahn et al. 1997) or scanner systems for flat or geometry‐based ablation and the use of sapphire fibers and rods as applicator’s tail end allow osseous ablation with the Er:YAG laser. The quality of the osteotomy is comparable to the osteotomy performed by the Er,Cr:YSGG laser. Comparison with the conventional osteotomies using a Lindemann bur and piezosurgery showed a better precision in the osteotomy margins at the Er,Cr:YSGG and Er:YAG laser cuts according to Mueller and Romanos (2007) (Figure 4.1).
Besides, electron microscopic evaluation of the margins after Er:YAG or Er,Cr:YSGG laser showed a better cut quality using the Er,Cr:YSGG laser (Figures 4.2 and 4.3).
Sufficient cooling with an aerosol (Figure 4.4), preferably consisting of air and water (Ertl 1995), is essential for a good ablation of bone, as well as also every kind of treatment on biological hard tissues
Water amounts between 1 and 30 ml/min and air amounts between 1 and 10 l/min lead to good results. In this way, the risk of thermal damage can be reduced significantly, especially in the irradiation. Figures 4.2 and 4.3 show the detail of an osteotomy without melting, produced by an Er:YAG and Er,Cr:YSGG laser under spray cooling, respectively. Water spray also prevents the filling of the bone cut with blood, which would change the ablation with the laser, as the penetration depth is a few 10 μm, and therefore the treated osseous surface would be shielded.
Another way to reduce thermal damage on tissues during processing is to reduce the pulse length. However, even at pulse lengths of 50–40 μs, a spray cooling is unavoidable. However, a disadvantage of reducing the pulse length is the increase in pulse peak power. Especially, when using an optical fiber or a sapphire tip in the applicator, it may then lead to increased fiber burning at high energy densities.
In summary, the potential possibilities of bone removal using lasers today may occur in a thickness up to 3 mm. Advantages are the absence of contact with the tissue, the possible precision, and the lack of smear layer during bone cutting with bonemeal.
Clinical applications of bone removal in the oral cavity are:
- Removal of exostoses and alveolar ridge recontouring after multiple tooth extractions (Figures 4.4 and 4.5)
- Bone removal for root tip resection
- Crown lengthening procedures (Figure 4.6)
- Window preparation in sinus augmentations (Figure 4.7)
- Primary drill for implant placement (Figure 4.8)
- Implant removal (Figures 4.9 and 4.10)
- Bone splitting (Figure 4.11)

Figure 4.1 Comparison of the osteotomies performed by Lindemann bur, piezosurgery (Mectron), Er:YAG laser (KaVo) and Er,Cr:YSGG laser (Biolase, Inc.) illustrating an excellent quality of the osteotomies using the two laser systems.
Source: Dr. Georgios E Romanos.

Figure 4.2 Electron microscopic analysis of an osteotomy using Er:YAG laser (KaVo) and 300 mJ and 15 Hz.
Source: Dr. Georgios E Romanos.

Figure 4.3 Electron microscopic analysis of an osteotomy using Er,Cr:YSGG laser (Biolase) and 300 mJ and 15 Hz presenting an excellent osteotomy margin.
Source: Dr. Georgios E Romanos.

VIDEdental - Online dental courses
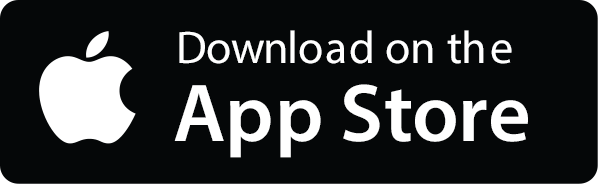
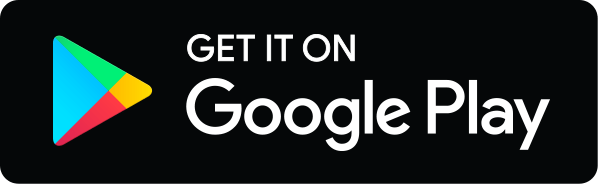