The structural and functional union of the implant with living bone is greatly influenced by the surface properties of the implant. The success of a dental implant depends on the chemical, physical, mechanical, and topographic characteristics of its surface. The influence of surface topography on osseointegration has translated to shorter healing times from implant placement to restoration. This article presents a discussion of surface characteristics and design of implants, which should allow the clinician to better understand osseointegration and information coming from implant manufacturers, allowing for better implant selection.
Key points
- •
The structural and functional union of the implant with living bone is greatly influenced by the surface properties of the implant.
- •
The success of a dental implant depends on the chemical, physical, mechanical, and topographic characteristics of its surface.
- •
The influence of surface topography on osseointegration has translated to shorter healing times from implant placement to restoration.
The fusion of the surface of a dental implant with the surrounding bone plays a crucial role in the longevity and the function of the implant-supported prosthesis. In fact, the most important biological event in the clinical healing phase of dental implants is cell adhesion at the interface between the host tissue and the implant. This structural and functional union of the implant with living bone is greatly influenced by the surface properties of the implant. In reality, the success of a dental implant is highly dependent on its chemical, physical, mechanical, and topographic characteristics of its surface. These different properties interact and determine the activity of the attached cells that are close to the dental implant surface. The topography of the implant surface has been shown to influence the differentiation and proliferation of osteoblasts, and the up-regulation of transcription factors that are responsible for the expression of bone matrix formation genes. The influence of surface topography on osseointegration has translated to shorter healing times from implant placement to restoration.
At present, the vast majority of dental implants marketed in the United States are made from commercially pure titanium (cpTi) or titanium alloys. A smaller group of implants are made out of a surface-coated hydroxyapatite (HA) or zirconium. Neither cpTi, its alloys, nor any of the other surface materials are fully 100% capable of directly bonding with living bone. Analysis of retrieved titanium implants showed that the bone-to-implant fusion was not entirely complete. Albrektsson and colleagues reported that the percentage of bone-to-implant contact area averaged 70% to 80%. However, even 60% bone-to-implant contact was enough osseointegration for successful implants that lasted for up to 17 years. Because of this recognized imperfection in total osseointegration, modifications of the implant surfaces are continuously being made for the purpose of enhancing and also shortening the time for osseointegration with each manufacturer claiming that theirs is the best.
There are several studies that compare one implant system with another, but a great number of them are comparisons with the Branemark system (Nobel Biocare, Zurich, Switzerland). There are no well-documented series of scientific studies that show the superiority of one implant surface over the other or one product versus another. Most of the better studies only focus on one implant system and documentation of their success. A good criterion for the quality of a dental implant is that studies should document that the implant survived for at least 5 years under function in the oral cavity. In this article, relevant scientific data are presented that should allow the clinician to better understand the information coming from implant manufacturers and that should allow for better implant selection.
Implant material
cpTi, titanium alloy (with or without HA), and zirconium are the materials of choice for dental implants. Implants made from other materials should not be used unless the manufacturer can produce sound scientific evidence with good clinical trials. Laboratory studies should not be accepted as validity for the quality of a dental implant because it is not able to predict actual patient outcome and clinical longevity.
Titanium
Titanium is biologically inert and has a high resistance to corrosion because of the spontaneous formation of a film of titanium oxide (TiO 2 ) on its surface, which separates the metal from its environment. Typically, the thickness of this oxide layer is about 3 to 10 nm. It is known that the protective and stable oxides on titanium surfaces are able to provide favorable osseointegration. The stability of the oxide depends strongly on the composition structure and thickness of the film. It is this oxide film that gives titanium and its alloys such good biocompatibility when used for dental implants. The passive film that forms on titanium is stable, even in a biological system as hostile as the oral cavity.
cpTi and titanium alloys are currently the materials of choice for dental implants. Unalloyed cpTi has various degrees of purity, which are graded from 1 to 4. The grading is related to the corrosion resistance, ductility, and strength. cpTi grade 1 has the highest purity, the highest corrosion resistance, formability, and lowest strength, whereas grade 4 offers the highest strength and moderate formability. Most dental implants are made from grade 4 cpTi because it is stronger than the other grades.
The titanium alloy used for dental implants is specially made for dental implant applications. The specific alloy used is grade 5 titanium alloy, which has greater yield strength and fatigue properties than pure titanium. Grade 5 alloy, which is usually designated as Ti6Al4V, has a chemical composition of 6% aluminum, 4% vanadium, 0.25% (maximum) iron, 0.2% (maximum) oxygen, and 90% titanium. The advantages of Ti6Al4V are its superior corrosion resistance, high fatigue strength, and low elastic modulus, which reduces stress shielding.
cpTi has limited mechanical properties. In cases where good mechanical characteristics are required, as in the posterior jaw where biting forces are high, Ti6Al4V alloy is preferred.
Hydroxyapatite
HA, the mineral component of bones and teeth, is used as a coating on the implant surface in an attempt to provide titanium and its alloys with improved bonding ability to living bone. Because bone contains HA, implants coated with this material are thought to be a good biomaterial for implant surface because of its excellent biocompatibility and good mechanical properties. HA coating has been shown to give improved bone implant attachment and, being osteoconductive in nature, more bone deposition has been noted. Enhancement of the osteoconductivity will increase the initial stability of the implant and shorten the treatment time.
Titanium under load-bearing conditions has good clinically biocompatibility coupled with high strength and fracture resistance but does not form a chemical bond with bone tissue. It is thought that HA coatings on titanium dental implants will accelerate surface bone apposition, thereby shortening the waiting period for dental implant restoration.
Studies have shown good primary stability of dental implants coated with HA with improved bone implant attachment. However, in a meta-analysis of HA-coated implants, the survival rates reported for HA-coated implants were similar to other implants, but the success rates were significantly lower than the overall survival rates. The 10-year survival rate was 87.39% compared with the 10-year success rate of 63.84%. These implants integrate fast and will survive but were only 64% clinically successful. The current HA applications of 1 μm thickness have now reduced the risk of loosening of the implant from the bone and have improved the success rate. Specific indications for the selection of HA-coated implants over metallic implants are reactions to metals, the need for a greater initial bone implant interface contact, placement in type IV bone, fresh extraction sites, and newly grafted sites.
Zirconium
Zirconia (Zr) is a “ceramic” compound, which is highly biocompatible and possesses the capacity to osseointegrate ( Fig. 1 ).
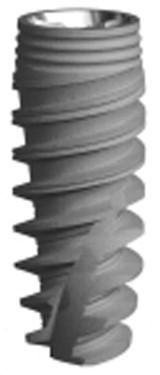
In its pure form, zirconium cannot be used in dentistry. It has to be manufactured, following many different steps, to be usable in dentistry. The manufacturing process of zirconium implants is very strict but varies for each implant company. The companies have not provided extensive information about the surface characteristics of their implants; this represents some difficulty in the evaluation of its surface because the final product may have different chemical compositions. Regardless of the composition, however, it has been shown that the biocompatibility of zirconium is good, even if the composition of the tested materials is different.
As a biomaterial for dental implants, zirconium possesses very favorable physical properties, such as flexural strength (900–1200 MPa), hardness (1200 Vickers), as well as a favorable threshold stress intensity factor. Another quality is its resistance to corrosion. Although highly unlikely, titanium can be subjected to corrosion, thus making the longevity and durability theoretically less than zirconium. The corrosion possibilities of titanium are almost entirely dependent on the iron content. At room temperature, titanium normally forms a stable oxide coating on its surface, which limits further oxidation. This oxide coating is very adherent, and chemically stable, and is capable of spontaneously and instantaneously repairing itself if damaged by its environment. cpTi grade 4, the strongest of the grades, has the highest allowable iron content of the unalloyed grades of 0.50% maximum iron. Despite its relatively high iron content, the corrosion resistance of cpTi grade 4 is very high based on the presence of the stable, continuous, tightly adherent oxide layer, which forms spontaneously on exposure to oxygen.
Animal studies indicate similar biocompatibility and osseointegration for zirconium compared with titanium implants. In a recent extensive review article, the literature suggested that zirconium implants had an inferior degree of osseointegration (removal torque tests) compared with titanium implants, but that some surface structural modifications allowed removal torque test values to come close to those obtained by titanium implants. The removal torque test values will depend more on the surface structure than on the implant material itself. To date, there does not appear to be consistency in the zirconium materials used and in their long-term stability. Its major indications are a replacement for metals and improved esthetic qualities in anterior restorations. Wennerberg and Albrektsson recommended proceeding slowly and with caution when considering Zr ceramic implants.
Implant material
cpTi, titanium alloy (with or without HA), and zirconium are the materials of choice for dental implants. Implants made from other materials should not be used unless the manufacturer can produce sound scientific evidence with good clinical trials. Laboratory studies should not be accepted as validity for the quality of a dental implant because it is not able to predict actual patient outcome and clinical longevity.
Titanium
Titanium is biologically inert and has a high resistance to corrosion because of the spontaneous formation of a film of titanium oxide (TiO 2 ) on its surface, which separates the metal from its environment. Typically, the thickness of this oxide layer is about 3 to 10 nm. It is known that the protective and stable oxides on titanium surfaces are able to provide favorable osseointegration. The stability of the oxide depends strongly on the composition structure and thickness of the film. It is this oxide film that gives titanium and its alloys such good biocompatibility when used for dental implants. The passive film that forms on titanium is stable, even in a biological system as hostile as the oral cavity.
cpTi and titanium alloys are currently the materials of choice for dental implants. Unalloyed cpTi has various degrees of purity, which are graded from 1 to 4. The grading is related to the corrosion resistance, ductility, and strength. cpTi grade 1 has the highest purity, the highest corrosion resistance, formability, and lowest strength, whereas grade 4 offers the highest strength and moderate formability. Most dental implants are made from grade 4 cpTi because it is stronger than the other grades.
The titanium alloy used for dental implants is specially made for dental implant applications. The specific alloy used is grade 5 titanium alloy, which has greater yield strength and fatigue properties than pure titanium. Grade 5 alloy, which is usually designated as Ti6Al4V, has a chemical composition of 6% aluminum, 4% vanadium, 0.25% (maximum) iron, 0.2% (maximum) oxygen, and 90% titanium. The advantages of Ti6Al4V are its superior corrosion resistance, high fatigue strength, and low elastic modulus, which reduces stress shielding.
cpTi has limited mechanical properties. In cases where good mechanical characteristics are required, as in the posterior jaw where biting forces are high, Ti6Al4V alloy is preferred.
Hydroxyapatite
HA, the mineral component of bones and teeth, is used as a coating on the implant surface in an attempt to provide titanium and its alloys with improved bonding ability to living bone. Because bone contains HA, implants coated with this material are thought to be a good biomaterial for implant surface because of its excellent biocompatibility and good mechanical properties. HA coating has been shown to give improved bone implant attachment and, being osteoconductive in nature, more bone deposition has been noted. Enhancement of the osteoconductivity will increase the initial stability of the implant and shorten the treatment time.
Titanium under load-bearing conditions has good clinically biocompatibility coupled with high strength and fracture resistance but does not form a chemical bond with bone tissue. It is thought that HA coatings on titanium dental implants will accelerate surface bone apposition, thereby shortening the waiting period for dental implant restoration.
Studies have shown good primary stability of dental implants coated with HA with improved bone implant attachment. However, in a meta-analysis of HA-coated implants, the survival rates reported for HA-coated implants were similar to other implants, but the success rates were significantly lower than the overall survival rates. The 10-year survival rate was 87.39% compared with the 10-year success rate of 63.84%. These implants integrate fast and will survive but were only 64% clinically successful. The current HA applications of 1 μm thickness have now reduced the risk of loosening of the implant from the bone and have improved the success rate. Specific indications for the selection of HA-coated implants over metallic implants are reactions to metals, the need for a greater initial bone implant interface contact, placement in type IV bone, fresh extraction sites, and newly grafted sites.
Zirconium
Zirconia (Zr) is a “ceramic” compound, which is highly biocompatible and possesses the capacity to osseointegrate ( Fig. 1 ).
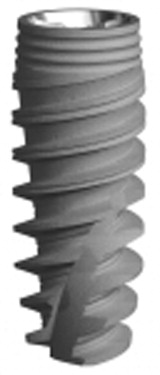
In its pure form, zirconium cannot be used in dentistry. It has to be manufactured, following many different steps, to be usable in dentistry. The manufacturing process of zirconium implants is very strict but varies for each implant company. The companies have not provided extensive information about the surface characteristics of their implants; this represents some difficulty in the evaluation of its surface because the final product may have different chemical compositions. Regardless of the composition, however, it has been shown that the biocompatibility of zirconium is good, even if the composition of the tested materials is different.
As a biomaterial for dental implants, zirconium possesses very favorable physical properties, such as flexural strength (900–1200 MPa), hardness (1200 Vickers), as well as a favorable threshold stress intensity factor. Another quality is its resistance to corrosion. Although highly unlikely, titanium can be subjected to corrosion, thus making the longevity and durability theoretically less than zirconium. The corrosion possibilities of titanium are almost entirely dependent on the iron content. At room temperature, titanium normally forms a stable oxide coating on its surface, which limits further oxidation. This oxide coating is very adherent, and chemically stable, and is capable of spontaneously and instantaneously repairing itself if damaged by its environment. cpTi grade 4, the strongest of the grades, has the highest allowable iron content of the unalloyed grades of 0.50% maximum iron. Despite its relatively high iron content, the corrosion resistance of cpTi grade 4 is very high based on the presence of the stable, continuous, tightly adherent oxide layer, which forms spontaneously on exposure to oxygen.
Animal studies indicate similar biocompatibility and osseointegration for zirconium compared with titanium implants. In a recent extensive review article, the literature suggested that zirconium implants had an inferior degree of osseointegration (removal torque tests) compared with titanium implants, but that some surface structural modifications allowed removal torque test values to come close to those obtained by titanium implants. The removal torque test values will depend more on the surface structure than on the implant material itself. To date, there does not appear to be consistency in the zirconium materials used and in their long-term stability. Its major indications are a replacement for metals and improved esthetic qualities in anterior restorations. Wennerberg and Albrektsson recommended proceeding slowly and with caution when considering Zr ceramic implants.
Surface characteristics
To improve the rate and quality of osseointegration, survivability, and function, manufacturers have focused on topographic changes of implant surfaces rather than alterations of chemical properties. One such significant alteration that affects the mechanical stability of the implant/tissue interface is the creation of surface irregularities that improve the mechanical interlocking between bone tissue and implant materials. Irregular surfaces improve metal shear strength and decrease implant loosening. In addition to providing better mechanical stability between bone tissue and the implant surface, roughness is also a configuration that will retain blood clots completely and stimulates the bone-healing process. Clinically, it has been known for a long time that the amount of bone-to-implant contact is an important factor in the long-term success of dental implants. This bone-to-implant contact is enhanced by implant surface roughness. Controlled surface roughness now plays an important role in enhancing the osseointegration of titanium implants.
Biochemical methods of surface modifications, for example, adding rhBMP-2 or fluoride, have been tried but have had a limited effect on the level of osseointegration. The general goals of biochemical modifications are to immobilize proteins, enzymes, or peptides on biomaterials for the purpose of inducing specific cell and tissue responses, or in other words, to control the tissue-implant interface with molecules that are delivered directly to the interface.
The rate and quality of osseointegration of dental implants are highly related to their composition and surface roughness. Rough-surfaced implants improve bone anchoring and biomechanical stability, and several reports have documented that the surface roughness of titanium and other implants affect the rate of osseointegration and the biomechanical fixation. High roughness results in mechanical interlocking between the implant surface and the adjacent bone along with stimulation of the bone-healing process. Because of this, it has been suggested that these types of implants can be loaded earlier than machined implants. A surface roughness in the range of 1 to 10 μm will maximize the interlocking between mineralized bone and the surface of the implant. Surface roughness in this range will result in greater bone-to-implant contact and higher resistance to torque removal. A theoretic approach suggested that the ideal surface should be covered with hemispherical pits approximately 1.5 μm in depth and 4 μm in diameter. However, there is currently no consensus on the degree of surface roughness that is optimum for bone cell attachment.
Implant surface irregularities can be achieved by surface concave texturing or by surface convex texturing. Surface concave textures are achieved by either etching the material by chemical or electrochemical actions or creating mechanical indentations by sand-blasting, shot-peening, or laser-peening. Convex textured surface is formed by depositing certain types of particles on the implant surface, for example, plasma-spraying ( Fig. 2 ).
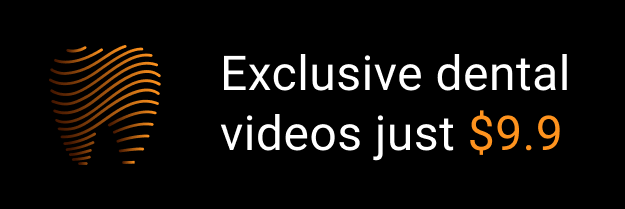