Introduction
Our goal was to verify the association between candidate polymorphisms and skeletal Class III malocclusion in a well-characterized homogeneous sample set.
Methods
Thirty-five single-nucleotide polymorphisms were studied from 10 candidate loci in 54 Class III subjects and 120 controls. Skeletal Class III characteristics included ANB angle less than 0°, SNB angle greater than 83° (mandibular prognathism), SNA angle less than 79° (maxillary deficiency), Class III molar relationship, and negative overjet. Inclusion criteria for the controls were ANB angle between 0° and 4°, Class I molar relationship, and normal overjet. Chi-square and Fisher exact tests and principal component (PC) analysis were used to determine overrepresentation of marker alleles with alpha of 0.05. Odds ratios and 95% confidence intervals were calculated.
Results
MYO1H (rs10850110 A<G) ( P <0.01; odds ratio, 7.44 [4.02-13.77]) was associated with an increased risk for the mandibular prognathism phenotype. These results were confirmed by PC analysis, which showed 4 PCs representing the sample variations (PC1, 37.24%; PC2, 20.02%; PC3, 12.18%; and PC4, 11.40%), and PC1 was associated with MYO1H ( P <0.001). We also found by PC analysis associations between MYO1H ( P <0.001) and GHR (rs2973015 A>G) ( P = 0.001) with PC2 and between FGF10 (rs593307 A<G) ( P = 0.001) with PC4.
Conclusions
Polymorphism in MYO1H could be used as a marker for genetic susceptibility to Class III malocclusion with mandibular prognathism, and polymorphisms in GHR and FGF were associated with maxillomandibular discrepancies. This study may contribute to improved diagnosis and further research assessing possible differences in treatment responses based on genetic polymorphisms.
Highlights
- •
Mandibular prognathism is the principal and most frequent component of skeletal Class III malocclusion.
- •
Class III prognathism is associated with MYO1H.
- •
Genes such MYO1H , GHR , and FGF10 also appear to contribute to maxillomandibular discrepancies.
The skeletal Class III malocclusion phenotype is heterogeneous and is usually characterized by some combination of excessive mandibular growth (mandibular prognathism, Mendelian Inheritance in Man [MIM] #176700) and deficient maxillary growth and can occur as part of a syndrome or as an isolated trait. The clinical aspects of Class III malocclusion can be perceived in childhood and become progressively more evident with growth, contributing to disturbances in both function and esthetics. Prevalence varies according to different populations; it is higher in Asians (19%) and lower in white people (1.0%). Its etiology is still unknown and has been attributed to many patterns of genetic inheritance and to environmental factors as well as to gene-environment interactions.
Several candidate loci have been related to skeletal Class III malocclusion. According to the first genome-wide linkage analysis, there were mandibular prognathism susceptibility loci in chromosomes 1p36, 6q25, and 19p13.2 in Korean and Japanese families. In Hispanic families, genome-wide linkage showed 5 suggestive loci to maxillary deficiency (1p22.1, 3p26.2, 11q22, 12q13.3, and 12q23). Suggestive evidence of linkage in a Han Chinese pedigree was shown on the 14q24.3-31.2 locus for mandibular prognathism. Based on the findings of genome-wide linkage studies, Tassopoulou-Fishell et al and Fontoura et al showed evidence that a polymorphism in Myosin 1H ( MYO1H ) was associated with mandibular prognathism. Furthermore, a genome-wide association study showed 2 loci (1p32.2 and 1p22.3) susceptible to mandibular prognathism in Japanese people. According to Nikopensius et al, the region 12q22-q23—harboring dual specificity phosphatase 6 ( DUSP6 )—may be genetically linked to both mandibular prognathism and maxillary deficiency. Growth hormone receptor ( GHR ) appears to play an important role in the mandibular growth in Japanese, Chinese, Korean, and Turkish peoples.
Furthermore, additional candidate genes can be proposed based on gene function and its potential impact on normal and abnormal postnatal growth. Fibroblast growth factors ( FGFs ) control the balance among skeletal cell growth, differentiation, and apoptosis. FGFs and fibroblast growth factor receptors ( FGFRs ) are essential for the control of endochondral and intramembranous bone formation during development. A mutation in FGFR1 at 8p11.2 is responsible for Pfeiffer syndrome (MIM #101600), which includes craniosynostosis as a phenotype. Moreover, mutations in FGFR2 at 10q26 cause Crouzon (MIM #123500) and Apert (MIM #101200) syndromes, and both present a Class III malocclusion phenotype. Our previous work showed that genes that are linked to rare syndromes can give insight for the comprehension of isolated traits, and FGFs and FGFRs may be involved in isolated forms of skeletal Class III malocclusion.
There is a consensus of a need to replicate previous genetic association findings because of the winner’s curse effect, and our initial report of an association between MYO1H and mandibular prognathism should be replicated. Hence, we aimed to verify the association between polymorphisms in loci identified in previous studies (1p22.1, 1p22.2, 1p36, 3q26.2, 5p13-p12, 6q26, 11q22.2-q22.3, 12q23, 12q13.13, and 19p13.2), and polymorphisms in several FGF family members for skeletal Class III malocclusion.
Material and methods
This study was approved by the research and ethics committee of Antônio Pedro University Hospital at the Universidade Federal Fluminense (number 314/2011) in Rio de Janeiro, Brazil, and by the University of Pittsburgh Institutional Review Board in Pittsburgh, Pa. Written consent was obtained from all adults or legal guardians, in the case of minors, before they entered this study.
The cohort of 652 subjects in active treatment from 3 reference centers for malocclusion treatment in Rio de Janeiro, Brazil (Departments of Pediatric Dentistry and Orthodontics at the Universidade Federal do Rio de Janeiro, Universidade Federal Fluminense, and Brazilian Dental Association) formed the initial sample. Patients were recruited for this study from January 2011 to January 2013.
Each subject’s clinical aspects, pretreatment lateral cephalometric records, and cast records were assessed for eligibility. Two groups were selected from these patients based on preestablished characteristics: a Class III malocclusion group and a control group (Class I patients). The inclusion criteria for the Class III malocclusion group were cephalometric ANB angle (Point A-nasion-Point B) of centric jaw relationship less than 0.0°, Class III molar malocclusion according to Angle’s classification, negative overjet, and age over 6 years. To increase homogeneity, the maxillary deficiency and the mandibular prognathism were distinguished within skeletal Class III malocclusion. The cephalometric parameters used to classify the maxillary deficiency were SNA angle (sella-nasion-Point A) less than 79°, and the mandibular prognathism was SNB angle (sella-nasion-Point B) greater than 83°. Inclusion criteria for the control group were skeletal Class I (ANB angle between 0° and 4°), Class I molar occlusion according to Angle’s classification, normal overjet, and age over 6 years. Exclusion criteria for both groups comprised growth disturbances, syndromes, cleft lip and palate, missing teeth, poor quality of radiographic records, consent form not signed, and trauma. The lateral cephalograms of all patients who had been previously classified in their records as having a Class III or Class I phenotype were retraced by the same examiner (C.V.C.) to check eligibility for the study. Reliability was determined by measurement of 20 cephalograms randomly selected from the sample. The intraexaminer agreement was assessed by a second cephalometric measurement after 2 weeks. An additional cephalometric parameter recorded was the sella-nasion–gonion-gnathion angle (SN-GoGn) of each subject to register the main facial growth direction. Thus, a total of 14 cephalometric measurements were assessed to find the most significant components of variation representing a distinct Class III phenotype to reduce the genetic heterogeneity (SNA angle, maxillary unit length, anterior cranial base, SND angle, SNB angle, ANB angle, facial convexity, 3 measurements of the length of mandibular base, facial depth, maxillary depth, and facial axis).
After the inclusion and exclusion criteria were applied, 185 unrelated patients remained in the sample. There were missing data for 11 patients: 7 subjects moved to another city, 3 subjects dropped out of the study, and 1 subject died. The final sample comprised 174 subjects. Fifty-four subjects (34 white, 20 black; 27 male, 27 female; mean age, 19.65 ± 8.7 years) were included in the Class III malocclusion group. In this group, 31 subjects had mandibular prognathism, and 25 had maxillary deficiency. One hundred twenty subjects (82 white, 38 black; 53 male, 67 female; mean age, 20.46 ± 11.15 years) were included in the control group. Assuming D’ = 1.0, a frequency of 20% of the high-risk marker allele, and genotypic relative risk of 1 copy of the high-risk allele of 2.0 and 2 copies of 3.0, our power calculations suggested that we would have 85% power to detect an association with an alpha of 0.05.
Saliva was collected from all participants (they were asked to spit), and the genomic DNA was extracted according to published protocols. All saliva samples were numbered with the patient’s name hidden, and all analyses were performed blindly to the case-control status.
Thirty-five single-nucleotide polymorphism (SNP) markers were selected in candidate genes to mandibular prognathism from previous studies and in genes related to the skeletal Class III phenotype ( Table I ). These markers were chosen based on information on the gene structure and linkage disequilibrium relationships available at the international HapMap Project Web site ( http://www.hapmap.org/ ). Real-time polymerase chain reaction was performed using the TaqMan method; for all TaqMan assays, an end-point analysis was performed in an automatic sequence-detection instrument (ABI Prism 7900HT; Applied Biosystems, Foster City, Calif) to test for the presence of an allelic variant in the genes, and the results were recorded according to fluorescent signals from reporters VIC and FAM. Each reaction mixture contained 10 μL 1x TaqMan universal polymerase chain reaction master mix, 0.5 μL 1x Taq-Man SNP kit (probe/primer mix), 2 μL DNA obtained, and 7.5 μL DNase-free water in a final volume of 20 μL. Standard amplification conditions were 95°C for 10 minutes, and 40 cycles at 92°C for 15 seconds and at 60°C for 40 seconds; 2 negative controls with sterile water as the template were used in each reaction plate. For quality control for genotyping, 10% of the sample was genotyped, with greater than 99% concordance.
Locus | Gene | Marker public identification | Base pair change |
---|---|---|---|
1p36.11 | Intergenic | rs4649030 | A/G |
3q26.32 | Intergenic | rs2087312 | G/T |
Intergenic | rs987526 | A/G | |
5p12 | Growth hormone receptor ( GHR ) | rs2973015 | A/G |
Growth hormone receptor ( GHR ) | rs1509460 | A/C | |
Growth hormone receptor ( GHR ) | rs2910875 | C/T | |
5p13-12 | Fibroblast growth factor 10 ( FGF10 ) | rs11750845 | C/T |
Fibroblast growth factor 10 ( FGF10 ) | rs1448037 | A/G | |
Fibroblast growth factor 10 ( FGF10 ) | rs900379 | C/T | |
Fibroblast growth factor 10 ( FGF10 ) | rs1011814 | A/G | |
Fibroblast growth factor 10 ( FGF10 ) | rs593307 | C/T | |
Fibroblast growth factor 10 ( FGF10 ) | rs7708529 | C/T | |
8p12.11.2 | Fibroblast growth factor receptor 1 ( FGFR1 ) | rs13317 | C/T |
6q26 | Parkinson juvenile disease protein 2 ( PARK2 ) | rs7750085 | A/T |
Parkinson juvenile disease protein 2 ( PARK2 ) | rs12207168 | A/G | |
Parkinson juvenile disease protein 2 ( PARK2 ) | rs1884153 | C/T | |
10q26 | Fibroblast growth factor receptor 2 ( FGFR2 ) | rs2981582 | C/T |
11q13 | Fibroblast growth factor 3 ( FGF3 ) | rs7932320 | A/G |
Fibroblast growth factor 3 ( FGF3 ) | rs1893047 | A/G | |
Fibroblast growth factor 3 ( FGF3 ) | rs12574452 | A/G | |
Fibroblast growth factor 3 ( FGF3 ) | rs10796856 | C/T | |
Fibroblast growth factor 3 ( FGF3 ) | rs4980700 | A/G | |
Fibroblast growth factor 3 ( FGF3 ) | rs35420992 | C/T | |
11q13.3 | Intergenic | rs4631909 | C/T |
11q22.3 | Caspase 4 isoform gamma precursor ( CASP4 ) | rs571407 | A/G |
12q13.13 | Keratin 7 ( KRT7 ) | rs1902768 | C/T |
Keratin 7 ( KRT7 ) | rs7300317 | A/G | |
12q23.3 | Intergenic | rs11113231 | A/G |
12q24.11 | Myosin 1H ( MYO1H ) | rs10850110 | A/G |
15q21.2 | Fibroblast growth factor 7 ( FGF7 ) | rs2413958 | C/T |
19p13.2 | Fibrilin 3 precursor ( FBN3 ) | rs7351083 | A/G |
Fibrilin 3 precursor ( FBN3 ) | rs4804264 | C/T | |
Fibrilin 3 precursor ( FBN3 ) | rs8103218 | C/T | |
Fibrilin 3 precursor ( FBN3 ) | rs12327845 | C/T | |
19p13.3 | Intergenic | rs10411185 | A/G |
Statistical analysis
Reliability was calculated using the intraclass correlation coefficient and confirmed by a median value of 0.982.
The chi-square, Fisher exact, and independent t tests were carried out to compare sex and ethnicity frequencies and to assess deviations in the allele and genotype distributions between both skeletal Class III and Class I subjects. The variations of SNA, SNB, and ANB angles of the Class III subjects and controls were tested separately according to sex, ethnicity (independent t test), and age (correlation coefficient). Odds ratios were used to measure the strength of the association between the frequencies of genotype in the Class III malocclusion and Class I participants. All P values were 2-tailed, and 95% confidence intervals (95% CI) were calculated. After the Bonferroni correction (0.05/35), the established alpha was 0.0014286, to accommodate for the concern of multiple tests. Hardy-Weinberg equilibrium was tested by a goodness-of-fit test, with 1 degree of freedom ( http://www.oege.org/software/hardy-weinberg.html ), comparing observed genotype frequencies with expected genotype frequencies among subjects. A P value less than 0.05 was considered to be significant, and only the results that were in Hardy-Weinberg equilibrium were further analyzed. Furthermore, principal components explaining more than 5% of the facial skeletal variation were selected for genotype-phenotype correlation analyses. Data were normalized and standardized using a linear model to assess the possible effects of age and sex and to consider the possibility of age-by-sex interactions. SNPs were coded 0, 1, and 2 according to the number of minor allele copies. Multivariate linear regressions adjusting for age, sex, and ethnicity were performed to test for associations between each SNP (one at a time) and the selected principal components. The same Bonferroni threshold described above was used here. All analyses were performed with SPSS software for Windows (version 20.0; IBM, Armonk, NY).
Statistical analysis
Reliability was calculated using the intraclass correlation coefficient and confirmed by a median value of 0.982.
The chi-square, Fisher exact, and independent t tests were carried out to compare sex and ethnicity frequencies and to assess deviations in the allele and genotype distributions between both skeletal Class III and Class I subjects. The variations of SNA, SNB, and ANB angles of the Class III subjects and controls were tested separately according to sex, ethnicity (independent t test), and age (correlation coefficient). Odds ratios were used to measure the strength of the association between the frequencies of genotype in the Class III malocclusion and Class I participants. All P values were 2-tailed, and 95% confidence intervals (95% CI) were calculated. After the Bonferroni correction (0.05/35), the established alpha was 0.0014286, to accommodate for the concern of multiple tests. Hardy-Weinberg equilibrium was tested by a goodness-of-fit test, with 1 degree of freedom ( http://www.oege.org/software/hardy-weinberg.html ), comparing observed genotype frequencies with expected genotype frequencies among subjects. A P value less than 0.05 was considered to be significant, and only the results that were in Hardy-Weinberg equilibrium were further analyzed. Furthermore, principal components explaining more than 5% of the facial skeletal variation were selected for genotype-phenotype correlation analyses. Data were normalized and standardized using a linear model to assess the possible effects of age and sex and to consider the possibility of age-by-sex interactions. SNPs were coded 0, 1, and 2 according to the number of minor allele copies. Multivariate linear regressions adjusting for age, sex, and ethnicity were performed to test for associations between each SNP (one at a time) and the selected principal components. The same Bonferroni threshold described above was used here. All analyses were performed with SPSS software for Windows (version 20.0; IBM, Armonk, NY).
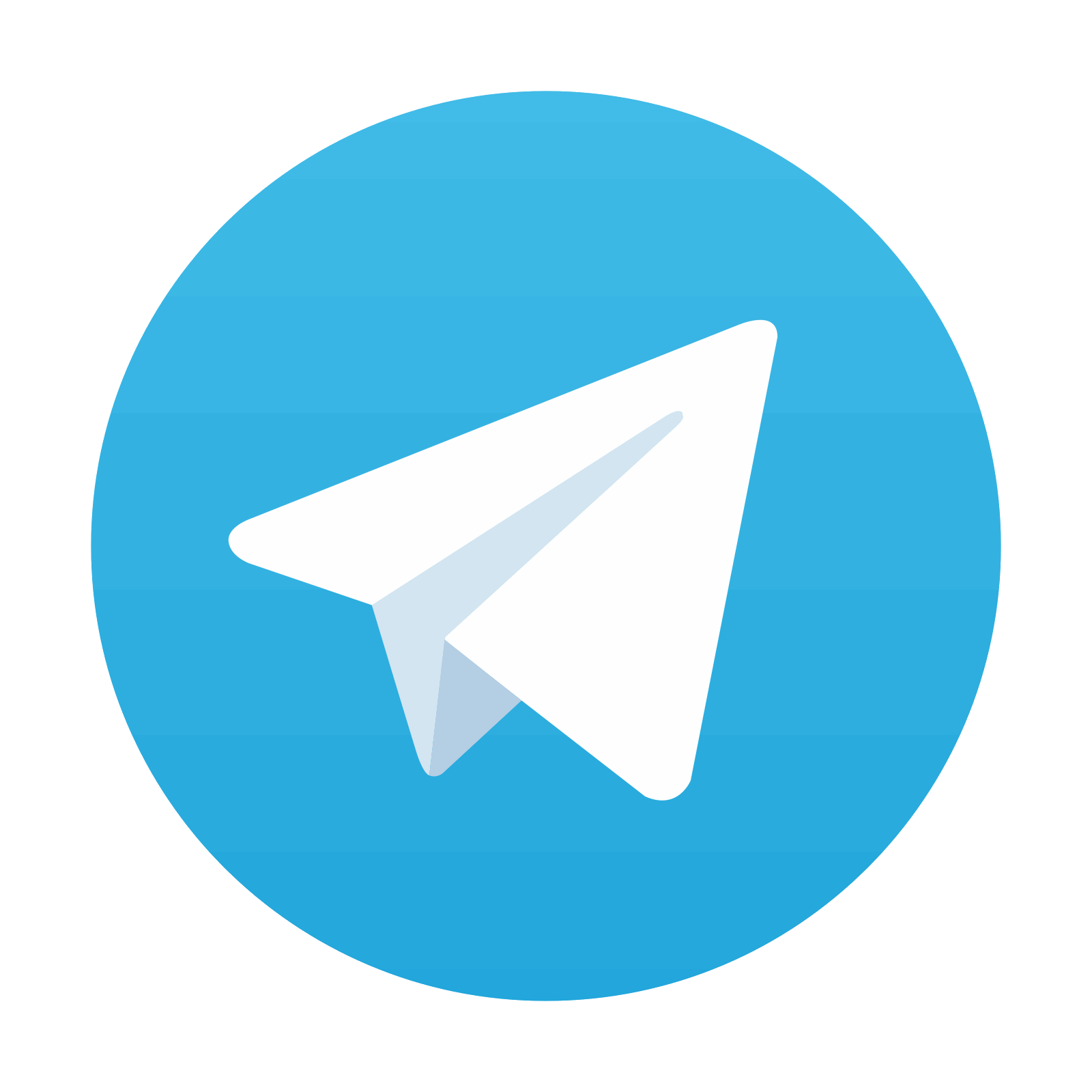
Stay updated, free dental videos. Join our Telegram channel

VIDEdental - Online dental courses
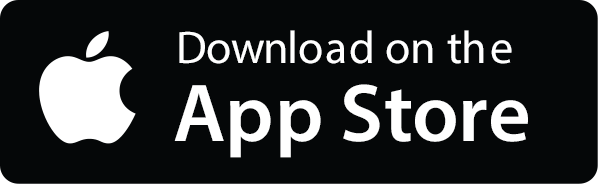
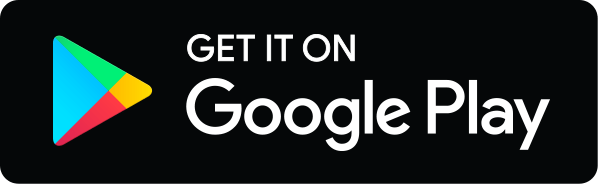