Abstract
Streak artefacts caused by dental metals deteriorate the quality of computed tomography (CT) images. We developed and evaluated a method for generating three-dimensional virtual models to plan orthognathic surgery in patients with multiple dental materials, to avoid the adverse effects of metal artefacts in image fusion. The method basically consists of four procedures: (1) fabrication of a splint in the open-mouth position with fiducial markers, (2) reconstruction of a virtual skull model in the open-mouth position from CT scanning, (3) reconstruction of two virtual dental models in the open-mouth position and either the intercuspal position (ICP) or centric relation (CR) from surface scanning, and (4) three serial steps of image registration and subsequent repositioning of the mandible to the ICP or CR. This method allows for the registration of skull and dental models under artefact-free conditions. To validate the method, CT and dental cast data from 30 patients were used. The registration accuracy was 0.080 mm for the initial registration, 0.033 mm for the second registration, and 0.028 mm for the third registration. The present method can be used to determine the occlusal relationships and craniofacial morphology of patients with dental metals and can be applied to computer-assisted diagnosis and surgery.
Recent rapidly developing computer technology has provided us with a new powerful tool for diagnosing facial and jaw deformities (computer-assisted diagnosis), and for planning and guiding surgical interventions (computer-assisted surgery). Although computed tomography (CT), from which virtual skull models with dentition are generated, is a fundamental procedure in computer-assisted diagnosis and surgery, it has several disadvantages: (1) limited spatial resolution and partial volume-averaging effects of CT cause image distortion, especially when small anatomical details such as the occlusal surface of teeth are scanned; (2) the threshold condition influences the morphology of the teeth and cranial bones; and (3) information about the occlusal surface is lost if the CT scan is performed with the teeth in occlusion. Therefore, the image quality obtained from CT scanning only is not sufficient to precisely represent tooth configuration and intercuspation, which is a definite disadvantage for predicting jaw position after orthognathic surgery. To overcome these problems, an image-fusion technique, in which a virtual skull model obtained from CT scanning and a virtual dental model obtained from laser scanning of a dental cast or CT scanning of an impression, was introduced into three-dimensional (3D) reconstruction methods to diagnose skeletal deformities.
In addition to the above-mentioned disadvantages of CT scanning, another significant problem is streak artefacts caused by strongly attenuating objects, i.e., metal artefacts. The presence of metal restorations, prostheses, dental implants, and orthodontic appliances in the field of view of the CT scan causes the metal artefacts, which may drastically degrade the CT images and limit the application of CT. This problem cannot be resolved entirely, even using the image-fusion technique, if the multiple and extensive artefacts extend into the fiducial markers used for image integration, thereby disturbing precise image fusion.
The aim of this study was to develop a method for generating virtual models that can realize the tooth configuration, occlusal relationships, and craniofacial morphology of patients with multiple metallic dental materials, to avoid the adverse effects of metal artefacts in the image-fusion process. In doing so, the accuracy of the generated virtual patient models may be improved and their application extended to a wide range of patients who need 3D diagnoses and surgical simulation.
Materials and methods
Thirty patients (10 males, 20 females) who were diagnosed with severe skeletal deformities and required orthognathic surgery were examined. The patients ranged in age from 15.3 years to 34.4 years, with a mean age of 26.4 years. All patients were treated in the university maxillofacial deformities clinic. Inclusion criteria were as follows: (1) patients with complicated 3D jaw deformities involving skeletal asymmetry, who were expected to need complicated movements of the segmented bones, including multidirectional translation and/or rotation with more than two rotational axes, and (2) patients with multiple dental metals, such as orthodontic appliances, restorations, and prostheses. Materials including CT data and dental cast models were obtained at the initial examination or just before orthognathic surgery. Ethical approval was granted, and all patients gave their informed consent to participate. When the patients were under 20 years of age, further informed consent was obtained from their parents.
The system, which consisted of an X-ray CT apparatus, non-contact surface scanner, and their related software, was used to generate a virtual patient model (fusion model of a virtual skull and dentition in the intercuspal position (ICP) or centric relation (CR)). Details of the generation method have been described previously. In a previous study, dental casts occluded in the ICP or CR were used as a starting model. In this study, however, dental casts in the open-mouth position and either ICP or CR were used ( Fig. 1 ). Our method consisted of the following five procedures: (1) reconstruction of a virtual skull model from CT scanning in the open-mouth position pre-surgery (VS-O), (2) reconstruction of two virtual dental models from the 3D surface scanning of dental casts in the open-mouth position and ICP or CR (VD-O and VD-C), (3) reconstruction of a preliminary fusion model of VS-O and VD-O by an initial intermodality registration, (4) reconstruction of another preliminary fusion model of VS-O, VD-O, and VD-C by a second intramodality registration, and (5) repositioning of the mandible by a third intramodality registration and reconstruction of the virtual patient model.

Step 1: Fabrication of a reference splint for fusing virtual skull and dentition models
To construct a reference structure to fuse a virtual skull with the dentition, a horseshoe-shaped reference splint was made of self-curing resin (Shofu Inc., Tokyo, Japan) ( Fig. 2 ). Ceramic balls 9/32-in. in diameter (Amatsuji Co. Ltd, Osaka, Japan) were attached to the buccal surface of the reference splint as fiducial markers. To avoid extending of the metal artefacts into the fiducial markers, an open-mouth position of one to two finger-breadths was registered at the splint, while the horizontal jaw position was arbitrarily determined. Each fiducial marker was positioned at the midpoint of vertical clearance between the maxillary and mandibular dentitions.

Step 2: Reconstruction of a virtual skull model
CT data of the craniofacial skeleton were acquired using a helical scanner (ProSpeed FII; GE Medical Systems, Milwaukee, WI, USA). The patient underwent CT scanning with a 16-bit 512 × 512 matrix at a slice thickness of 1.0 mm, slice pitch of 1.0 mm, reconstruction pitch of 0.5 mm, and field of view of 256 mm. The patient lay in the supine position with the reference splint in place during the CT scan. The patient’s head was positioned with the plane containing the three fiducial markers perpendicular to the horizontal plane as close as possible. This head position ensured that the fiducial markers were located outside of expected artefacts on a scout view, which allowed easy and precise extraction of the markers.
The digital two-dimensional (2D) CT data were transferred directly to a PC and stored in DICOM format. The DICOM data were transferred to a workstation (xw9300; Hewlett-Packard Co., Palo Alto, CA, USA) and reconstructed three-dimensionally using 3D medical imaging software (V-works 4.0; Cybermed Inc., Seoul, Korea). The bone areas, together with the teeth and fiducial markers, were segmented from a given multi-planar reconstruction (MPR) image using a threshold value ranging from 320 to 3071, polygonized, and outputted as VRML files. The VRML data were then imported into 3D reverse engineering software (Imageware 10.6, UGS PLM Solutions, Plano, TX, USA) ( Fig. 3 a and b ). The artefactual structures, dentition, and alveolar bone were selected manually and eliminated using the ‘Trim Tool’ in Imageware, leaving the fiducial markers of the splint, which were indicated in yellow on the monitor ( Fig. 3 c and d).

Step 3: Reconstruction of virtual dentition models
To make dental cast models, alginate impressions (Starmix; Morita Corp., Tokyo, Japan) were taken with the stabilizing archwires removed and filled with a die stone (Noritake Stone Ex Ortho-White; Kuraray-Noritake Dental Inc., Tainai, Japan). The bracket portion of the dental cast model was trimmed so that undercuts could be minimized during the subsequent 3D surface scanning. Then three ceramic balls were attached to the maxillary and mandibular cast bases as fiducial markers to determine changes in the positions of the maxillary and mandibular dentition during image fusion ( Fig. 4 a–d ).

A 3D surface scanning system using a slit laser beam (VIVID910; Konika-Minolta, Tokyo, Japan) was used to acquire 3D data from the dental cast model, as described previously. Its accuracy was 0.22 mm in the x -direction, 0.16 mm in the y -direction, and 0.10 mm in the z -direction. Surface scanning was first performed on each maxillary and mandibular dental cast independently, which registered the open-mouth position, from several different directions to eliminate blind spots. Many range images acquired from different viewpoints were aligned, merged, and outputted as two STL files using 3D scanning software (RapidForm 2006; Inus Technology Inc., Seoul, South Korea). The same procedures were performed on combined maxillary and mandibular casts with the reference splint in place, which registered the open-mouth position, and then with the combined casts at the ICP or CR. These STL files were imported into Imageware. The independent maxillary and mandibular surface models were registered using the combined surface models to reconstruct the virtual dentition in open-mouth position and ICP (VD-O and VD-C, respectively) and the reconstructed models were imported into Imageware ( Fig. 4 e–h). The fiducial markers attached to the maxillary and mandibular casts are shown in red and blue, respectively, in the virtual images.
Step 4: Initial registration of the virtual skull and dentition model
To create a preliminary fusion model of the skull and dentition in the open-mouth position pre-surgery, the VD-O image was automatically fused into the VS-O image by surface-based rigid registration (intermodality registration) of the fiducial markers (yellow) on the splint using the iterative closest point method ( Fig. 1 ). Automatic registration is a built-in function of Imageware.
Step 5: Second registration of the preliminary fusion model in the open-mouth position and dentition model in the ICP or CR
To create another preliminary fusion model of the skull and dentition, the VD-C image was further fused into the VS-O and VD-O images obtained in the previous step by an intramodality registration of the fiducial markers (red) of the maxillary cast ( Fig. 1 ). In the fusion image obtained, discrepancies in the corresponding fiducial markers (blue) of the mandibular cast indicate movement of the mandible from the open-mouth position to the ICP or CR.
Step 6: Third registration for repositioning of the mandible
A third registration of corresponding fiducial markers (blue) of the mandibular casts in the fusion images of VS-O, VD-O, and VD-C obtained in the previous step was performed, and translation vectors were calculated ( Fig. 1 ). Based on the vectors obtained, the mandible was transferred from the open-mouth position to the ICP or CR.
Step 7: Extraction of the region of interest (ROI)
To display only the region of interest (ROI), all fiducial markers in 3D images obtained in the previous step were changed to ‘undisplayed’, and protruding portions of the cast base were trimmed. As a result, a virtual patient model of the skeleton and dentition pre-surgery was obtained ( Fig. 1 ).
Step 8: Construction of the 3D coordinate system in the virtual patient model
After generating the virtual patient model, the 3D coordinate system was defined as follows: Frankfort horizontal (FH) plane (connecting the right and left points porion (Po) and left point orbitale (Or)); frontal plane (perpendicular to the FH plane and through right and left points Po); and midsagittal plane (perpendicular to the FH plane and through the midpoint between the right and left points Po (the origin)). The x , y , and z axes represent mesio-lateral, antero-posterior, and supero-inferior directions, respectively.
Evaluation of registration accuracy
To examine the accuracy of registering two images, i.e., the reference and sensed images, the root mean square error (RMSE) of the fiducial markers was calculated for each registration procedure. The RMSE was calculated from the distance between a closest pair of points on the surface of each fiducial marker in a set of two registered images. If a pair of points was defined as r s = ( x s , y s , z s ) and r t = ( x t , y t , z t ), the Euclidean distance between the two points was expressed as
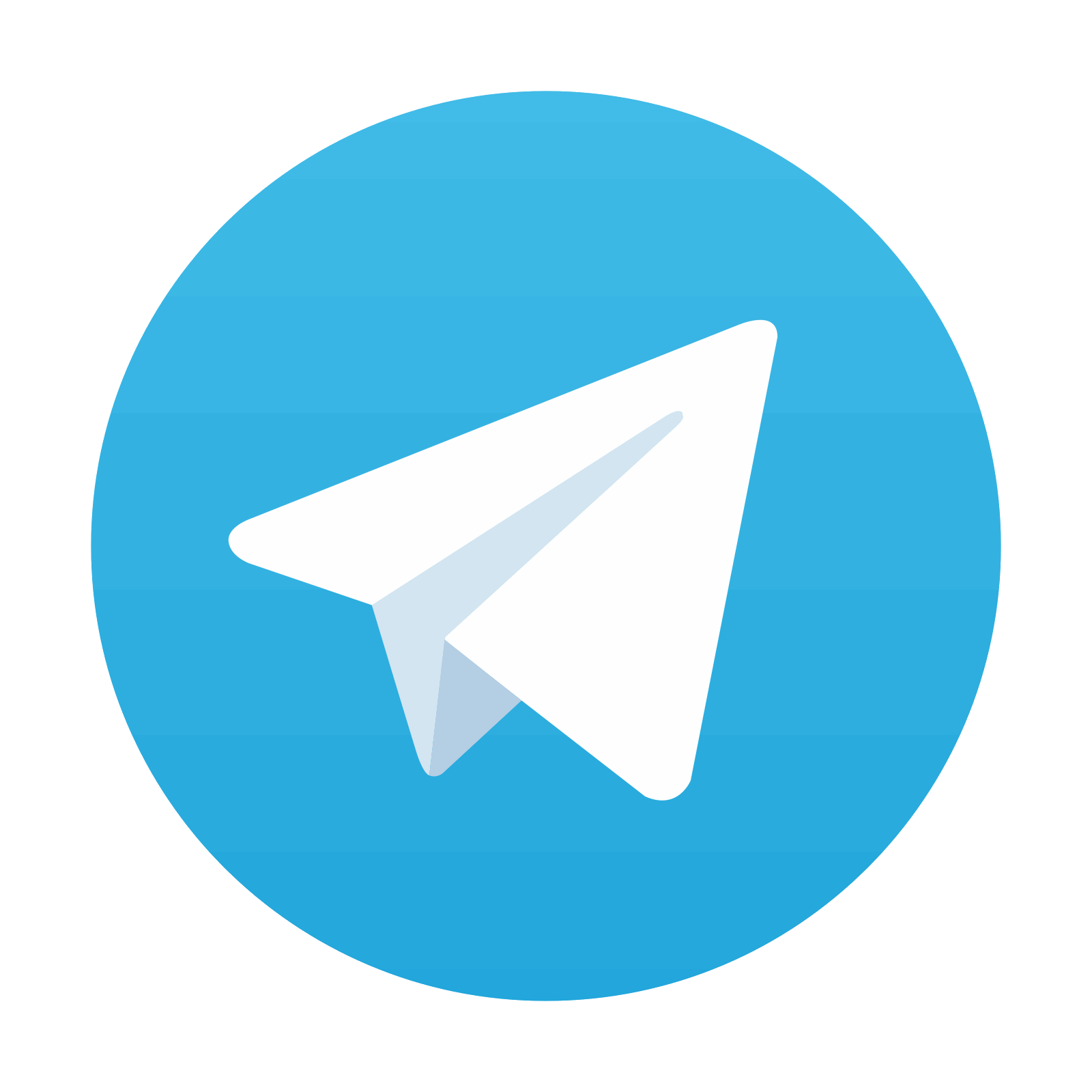
Stay updated, free dental videos. Join our Telegram channel

VIDEdental - Online dental courses
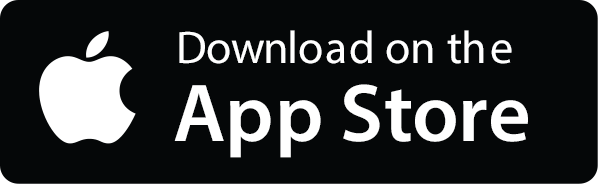
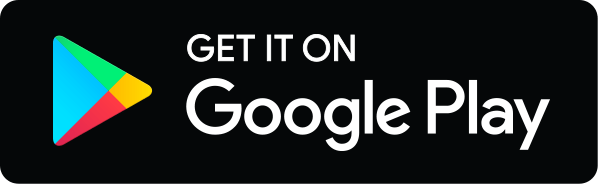