Abstract
Objectives
In the present study, lithium-disilicate ceramic inlays should be analyzed biomechanically according to their thickness and dimension, and it should be clarified as to whether there is a significant relationship between the inlay volume and the induced tensile stress level.
Methods
Using a new parametric CAD modeling procedure, 27 lithium-disilicate ceramic inlays with various parameters of “depth”, “width”, “angle” and restoration volume were generated. These inlays were integrated into the CAD model of a lower molar created from the CT data of an anatomical preparation. The resulting CAD models were, finally, three-dimensionally cross-linked to FEM models. After applying a compressive force of 200 N, Principal Tensile Stresses (PTSs) could be measured in the inlay. The values were subject to statistical analysis afterwards.
Results
The volume of the inlay restorations varied between 35.7 mm 3 and 82.5 mm 3 . The maximum PTS values ( n = 10) only showed a slight negative correlation with the inlay volume. The correlation coefficient according to Spearman was −0.082 ( p ≤ 0.001). If the highest 1000 PTS values of each inlay were considered ( n = 1000), the correlation coefficient was further reduced to +0.068 ( p ≤ 0.001). No significant correlation between the inlay volume and the induced PTS level could be detected.
Significance
Under the conditions and limitations of the present FEM study, the inlay volume did not significantly influence the tensile stress level of ceramic inlays. The results may support the thesis that volume-reduced all-ceramic inlays might not have an increased fracture risk. Further studies are needed to confirm this.
1
Introduction
All-ceramic restorations have been approved for the treatment of class II cavities. They can restore the natural morphology of the teeth, achieving both a high survival rate and a good visual appearance . A fracture of the ceramic is a considerable complication, which can lead to the failure of the restoration . In addition to the indication, material suitability and quality of the adhesive bond, the form and dimension of the cavity preparation can have an effect on long-term success. The class II cavity preparation for full ceramic restoration can be described parametrically using a basic form determined by the parameters of depth, width and angle (DWA) . However, the volume of enamel and dentin removed by preparation remains mostly unknown. In vitro tests have shown that the mechanical stability of the tooth is reduced by the preparation , but the adhesive bond between the ceramic restoration and the enamel is able to re-establish the stability of the tooth . Nonetheless, the original tooth stability cannot be recovered completely. The principle of each cavity preparation is to protect the natural enamel as far as possible , often leading to a gracile restoration. While gold inlays can almost be shaped in a gracile manner without fracturing , this is not the case with ceramic inlays . Here, the stability of the inlay depends upon its dimensions and the type of ceramic used . Accordingly, the traditional preparation guidelines for ceramic inlays are not as substance-friendly as with gold inlays, and often lead to considerable losses of enamel. With the continuous development of dental ceramics and innovative manufacturing processes , the question arises as to whether the preparation guidelines for ceramic inlays could be adapted to minimally invasive treatment. Therefore, the aim of the present study was to analyze various sizes and shapes of lithium-disilicate ceramic inlays biomechanically, and to investigate the tensile stresses induced by mastication. In particular it should be clarified as to whether the risk of fracture is higher with volume-reduced lithium-disilicate ceramic inlays than with normally dimensioned ones.
2
Materials and methods
The CT data from an anatomical preparation of the lower jaw ( Fig. 1 ) served as the morphological basis for the FEM models. The resolution was 0.08 mm of the isotropic edge length of each voxel (TomoScope HV 500, 220 kV; 0.125 mA). Using the software Amira 5.3.1 (Visage Imaging, Inc., San Diego, USA), three-dimensional polygon meshes of the anatomical structures could be generated by manual segmentation of the X-ray data. The resulting polygon meshes could be transferred into non-uniform rational B-splines (NURBS) using reverse engineering in the Rapidform XOR 3 software (INUS Technology, Inc., Seoul, Korea). Finally, a combined CAD model was created in the Inventor 2012 software (Autodesk GmbH, Munich, Germany), including an osseous segment of the mandible and tooth 46 with a periodontal ligament. The crown of tooth 46 showed a parametric preparation cavity which was combined with a corresponding parametric CAD inlay model ( Figs. 2 and 3 ).
2.1
Parametric cavity modeling
The basic form of the cavity constructed for tooth 46 was taken from the literature . Form and dimensions were determined by the three parameters depth ( D ), width ( W ) and angle ( A ), with each of them taking on a small (1), medium (2) or large (3) value ( Fig. 2 ). The depth ( D ) showed the minimal distance to the deepest point in the occlusal surface and could have a value of 1.00 mm ( D = 1), 1.25 mm ( D = 2) or 1.50 mm ( D = 3). In contrast, the width was the distance of the isthmus walls at the level of the cavity floor and could have a value of 1.50 mm ( W = 1), 2.00 mm ( W = 2) or 2.50 mm ( W = 3). The angle ( A ) defined the decline of the cavity walls and could have a value of 10° ( A = 1), 15° ( A = 2) or 20° ( A = 3). By combining all of the parameters (DWA) and values, a total of 27 CAD models with different cavity shapes was created ( Fig. 3 ), and the labeling of the individual CAD models was done using parametric coding (DWA from 111 to 333). The tooth enamel removed in the virtual preparation was replaced anatomically by a virtual lithium-disilicate ceramic inlay, which was fixed in the cavity by a 40 μm thick layer of adhesive ( Fig. 3 ).
2.2
FEM modeling
All of the CAD models were entered into the computer program ANSYS 13.0 (ANSYS, Inc., Canonsburg, USA) and three-dimensionally cross-linked to build corresponding FEM models. The edge length of the inlay elements was set at 0.05 mm ( Fig. 4 ). The selection of the element form was done by the software itself, while parabolic tetrahedrons were generated generally. The individual structures of the FEM model were defined by specific material properties ( Tables 1 and 2 ), which were taken from the literature . The ceramic used for the inlays was lithium disilicate . The contact conditions between the structures were defined as fixed. Before carrying out the statistical and mechanical analysis, and as a boundary condition, the FEM models were fixed at the lower margin of the mandible as far away as possible from the region of interest. The mesial and distal ends of the bone segment were mounted in a non-lifting, friction-free manner and a compressive force of 200 N was applied on the occlusal contact in the central region of the ceramic inlay. The direction of the force vector was parallel to the longitudinal axis of the tooth. After carrying out the simulation ( Fig. 5 ), the Principal Tensile Stress (in MPa) could be measured at the nodes of the FEM inlay model. All of the PTS values were tested for singularities, but none were found in all the inlays as the values sorted by size resulted in a continuous function.
Young’s modulus | Poisson’s ratio | References | |
---|---|---|---|
Dentin | 24.4 GPa | 0.43 | |
LS 2 ceramic (e.max Press) | 91.0 GPa | 0.23 | |
Nerve tissue | 0.058 GPa | 0.42 | |
Periodontal ligament | 0.050 GPa | 0.49 | |
Enamel | 80.0 GPa | 0.25 | |
Adhesive (Variolink II) | 10.31 GPa | 0.24 |
Compact bone | Cancellous bone | |
---|---|---|
E x | 12.6 GPa | 1.148 GPa |
E y | 19.4 GPa | 1.148 GPa |
E z | 12.6 GPa | 0.21 GPa |
G xy | 5.7 GPa | 0.434 GPa |
G yz | 5.7 GPa | 0.068 GPa |
G xz | 4.85 GPa | 0.068 GPa |
v xy | 0.253 | 0.322 |
v yz | 0.390 | 0.055 |
v xz | 0.300 | 0.055 |
2.3
Statistical analysis
For all 27 FEM models, the volume of the ceramic inlay (in mm 3 ) was calculated and the highest 10 Principal Tensile Stress (PTS) values were measured. Due to possible structural imperfections within the ceramic, a tear can also be initiated by slightly lower stress values than the maximum PTS. Therefore, the highest 1000 PTS values within each inlay were also considered for further statistical analysis. The analyzed nodes ( n = 1000) corresponded to an inlay volume of 0.01 mm 3 . The calculated volumes and the measured PTS values were fed into the statistical program IBM SPSS Statistics 19 (IBM Deutschland GmbH, Ehningen, Germany) and analyzed descriptively and exploratively ( Table 3 ). The variables “inlay volume” and “depth” of the lithium-disilicate inlay should be correlated with the induced PTS values. Since these variables were not normally distributed in the Kolmogorov–Smirnov test, the rank correlation according to Spearman was calculated.
Inlay (DWA) | Volume (mm 3 ) | Elements | Nodes | n | Mean (MPa) | Median (MPa) | SD (MPa) | Min (MPa) | Max (MPa) |
---|---|---|---|---|---|---|---|---|---|
111 | 35.68 | 2513946 | 3436435 | 1000 | 85.93 | 82.57 | 11.00 | 73.21 | 121.72 |
112 | 39.32 | 2760141 | 3770545 | 1000 | 86.89 | 83.39 | 11.32 | 74.16 | 132.22 |
113 | 43.04 | 3029442 | 4135864 | 1000 | 87.47 | 83.76 | 11.11 | 74.80 | 132.01 |
121 | 46.88 | 3296942 | 4496796 | 1000 | 86.90 | 83.32 | 11.22 | 74.12 | 130.97 |
122 | 50.82 | 3569901 | 4866641 | 1000 | 87.06 | 83.09 | 11.24 | 74.28 | 129.28 |
123 | 54.97 | 3866478 | 5268892 | 1000 | 87.29 | 83.99 | 11.35 | 74.45 | 132.28 |
131 | 58.98 | 4145188 | 5644779 | 1000 | 87.14 | 84.03 | 11.25 | 74.19 | 136.44 |
132 | 63.24 | 4446687 | 6053683 | 1000 | 86.81 | 83.57 | 11.14 | 74.04 | 126.07 |
133 | 67.89 | 4767963 | 6489048 | 1000 | 87.26 | 83.82 | 11.19 | 74.80 | 128.71 |
211 | 39.38 | 2772525 | 3786712 | 1000 | 85.84 | 82.56 | 10.80 | 73.21 | 124.47 |
212 | 43.71 | 3060791 | 4177898 | 1000 | 87.06 | 83.80 | 11.32 | 74.09 | 131.47 |
213 | 47.93 | 3375077 | 4603367 | 1000 | 87.40 | 84.13 | 11.19 | 74.85 | 127.20 |
221 | 51.67 | 3631954 | 4949002 | 1000 | 86.42 | 82.81 | 11.32 | 73.80 | 125.62 |
222 | 56.35 | 3946091 | 5374061 | 1000 | 87.26 | 83.40 | 11.24 | 74.45 | 128.72 |
223 | 60.87 | 4290408 | 5841203 | 1000 | 86.55 | 83.10 | 11.42 | 73.62 | 126.35 |
231 | 64.90 | 4557607 | 6200988 | 1000 | 86.69 | 83.23 | 11.24 | 73.72 | 126.64 |
232 | 69.88 | 4907382 | 6674346 | 1000 | 86.75 | 83.10 | 11.19 | 74.07 | 126.82 |
233 | 75.14 | 5280173 | 7179642 | 1000 | 86.97 | 83.69 | 11.24 | 74.37 | 129.89 |
311 | 43.10 | 3034943 | 4141352 | 1000 | 85.42 | 82.24 | 11.05 | 72.64 | 125.31 |
312 | 47.71 | 3365798 | 4589774 | 1000 | 86.33 | 82.80 | 11.30 | 73.76 | 131.75 |
313 | 53.00 | 3727179 | 5079232 | 1000 | 87.05 | 83.73 | 11.25 | 74.38 | 128.73 |
321 | 56.51 | 3964626 | 5397726 | 1000 | 86.52 | 83.25 | 11.12 | 73.73 | 124.85 |
322 | 61.72 | 4330006 | 5892321 | 1000 | 86.30 | 83.03 | 11.31 | 73.57 | 127.89 |
323 | 67.20 | 4724292 | 6426672 | 1000 | 86.67 | 83.17 | 11.07 | 74.10 | 127.18 |
331 | 70.77 | 4970283 | 6757195 | 1000 | 86.93 | 83.46 | 11.16 | 74.35 | 127.22 |
332 | 76.43 | 5370545 | 7299006 | 1000 | 86.82 | 83.31 | 11.03 | 74.51 | 126.56 |
333 | 82.47 | 5802512 | 7884044 | 1000 | 87.08 | 83.86 | 11.06 | 74.40 | 127.72 |
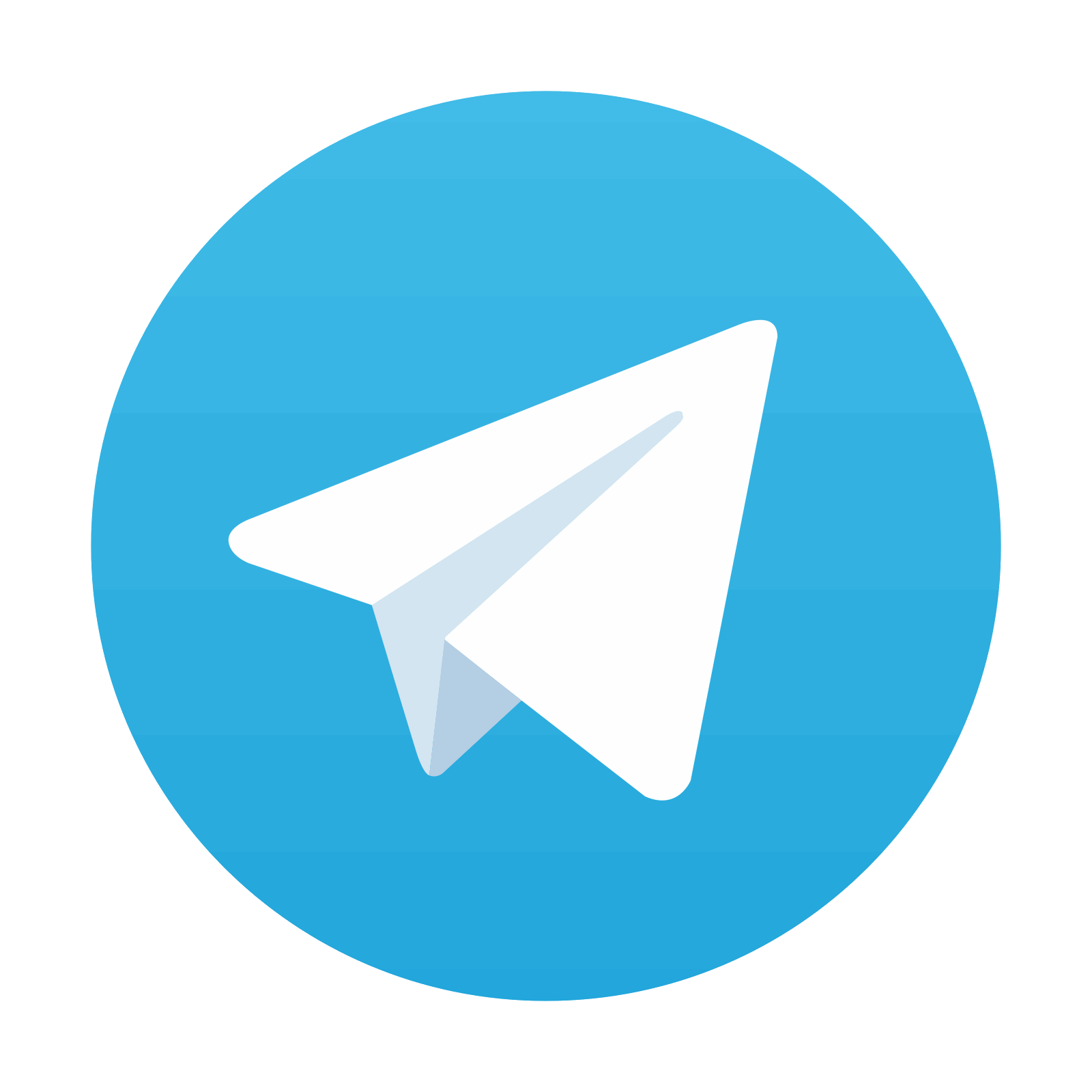
Stay updated, free dental videos. Join our Telegram channel

VIDEdental - Online dental courses
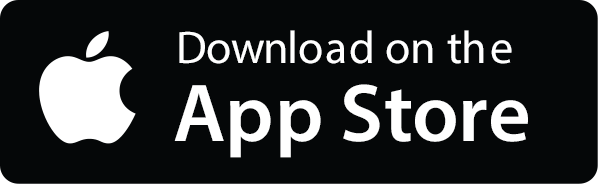
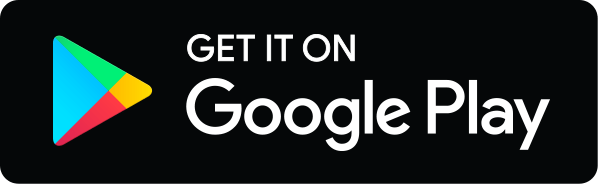
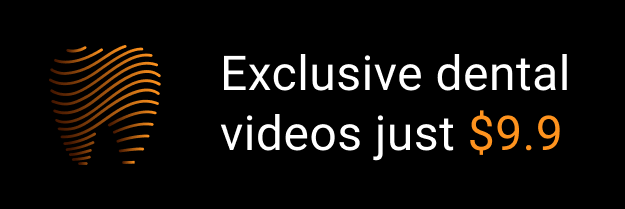