Highlights
- •
Fibers improve mechanical properties of a resin modified glass ionomer cement (RMGIC).
- •
Mechanical properties with RMGIC matrix are improved over GIC matrix.
- •
Braided long fibers give higher flexural strength than discontinuous short fibers.
- •
Resin pre-impregnation of braided long fibers greatly enhanced the flexural strength.
- •
Pre-impregnation of the short glass fibers had no effect on mechanical properties.
Abstract
Objectives
Understand how discontinuous short glass fibers and braided long fibers can be effectively used to reinforce a resin modified glass ionomer cement (RMGIC) for carious lesion restorations.
Methods
Two control groups (powder/liquid kit and capsule) were prepared from a light cured RMGIC. Either discontinuous short glass fibers or braided polyethylene fiber ribbons were used as a reinforcement both with and without pre-impregnation with resin. For the former case, the matrix was the powder/liquid kit RMGIC, and for the latter case the matrix was the capsule form. Flexural strength was evaluated by three-point beam bending and fracture toughness was evaluated by the single-edge V-notch beam method. Compressive strength tests were performed on cylindrical samples. Results were compared by analysis of variances and Tukey’s post-hoc test. Flexural strength data were analyzed using Weibull statistical analysis.
Results
The short fiber reinforced RMGIC both with and without pre-impregnation showed a significant increase of ∼50% in the mean flexural strength and 160–220% higher fracture toughness compared with the powder/liquid RMGIC control. Reinforcement with continuous braided fibers gave more than a 150% increase in flexural strength, and pre-impregnation of the braided fibers with resin resulted in a significant flexural strength increase of more than 300% relative to the capsule control. However, for the short fiber reinforced RMGIC there was no significant benefit of resin pre-impregnation of the fibers. The Weibull modulus for the flexural strength approximately doubled for the fiber reinforced groups compared to the control groups. Finally, compressive strength was similar for all the groups tested.
Significance
By using a RMGIC as a matrix, higher flexural strength was achieved compared to reported values for short fiber reinforced GICs. Additionally, the short fibers provided effective toughening of the RMGIC matrix by a fiber bridging mechanism. Finally, continuous braided polyethylene fibers gave much higher flexural strength than discontinuous glass fibers, and their effectiveness was enhanced by pre-impregnation of the fibers with resin.
1
Introduction
The maintenance of oral health in geriatric patients is a significant issue in dental care. Age-related salivary changes such as xerostomia combined with other factors including poor oral hygiene or gingival recession exposing root surfaces tend to result in a higher prevalence of caries in elderly patients [ ]. Currently, the minimal intervention approach known as Atraumatic Restorative Treatment (ART) has been the treatment of choice for these patients [ ]. The ART technique has numerous advantages compared to conventional adhesive restorations such as it does not require local anesthesia, a rubber dam for isolation, or drilling and it can be performed in a shorter treatment time, thus reducing patient discomfort.
However, restorations placed using the ART technique only achieve high survival rates for relatively small cavities with sufficient support of tooth structure, such as in class I occlusal restorations [ ]. In addition, clinical studies have shown that restorations placed in the root and cervical surfaces are one of the least durable types of restorations [ , ]. Indeed, they commonly experience high loss of retention, failure at the restoration margins, and secondary caries [ , ]. The high failure rates of complex cavities and Class V restorations are related to the poor mechanical properties of the materials used [ ]. Currently, the preferred material for root and cervical lesion restorations are glass ionomer cements (GICs) and resin modified GICs (RMGICs) [ , ] due to their excellent adhesion to the tooth and their natural capacity for fluoride release. Furthermore, compared to resin-based composites, GICs are easier to handle and require less steps during the placement procedure.
Although RMGICs represent a significant improvement in mechanical properties compared with conventional GICs, the strength and fracture resistance remains much less than typical resin composites [ , ], and this is a significant concern when using them for permanent restorations. A few studies have shown that adding fiber reinforcements represents one potential way to improve the strength and fracture resistance for this class of dental materials [ ]. However, one challenge is achieving good bonding between the matrix and the fibers [ ], and no studies have examined fiber reinforcement of RMGICs.
For fiber composite reinforcement, it is well known that control of the fiber/matrix interfacial properties is essential for achieving good mechanical properties [ ]. In the field of resin based dental restorative composites, surface treatments such as cold gas plasma, silanization, or etching have been used to improve the interfacial bonding properties [ , ].
In addition, studies show that fibers pre-impregnated with resin can have improved interfacial bonding, resulting in higher flexural strength [ ], and this methodology has been successfully applied to porous continuous fibers for periodontal and post traumatic splints [ ] and orthodontic retainers [ ].
The purpose of this study was to evaluate the mechanical properties of resin modified glass ionomer cements reinforced using discontinuous short glass fibers or braided long fibers. Additionally, the second purpose was to examine the effect of resin pre-impregnation of the fibers on the mechanical properties. The hypotheses of this study were that (1) by using a resin modified GIC matrix, enhanced properties would be achieved relative to fiber reinforced GICs that have been reported in the literature, (2) the RMGIC reinforced with long braided fibers would perform better than with short discontinuous fibers and (3) that fibers pre-impregnated with resin would give improved mechanical properties compared to non-impregnated fibers.
2
Materials and methods
2.1
Specimen preparation
Two control groups (powder/liquid kit and capsule) were prepared from a light cured, resin modified glass ionomer cement (Riva Light Cure, SDI Limited, Australia) following the manufacturer dose and mixing recommendations. The hand mixed group was prepared with a powder-to-liquid mass ratio of 3.1:1. The capsule group was activated and mechanically mixed in an amalgamator for 10 s.
Braided polyethylene woven fibers ribbons (Construct™, Kerr, USA) were used as a reinforcement with and without pre-impregnation with flowable resin (Construct™, Kerr, USA) in a laminate construction ( Fig. 1 ) that may be quickly applied in a clinical setting. For the impregnated group, the cut ribbons of fibers were placed on a glass slab and using a spatula the fiber was saturated with resin on both sides. The impregnated fiber was kept away from light until it was incorporated with the RMGIC and the whole composite structure was cured as described below. Additional details on the RMGIC and the flowable resin can be found in Table 1 .

Material | Manufacturer | Composition |
---|---|---|
Resin-modified glass-ionomer cement | Riva Light-Cure Powder/Liquid and Capsule (SDI Limited, Australia) | Liquid: polyacrylic acid, tartaric acid, HEMA; powder: fluoroaluminosilicate glass |
Flowable resin | Construct™ (Kerr, USA) | a Filler (wt.%): 30–60; a Filler composition: glass, oxide, fumed silica; resin composition: bis-EMA, TEGDMA, light-cure initiators, and stabilizers |
For the flexural strength samples, the 1.0 mm wide fiber ribbons were cut into 25 mm lengths and placed at the base of a stainless steel split mold. The fiber ribbon was covered with the RMGIC capsule material to fill the mold. Compressive strength specimens were prepared with a 3 mm braided fiber ribbon. The fibers were stretched to 4 mm width and placed in the middle of the cylinder height between layers of RMGIC ( Fig. 1 ).
Discontinuous short glass fibers (S-2 glass® fibers, AGY, USA) with a diameter of 5 μm were cut by the manufacturer into a uniform length of 0.5 mm to create fibers with an aspect ratio of ∼100. Adding fibers to the capsules was trialed but did not allow for good mixing. So, instead the short fibers were hand mixed with the bulk powder/liquid kit formulation of the same RMGIC (Riva Light Cure, SDI Limited, Australia). The short fibers were treated in two different ways: (1) wetted with the mixing liquid from the RMGIC kit or (2) pre-impregnated with flowable resin (Construct™, Kerr, USA).
For the former group, the short fibers (5 vol.% = 25 mg) were wetted with two drops of the mixing liquid. For the latter group, the short fibers were placed on a glass slab and the fibers were saturated with 75 mg of flowable resin. Two doses of powder were dispensed onto the mixing pad with two drops of liquid. Half of the powder was mixed with the liquid for 15 s. Then the wetted fibers were added with the other half of the powder followed by another 15 s of hand mixing. The mixture was then placed in a stainless-steel split mold for curing.
For all groups, the mixed material was pressed flat in the mold with clear polymer strips and a glass slide. The beam specimens were light cured by three adjacent exposures of 20 s and the cylinder specimens were photoactivated for 20 s from each side of the split mold. All samples were light-cured in continuous mode with an irradiance of 1200 mW/cm 2 using a commercial light curing unit (Radii-Cal, SDI Limited, Australia). Any excess material at the mold edges was removed by polishing and a thin coating of petroleum jelly was applied to each sample, which is the typical clinical procedure. The samples were stored in deionized water at 37 °C for 24 h prior to mechanical testing. All subsequent mechanical tests were performed using a universal testing machine (Instron 4501, Instron Corp., USA) with a crosshead speed of 0.75 mm/min.
2.2
Flexural strength
Flexural strength experiments were performed in general accordance with ISO standard 4049 [ ] using three-point bending with a support span distance of L = 20 mm. The unnotched flexural strength specimens ( n = 15 for each group) were loaded to fracture. The flexural strength values, FS , for the control samples and for the short fiber reinforced RMGIC were calculated according to [ ]:
FS=3PL2bh2
where P was the load at the fracture point, L was the support span distance, b was the specimen width, and h was the specimen height. For the braided long fiber samples, the fibers were oriented parallel to the length and on the tension side of the bend beams ( Fig. 1 ). Due to the laminate nature of the beam samples, the flexural strength was calculated using the transformed section method [ ]. Using this method, a laminate of two materials with different elastic modulus can be transformed to an equivalent cross-sectional shape of homogeneous material, which in this case was chosen as the RMGIC. To obtain the transformed section, the effective beam width of the braided fiber layer was increased based on the modulus ratio according to:
bbraidedfiber’=ERMGICEbraidbbraid
where b’ was the transformed width and b was the actual width.
The modulus of elasticity of the RMGIC, E RMGIC, was calculated from the slope, α , of the linear elastic region of the load-displacement curves during the control sample flexural tests according to:
ERMGIC=αL34bh3
This procedure gave a value of E RMGIC = 7 GPa.
To obtain the modulus of elasticity for the braided fibers, E braid , five pre-impregnated fiber specimens of approximately 20 mm length were prepared for tension testing. The wetted fiber braids were pressed flat with a glass slide and light-cured by three adjacent exposures of 20 s. The braided fibers were tested in tension at a crosshead speed of 0.2 mm/min using a 150 N capacity load cell in a loading stage (MICROTEST 2 kN, Deben UK Ltd, UK). The loading stage was placed under an optical microscope to measure the sample displacements. E braid was determined according to:
Ebraid=σL0ΔL
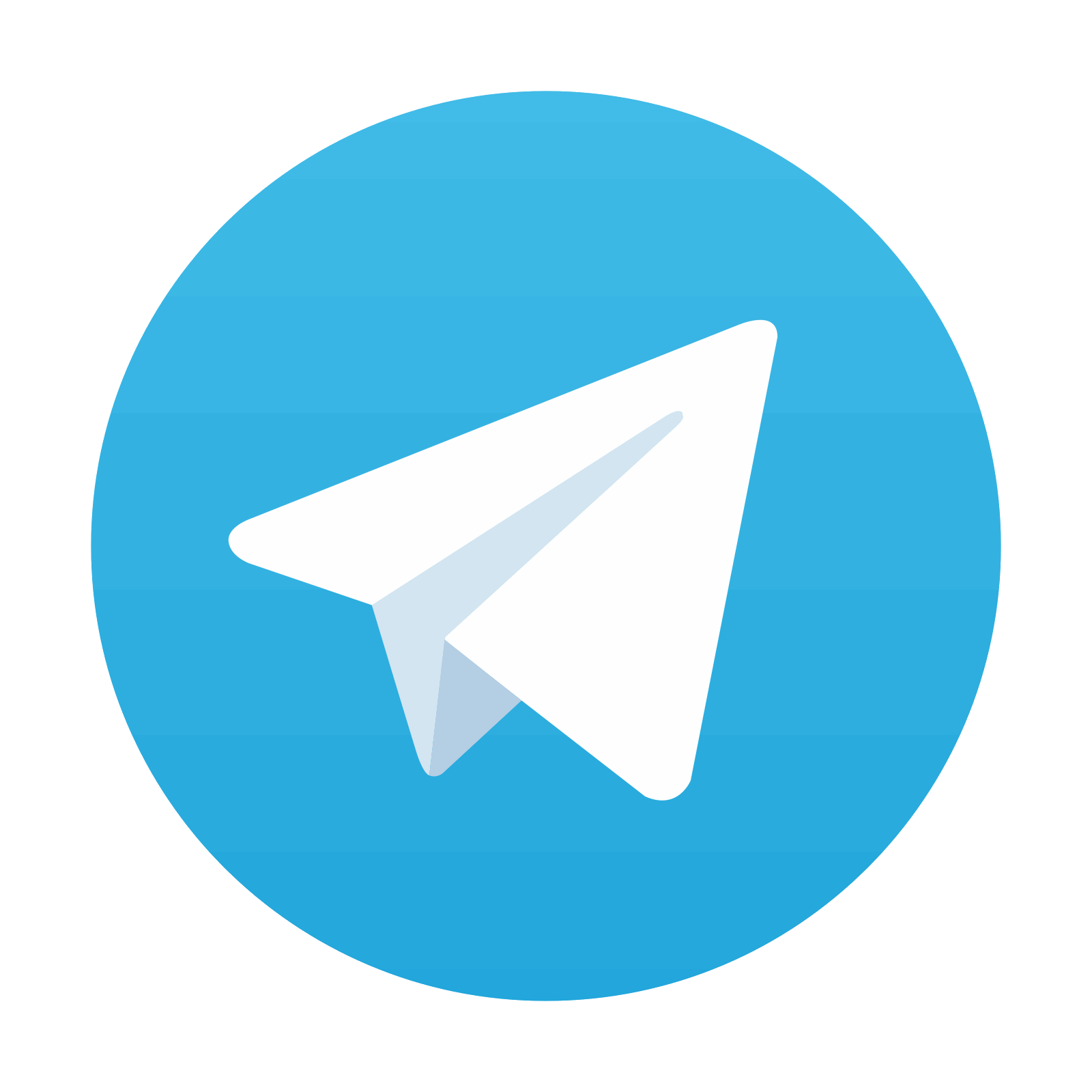
Stay updated, free dental videos. Join our Telegram channel

VIDEdental - Online dental courses
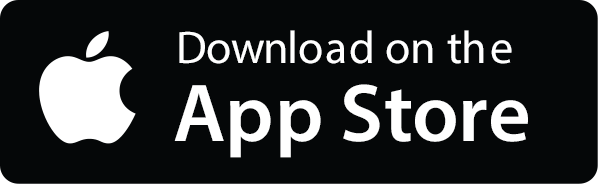
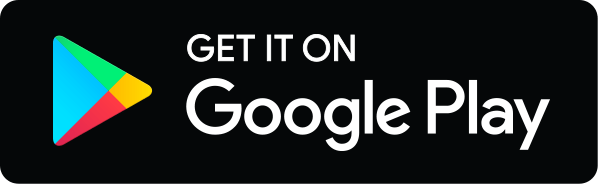
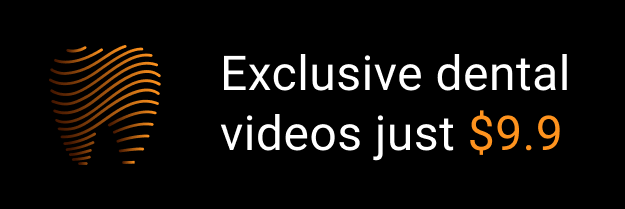