Fig. 2.1
(a) Type I primary failure of eruption in a developing dentition (7.5-year-old child). The right posterior segment shows a progressive eruption failure in the upper and lower arches with an eruption pathway that is clear. The left posterior segment does not appear affected. (b) Failure of eruption due to a bony pathway that is not clear. The alveolar bone can still be observed coronal to the erupting first molar in the lower left quadrant. This scenario often represents an idiopathic eruption failure due to some other pathology (i.e., not PFE or syndromic cause)
The remaining theories possibly offer the strongest explanation of tooth eruption, particularly in light of recent molecular biological advances [4, 5, 8]. However, these theories have fueled a debate of whether the bone “pushes” the teeth or the tooth “pulls” the bone with it during the eruption process. The “bone remodeling theory,” originally proposed by Ten Cate [11] and endorsed by Wise et al. [14, 21], asserts that bone growth within the area apical to the developing tooth “pushes” the tooth during the eruption process. The consideration is whether this bone growth is causal and indeed represents the “motive force” described by Wise [14] or whether the bone growth occurs as a response to the occlusal movement of the developing tooth. Significant evidence exists in animal models to support this theory; experiments in rats reveal that the amount and duration of bone growth occurring at the apical base of the tooth is necessary and sufficient to propel the tooth into the oral cavity [14]. However, the fact remains that tooth eruption in humans occurs over a protracted time period with limited accessibility for study and the above studies in rodents may not parallel the human response; hence the complete understanding of events leading to eruption in humans remains elusive. The theory of preemergent eruption that most closely fits this model is the “dental follicle theory,” which relates to the physiologic coupling of the resorptive eruption path formation and root development processes and contends that the dental follicle is necessary for eruption [12, 13]. This provides the most compelling explanation of the mechanism underlying tooth eruption. Moreover, it is this theory that aligns best with the “bone remodeling theory” and the association of the PTH1R gene with PFE. The follicle theory stems from classical studies in dogs where removal of the dental follicle prevented eruption [12, 13]. The dental follicle has since been shown to provide the environment and chemoattractants for monocytes to differentiate into osteoclasts [5]; this facilitates the bone resorption necessary for normal tooth eruption. Specifically, stellate reticulum cells found in the dental follicle are observed to secrete parathyroid hormone-related peptide (PTHrP), which induces overexpression of colony-stimulating factor-1 (CSF1) and receptor activator of NF-kappaB ligand (RANKL) responsible for osteoclastogenesis [24, 25]. A concomitant overexpression of BMP2 that leads to osteogenesis is occurring in the apical end of the dental follicle [24] in a chronological and spatial fashion [21].
The complete explanation of the physiologic coupling of the eruption and resorption processes associated with preemergent tooth eruption is not yet fully understood, but we know that the molecular crosstalk surrounding the erupting tooth is somehow activated upon completion of the crown. We can therefore postulate that the rate-limiting factor of preemergent eruption is the resorptive pathway formed by osteoclast cells. Accordingly, a tooth embedded in the bone has the potential to begin to erupt after root formation is completed, as long as the eruptive pathway is mechanically cleared at the appropriate developmental stage. This natural phenomenon of a “clear pathway” forms the basis of the diagnostic rubric for eruption disorders discussed in detail below.
Theories Associated with Postemergent Tooth Eruption
Although the dominant theories of tooth eruption appear to correlate with the preemergent stage, the postemergent stage of eruption is central to some theories. Postemergent tooth eruption is defined as the eruption stage of a developing tooth after it has broken through the gingiva into the oral cavity. This stage continues until the tooth reaches the level of the occlusal plane and is in complete function and the overall growth of the jaws has completed. Postemergent eruption is further broken into four phases, the pre-functional spurt (rapid phase), the juvenile occlusal equilibrium (slower phase), the pubertal or adolescent eruptive spurt, and the adult occlusal equilibrium. After the gingival barrier is broken, the postemergent spurt results in rapid eruption until the tooth reaches the level of functional occlusion. As the tooth continues to erupt during its postemergent stage, the theory of “collagen cross-linking, contraction, and maturation” introduced above becomes more viable, due to the fact that the PDL indeed becomes more organized after the tooth comes into functional occlusion. This theory contends that increased organization in collagen cross-linking creates a propulsive thrust to facilitate eruption. Even though the tooth is subjected to occlusal forces, the actual eruption rate is increased.
The “hydrostatic pressure theory” occurs during postemergent eruption and is based on the ability of the extracellular matrix apical to the developing tooth to swell considerably (30–50 %) facilitating occlusal migration of the tooth [22, 23]. This theory asserts that increases in the periapical tissue fluid pressure (especially vasculature) push the tooth occlusally [11]. Moreover, human studies of premolar eruption following a local injection of vasodilators resulted in tooth eruption [23]. The argument against this theory is that a short-lived exposure to pharmacologic agents such as vasodilators would not be sufficient to sustain the long-term physiologic activity necessary for tooth eruption [14].
Postemergent Eruption and the Equilibrium Theory
After the functional plane is reached by the tooth, it undergoes the juvenile occlusal equilibrium, in which the eruption of the tooth is balanced in response to the vertical growth of the mandibular ramus. As the mandible grows vertically away from the maxilla, the teeth have more room to erupt occlusally in order to maintain occlusal contact with the opposing arch. This model of tooth eruption reinforces the idea that postemergent tooth eruption, after reaching functional occlusion, is controlled by forces impeding eruption, as opposed to encouraging forces. These balancing forces of masticatory function and the soft tissue pressures from the lips, cheeks, and tongue are the rate-limiting factors of post-functional occlusal eruption [1]. However, studies have shown that lasting eruptive movement occurs while the teeth are not in contact, which supports the idea that most of the eruptive control is based on the light and continuous force of the soft tissues. While the mechanism itself is not fully understood, when this process of vertical growth and occlusal tooth eruption is not adequately matched, eruption problems arise, as seen with issues of ankylosis and other eruption disorders which can result in areas of posterior open bites and over-closed jaw relationships.
The last phase of postemergent eruption is called the adult occlusal equilibrium. In this continuous phase, teeth will continue to erupt at an extremely slow rate throughout adult life. It has been demonstrated that if a tooth is lost at any age, the opposing tooth has the ability to erupt more rapidly, demonstrating that the eruption mechanism remains active throughout life and is capable of producing significant tooth during any stage in the life cycle. Finally, both pre- and postemergent eruption stages play a significant role in clinical eruption disorders and form the basis of our diagnostic approach reviewed below.
Diagnosis of Tooth Eruption Problems
While rodent models and molecular advances lend some support to the various theories of eruption, the details of the entire process of tooth eruption, including the micro- and macro-environment, remain poorly understood. Nonetheless, the biological facts surrounding the proposed theories provide the basis for understanding and diagnosing clinical disorders of eruption. Accordingly, adopting a diagnostic system that uses biologically rather than clinically based categories would provide a more effective means of accurately distinguishing eruption disorders [26]. Such categories should include those based on (1) a biological dysfunction such as PFE or eruption failure secondary to a genetic syndrome [16] and/or (2) a physical obstruction such as mechanical failure, cysts, and lateral tongue pressure, for instance. Impacted teeth may potentially belong to either of the above categories depending upon the location of the impacted tooth (i.e., palatal canine impaction versus buccal canine impaction). While the occurrence of palatally impacted canines is hypothesized to be both multifactorial and genetic in origin [27–29], teeth can also become impacted secondary to an obstruction of the eruption pathway, such as crowded dental arches.
It is for this reason that a diagnostic rubric to distinguish eruption disorders must ask the necessary question “is the eruptive pathway clear?” [30]. The answer to this creates the foundation for determining whether the eruption failure is due to an obstruction or not. The diagnostic rubric shown in Fig. 2.2a is based on studies that examined characteristics of eruption disorders; the accompanying case study (Fig. 2.2b, c) nicely illustrates how this tool can be utilized for a clinical diagnosis. The combination of objective genetic information and clinical data from affected persons can be used to establish a genotype-phenotype correlation for PFE and, by extension, an objective diagnosis, i.e., determined by associating clinical (phenotypic) features with genetic (genotypic) analysis. Eruption disorders from a cohort of 64 patients were analyzed phenotypically and genetically in order to categorize them into clinical groups: (1) those definitively diagnosed with PFE through genetic analysis, (2) those that showed a mutation in PTH1R (n = 11; genetic PFE cohort), (3) patients diagnosed with PFE based on clinical records alone (n = 47; clinical PFE cohort), and (4) patients diagnosed with ankylosis based on clinical criteria (N = 6; clinical ankylosis cohort). Those in the ankylosis cohort had a confirmed history of trauma or were treated with extraction of the affected tooth or teeth and had successful orthodontic treatment of the remaining teeth. All other subjects were diagnosed with PFE based on history of unsuccessful orthodontic treatment or genetic analysis. For those PFE patients who underwent genetic (mutational) analysis, a mutation or polymorphism in the PTH1R gene was identified in 11 patients, and an unclassified nonfunctional single nucleotide polymorphism in PTH1R was identified in the remaining [30]. Based on the findings of the above study, collectively all PFE subjects (genetic cohort) had at least one affected first permanent molar; the affected teeth in each dental quadrant were adjacent to one another and had a supracrestal presentation (i.e., completely cleared eruption pathway, with no alveolar bone occlusal to the affected tooth). These criteria represent the hallmark features of PFE versus a mechanical obstruction since it is based on cases of PFE genetic cohort that were categorized based on objective genetic confirmation. Other classifications of PFE include type I versus type II PFE [7, 26]. Type I is marked by a progressive open bite from the anterior to the posterior of the dental arches, while type II presents similarly but with greater although inadequate eruption of a second molar (Fig. 2.3). In either case, we speculate that the eruption defect, which we now know is genetically controlled, is expressed at the same developmental time for all affected teeth but the predominant “molar” phenotype that we observe may be the result of a coordinated series of molecular events that act in a temporally and spatially specific manner such that posterior rather than anterior alveolar bone is affected. The exact reason for the variation in eruption potential between the first and second molars in type II is unknown but may be related to this same temporal and spatial specificity of expression.
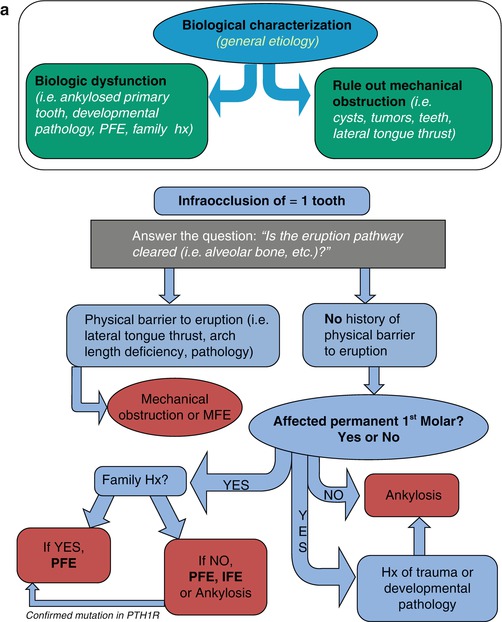
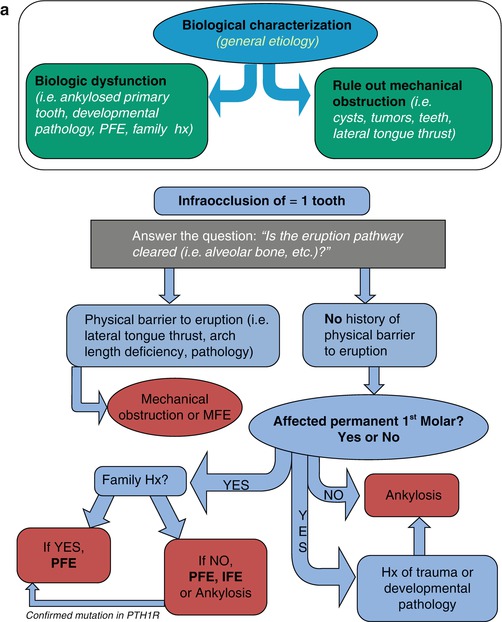
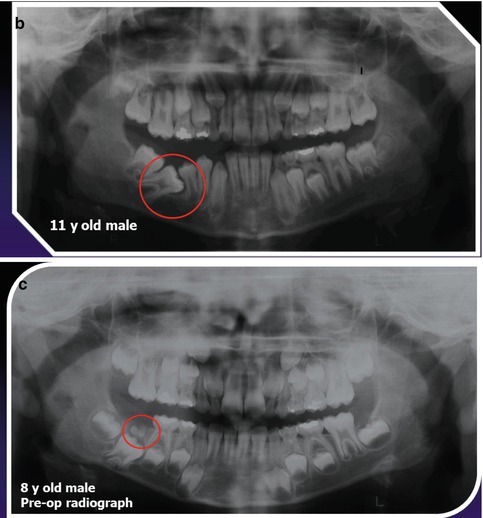
Fig. 2.2
(a) Diagnostic rubric for nonsyndromic eruption disorders based on a retrospective study of PFE subjects who carry a mutation in the PTH1R gene and those who do not. The flowchart provides a decision tree to allow a more systematic diagnosis of eruption disorders. Although there is still some uncertainty, initially sorting based on biological versus mechanical factors provides a sound basis to triage clinical scenarios (b, c). In the clinical scenario shown here, the natural history of this patient was extremely important. A differential diagnosis of the initial panoramic radiograph taken at the information gathering visit could be PFE or MFE. It was evident after acquiring historical radiographs (3 years earlier) that the cause of the eruption failure was an odontoma that was not removed before the 6 year molar was ready to erupt. Shown encircled is the unerupted 6 year molar in the 8 year old patient radiograph (2.2c) and the same molar at 11 years old that is now permanently impacted. Removal of the adjacent second premolar allowed eruption of the impacted molar and correction of the subsequent malocclusion. Reprinted from American Journal of Orthodontics and Dentofacial Orthopedics. Aug 144 (2)194–202
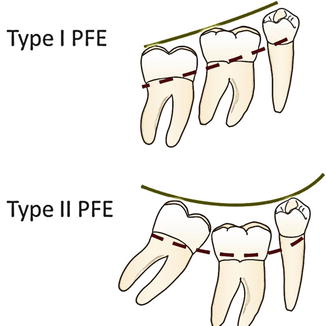
Fig. 2.3
Graphic representation of primary failure of eruption in type I and type II showing the progressive worsening of the posterior lateral open bite, to a lesser extent in type II PFE. Ankylosis occurs at about a 6.6% prevalence with mandibular primary molars more commonly affected than maxillary primary molars [38].
Despite the more definitive criteria established through the eruption disorder rubric, difficulty still exists for those clinical situations that present with isolated ankylosis since it may initially appear indistinguishable from PFE. Ankylosis, or the fusion of a tooth to the bone in the absence of a periodontal ligament, can be thought of as a mechanical eruption failure, primarily because it can occur secondary to trauma and the fusion to the bone provides a mechanical barrier to eruption [31]. It is true that ankylosis can also occur secondarily from orthodontic forces applied to a tooth with a defective eruption mechanism as in PFE [3]. The diagnosis of ankylosis can at times be made radiographically by the absence of a periodontal ligament space [32] and based on the absence of physiologic mobility and the sharp solid sound on percussion of the tooth [31]. However, the determination of an absent periodontal ligament space can be often misinterpreted on a radiograph (e.g., if ankylosis occurs in facial/lingual root surfaces, the PDL loss will not be visible on a 2D radiograph), making the diagnosis of ankylosis somewhat subjective [33]. In these instances, ankylosis can be difficult to distinguish from PFE. This fact has been exemplified in two siblings previously diagnosed with ankylosis that were re-diagnosed as PFE following identification of a mutation in the PTH1R gene [4] (Figs. 2.4a
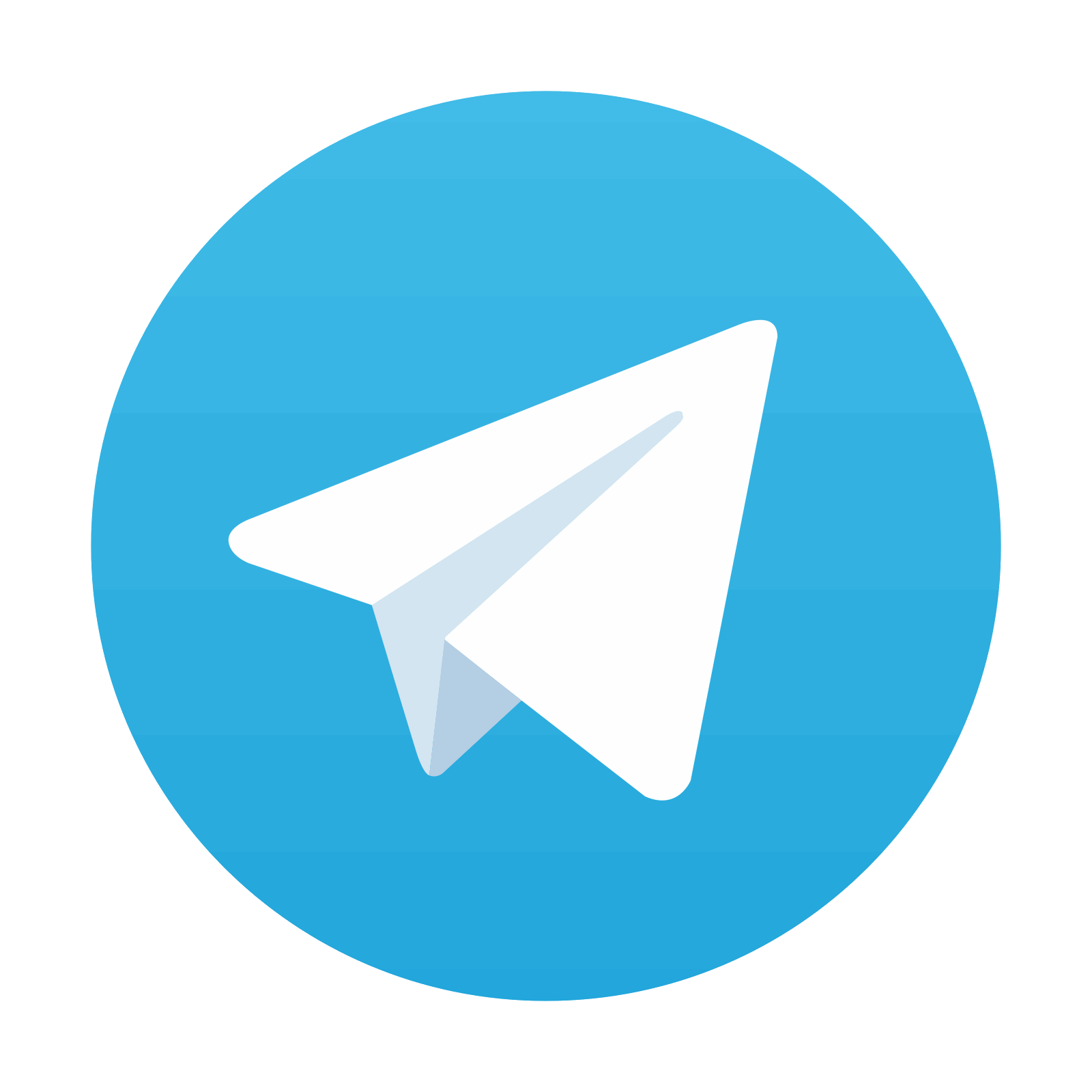
Stay updated, free dental videos. Join our Telegram channel

VIDEdental - Online dental courses
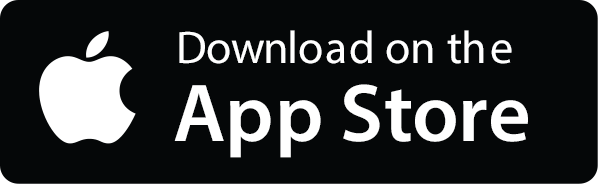
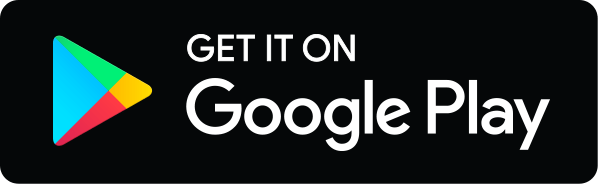