Abstract
Objective
To investigate the reinforcement of Bis-GMA/TEGDMA dental resins (without conventional glass filler) and the corresponding composites (with conventional glass filler) containing varied mass fractions of halloysite nanotubes (HNTs).
Methods
Three dispersion methods were studied to separate the silanized halloysite as individual HNTs and to uniformly distribute them into dental matrices. Photopolymerization induced volumetric shrinkage was measured by using a mercury dilatometer. Real time near infrared spectroscopy was adopted to study the degree of vinyl double bond conversion and the photopolymerization rate. Mechanical properties of the composites were tested by a universal mechanical testing machine. Analysis of variance (ANOVA) was used for the statistical analysis of the acquired data. Morphologies of halloysite/HNTs and representative fracture surfaces of the reinforced dental resins/composites were examined by SEM and TEM.
Results
Impregnation of small mass fractions ( e.g. , 1% and 2.5%) of the silanized HNTs in Bis-GMA/TEGDMA dental resins/composites improved mechanical properties significantly; however; large mass fractions ( e.g. , 5%) of impregnation did not further improve the mechanical properties. The impregnation of HNTs into dental resins/composites could result in two opposite effects: the reinforcing effect due to the highly separated and uniformly distributed HNTs, and the weakening effect due to the formation of HNT agglomerates/particles.
Significance
Uniform distribution of a small amount of well-separated silanized HNTs into Bis-GMA/TEGDMA dental resins/composites could result in substantial improvements on mechanical properties.
1
Introduction
Dental composites, consisting of polymeric resin matrices with mass fractions of ∼30% and inorganic fillers with mass fractions of ∼70%, were first developed approximately half a century ago; compared to dental amalgams, the composites possess better esthetic properties and fewer safety concerns, while have shown reasonably satisfactory clinic results. Hence, the dental composites have been widely adopted by the dental profession as restorative materials of choice.
Dental resins are typically cured/hardened by photo-initiated free radical polymerization. Camphorquinone (CQ) is a common visible-light initiator, and ethyl-4-( N,N ′-dimethylamino) benzoate (4EDMAB) is a common co-initiator/accelerator. The dental monomer of 2.2′-bis-[4-(methacryloxypropoxy)-phenyl]-propane (Bis-GMA) has been widely used as a base monomer since it was invented in the early 1960s . Bis-GMA is a very viscous liquid; to improve the handling quality, a diluent monomer of tri (ethylene glycol) dimethacrylate (TEGDMA) is usually added to thin the resins. In the Bis-GMA/TEGDMA dental resin systems, Bis-GMA functions to reduce the photopolymerization induced volumetric shrinkage and to enhance the resin reactivity, while TEGDMA provides for the increased vinyl double bond conversion .
Compared to dental amalgams, dental composites reinforced with inorganic fillers ( e.g. , silanized glass/ceramic particles) have relatively low strength and durability, which limited their applications . Presently, the commercially available dental composites have the values of flexural strength varying from 80 to 140 MPa, which can fulfill basic requirements of filling in small tooth-cavities but cannot be utilized for large stress-bearing restorations ( e.g. , crowns and bridges). Furthermore, the service time of dental composites is generally shorter than 5 years , while dental amalgams typically have the service time of 15 years or longer . Investigations on the composite failures have revealed that, ironically, inorganic filler particles that are added to the dental resins for fortifying the materials are actually responsible, at least in part, for their demise . Many inorganic filler particles are spherical or irregular in shape; during function, the masticatory stress is transmitted through boluses of foods onto surfaces of filler particles projecting from the occlusal surface. Since the filler particles are considerably harder than the resin matrices, much of the stress is transmitted through the particles into the resins. Wherever the submerged portions of particles are angular or irregular in shape, the stress concentration could become excessively high. Such a condition tends to generate small cracks around the particles, thereby weakening the composites locally.
The reinforcement of dental composites using micron-scaled fibers/whiskers with high mechanical strength has been demonstrated to result in dramatic improvements on the properties . For example, Xu and co-workers revealed that, two-fold increase in strength and toughness could be readily achieved through the impregnation of extremely strong ceramic fibers/whiskers into dental composites; additionally, it would also lead to improvements on polishability, water-absorption, and strength-durability . The bridging mechanism has been proposed to explain the reinforcement effect: if a micro-crack is initiated in a matrix under contact wear and/or other stresses, the fibrillar filler particles remain intact across the crack planes and support the applied load. Crack-opening is therefore resisted by the bridging fillers, and the matrix is reinforced consequently. The requirements for effective bridging fillers include high mechanical properties ( e.g. , strength and modulus) and large surface-to-volume ratios.
Unlike micron-scaled fibers/whiskers, halloysite (Al 2 Si 2 O 5 (OH) 4 ·2H 2 O) is composed of nano-scaled crystals (tubes); the halloysite nanotube (HNT) is a two-layered aluminosilicate with predominantly hollow/tubular structure . Since halloysite is a naturally occurring mineral that is easy to purify, HNTs are available in large quantity; and it is much more cost-effective than many other nano-scaled fillers (such as carbon nanotubes/nanofibers) . Furthermore, halloysite is safe, biocompatible, and easy to process . HNTs have also been studied for clinical relevance; for example, Hughes and King reported that the naturally occurring HNTs could be used to enhance the capture of flowing cells . The diameters of HNTs are typically in tens of nanometers, and the lengths are usually in the range from ∼200 nm to 1–2 μm . The outer surface of HNTs has chemical properties similar to SiO 2 , while the inner surface has chemical properties similar to Al 2 O 3 . Nano-scaled crystals of HNTs possess a high degree of structural perfection and the concomitant superior mechanical properties; for example, the Young’s modulus of HNTs is estimated in the range of 230–340 GPa, which is calculated via the same geometric optimization protocol as used for imogolite nanotubes . Additionally, unlike layered silicates (such as montmorillonite), which are difficult to completely exfoliate into nano-scaled silicate layers and to uniformly distribute in dental matrices, halloysite is relatively easy to separate into individual HNTs and to distribute uniformly in dental matrices. This is because the spacing among agglomerated HNTs in halloysite is much larger than that among silicate layers in montmorillonite; as a result, the interaction of HNTs is considerably weaker than that of silicate layers in montmorillonite. Hence, there is no need for chemical substitution of metal ions with alkyl ammonium ions (a widely adopted method for intercalation/exfoliation of montmorillonite for the preparation of nanocomposites); the HNTs in halloysite can be well-separated by simply dispersing the halloysite powder in polar solvents (such as acetone) followed by mechanical stirring . Furthermore, the interfacial bonding strength between the silanized HNT filler and the dental resin matrix can be reasonably high since there are abundant Si OH groups on the outer surface of HNTs; and these groups can readily react with silane coupling agents such as 3-methacryloxypropyltrimethoxy (MPTMS).
The aim of this study was to investigate the Bis-GMA/TEGDMA dental resins (without conventional glass filler) and the corresponding composites (with conventional glass filler) reinforced with varied mass fractions of HNTs. The hypothesis was that uniform distribution of well-separated silanized HNTs into Bis-GMA/TEGDMA dental resins/composites would result in substantial improvements on the mechanical properties. To test the hypothesis, photo-cured Bis-GMA/TEGDMA dental resins/composites containing varied mass fractions of silanized HNTs were systematically fabricated; three dispersion methods were studied to separate the silanized halloysite as individual HNTs and to uniformly distribute them into dental matrices. The photopolymerization induced volumetric shrinkage was measured by a mercury dilatometer. The flexural strength, elastic modulus, and work of fracture were acquired from the standard three-point flexural test (ASTM D 790). The degree of vinyl double bond conversion and the photopolymerization rate were studied in situ by real time near infrared spectroscopy (RT-NIR). Analysis of variance (ANOVA) was used for the statistical analysis of the acquired data. Morphological structures of HNTs and representative fracture surfaces of the reinforced dental resins/composites were examined by transmission electron microscope (TEM) and scanning electron microscope (SEM).
2
Materials and methods
2.1
Materials
Bis-GMA, TEGDMA, CQ, 4EDMAB, MPTMS, and acetone were purchased from the Sigma–Aldrich Co. (Milwaukee, WI) and used without further purification. Purified halloysite powder (1250 mesh) was provided by the Dalian Global Mineral Co. (Dalian, China). The conventional (dental) glass filler for this study was finely milled 7% (mass fraction) silanized barium borosilicate glass powder (V-117-2707) provided by the Esstech Co. (Essington, PA).
2.2
Fabrication
2.2.1
Silanization of HNTs
The as-received halloysite powder was first dispersed in acetone with a mass fraction of 5%. The suspension was then vigorously stirred for 4 h at 400 rpm using a Heidolph RZR 50 Heavy Duty Stirrer. This process would convert the halloysite powder into separated HNTs, but the separated HNTs (existing in suspension) could re-aggregate into agglomerates/particles, particularly if acetone was removed. Thereafter, MPTMS was added into the suspension with a mass fraction of 10% to halloysite; the system was then stirred mechanically for 4 h at 400 rpm followed by heated at 90 °C to remove the solvent of acetone.
2.2.2
Dispersion of silanized HNTs into dental matrix
The silanized HNTs (with mass fractions ranging from 0% to 5%) were added into a dental resin system, which consisted of 49.5% Bis-GMA, 49.5% TEGDMA (the mass ratio of Bis-GMA/TEGDMA was 1/1), 0.2% CQ, and 0.8% 4EDMAB. Three methods were studied to identify the optimal one for uniform dispersion of the silanized halloysite as highly separated HNTs into the dental matrix. The prepared systems of Bis-GMA/TEGDMA/HNT were further mixed with conventional dental glass fillers (with the combined mass fraction of glass filler and HNT being 70%) to prepare three dental pastes.
- •
Method A : The silanized HNTs were first dispersed in TEGDMA followed by being mechanically stirred for 2 h at 400 rpm. Bis-GMA, CQ, and 4EDMAB were then added; after that, the system was mechanically stirred for another 30 min at 400 rpm.
- •
Method B: The silanized HNTs were dispersed into the mixture of TEGDMA/acetone (mass ratio: 20/80) first followed by being mechanically stirred for 2 h at 400 rpm. Acetone was then removed by evaporation; thereafter, Bis-GMA, CQ, and 4EDMAB were added, and the system was then stirred for another 30 min at 400 rpm.
- •
Method C: The silanized HNTs were first dispersed into 80% (mass fraction) acetone diluted dental resin system (composition as described above) followed by being stirred for 2 h at 400 rpm. Thereafter, acetone was removed by evaporation, and the system was further mechanically stirred for another 30 min at 400 rpm.
2.3
Characterization and evaluation
2.3.1
Volumetric shrinkage
The photopolymerization induced volumetric shrinkage was measured by a mercury dilatometer made by the Paffenbarger Research Center of the American Dental Association Foundation (Gaithersburg, MD). The essential component of this dilatometer is a mercury-filled tube with one end clamped against a glass slide, in which dental pastes are polymerized upon initiation by using a visible light-curing unit. The volume change is monitored by a Teflon plunger, which is sitting on top of the mercury column and connecting to a linear variable differential transducer (LVDT). Since the volume of mercury is affected by temperature, a thermistor is applied to monitor the temperature variation. The output of thermistor and the read of LVDT are collected by a computer, and the volume changes of the mercury are corrected by using the least-squares algorithm in considering of temperature fluctuations. During the measurement, after the reading of corrected LVDT was stabilized, the curing light was turned on for 1.0 min followed by the post-curing time of 1.0 h; thereafter, the curing light was turned on once more for another 30 s. If there was no significant change of the corrected LVDT reading during this time, the photopolymerization was considered complete and the data were recorded for further analysis. Three replicates were measured for each composite mixture (dental paste).
2.3.2
Photopolymerization kinetics
Real time near infrared spectroscopy (RT-NIR) was employed to investigate the photopolymerization kinetics of Bis-GMA/TEGDMA dental resins containing varied mass fractions of the silanized HNTs. The photopolymerization rate and the degree of vinyl double bond conversion were measured. A Bruker Tensor-27 FT-IR spectrometer equipped with a liquid nitrogen-cooled mercury–cadmium–telluride (MCT) detector was used to carry out this study. During the RT-NIR measurements, the spectrometer was continuously purged with dry nitrogen gas, and the uncured resin was placed in a U-shaped Teflon mold with both sides covered by glass slides (note that glass is transparent in the NIR region between wavenumbers of 4000 and 6500 cm −1 ). The length and width of the mold were 4 mm, and the thickness was 2 mm. The glass slides were tightly attached to the mold using small metal clamps. A standard visible light curing unit (Maxima 480), purchased from the L. D. Caulk Company (Milford, DE), was placed directly above the sample holder. The absorption band of vinyl double bond (6100–6250 cm −1 ) were monitored in situ by RT-NIR at ambient condition for 5 min, with 4 scans/spectrum and 8 wavenumber resolution, using series run. In RT-NIR series run, infrared spectra were collected periodically (the time interval of 1 s was used in this study) during the photopolymerization of the specimens. The curing light was manually turned on at 8 s and turned off at 38 s.
2.3.3
Mechanical properties
A standard three-point flexural test (ASTM D 793) with a span of 20 mm was used to fracture the specimens at crosshead speed of 0.5 mm/min using a computer-controlled universal mechanical testing machine (QTEST™/10, MTS Systems Co., USA). Mechanical properties of both Bis-GMA/TEGDMA dental resins (without conventional glass filler) and the corresponding composites (with the glass fillers) containing varied mass fractions of the silanized HNTs were acquired. The dimensions of Teflon mold for making the three-point flexural testing specimens were 2 mm × 2 mm × 25 mm. The specimens were photo-cured for 2 min on each side, and then carefully removed from the molds; all four sides of each specimen were carefully hand-polished with 2400 and 4000 grit silicon carbide paper and water coolant in a longitudinal direction. Five replicates were prepared for each sample.
2.3.4
Morphology
A Zeiss Supra 40 VP field-emission scanning electron microscope (SEM) was employed to examine morphologies of HNTs and representative fracture surfaces of Bis-GMA/TEGDMA dental resins/composites containing varied mass fractions of HNTs. A Hitachi H-7000 FA transmission electron microscope (TEM) was used to examine the structure of HNTs. Prior to SEM examination, the specimens were sputter-coated with gold to avoid charge accumulation. Prior to TEM examination, the silanized halloysite was dispersed in acetone with a mass fraction of ∼1%, and the suspension was then mechanically stirred for 30 min at 400 rpm. Thereafter, the carbon-coated TEM grids were dipped into the suspension and quickly removed; the samples were then used for TEM examination after the acetone on the TEM grids evaporated.
2.3.5
Statistical analysis
The acquired data of volumetric shrinkage, flexural strength ( S F ), elastic modulus ( E Y ), and work of fracture (WOF) were analyzed with one-way analysis of variance (ANOVA) using SPSS software, version 20 (SPSS, Chicago, IL). Significant differences were detected using the Tukey-HSD (honestly significant difference) test. References to significant differences were based on a probability of p < 0.05 unless otherwise stated.
2
Materials and methods
2.1
Materials
Bis-GMA, TEGDMA, CQ, 4EDMAB, MPTMS, and acetone were purchased from the Sigma–Aldrich Co. (Milwaukee, WI) and used without further purification. Purified halloysite powder (1250 mesh) was provided by the Dalian Global Mineral Co. (Dalian, China). The conventional (dental) glass filler for this study was finely milled 7% (mass fraction) silanized barium borosilicate glass powder (V-117-2707) provided by the Esstech Co. (Essington, PA).
2.2
Fabrication
2.2.1
Silanization of HNTs
The as-received halloysite powder was first dispersed in acetone with a mass fraction of 5%. The suspension was then vigorously stirred for 4 h at 400 rpm using a Heidolph RZR 50 Heavy Duty Stirrer. This process would convert the halloysite powder into separated HNTs, but the separated HNTs (existing in suspension) could re-aggregate into agglomerates/particles, particularly if acetone was removed. Thereafter, MPTMS was added into the suspension with a mass fraction of 10% to halloysite; the system was then stirred mechanically for 4 h at 400 rpm followed by heated at 90 °C to remove the solvent of acetone.
2.2.2
Dispersion of silanized HNTs into dental matrix
The silanized HNTs (with mass fractions ranging from 0% to 5%) were added into a dental resin system, which consisted of 49.5% Bis-GMA, 49.5% TEGDMA (the mass ratio of Bis-GMA/TEGDMA was 1/1), 0.2% CQ, and 0.8% 4EDMAB. Three methods were studied to identify the optimal one for uniform dispersion of the silanized halloysite as highly separated HNTs into the dental matrix. The prepared systems of Bis-GMA/TEGDMA/HNT were further mixed with conventional dental glass fillers (with the combined mass fraction of glass filler and HNT being 70%) to prepare three dental pastes.
- •
Method A : The silanized HNTs were first dispersed in TEGDMA followed by being mechanically stirred for 2 h at 400 rpm. Bis-GMA, CQ, and 4EDMAB were then added; after that, the system was mechanically stirred for another 30 min at 400 rpm.
- •
Method B: The silanized HNTs were dispersed into the mixture of TEGDMA/acetone (mass ratio: 20/80) first followed by being mechanically stirred for 2 h at 400 rpm. Acetone was then removed by evaporation; thereafter, Bis-GMA, CQ, and 4EDMAB were added, and the system was then stirred for another 30 min at 400 rpm.
- •
Method C: The silanized HNTs were first dispersed into 80% (mass fraction) acetone diluted dental resin system (composition as described above) followed by being stirred for 2 h at 400 rpm. Thereafter, acetone was removed by evaporation, and the system was further mechanically stirred for another 30 min at 400 rpm.
2.3
Characterization and evaluation
2.3.1
Volumetric shrinkage
The photopolymerization induced volumetric shrinkage was measured by a mercury dilatometer made by the Paffenbarger Research Center of the American Dental Association Foundation (Gaithersburg, MD). The essential component of this dilatometer is a mercury-filled tube with one end clamped against a glass slide, in which dental pastes are polymerized upon initiation by using a visible light-curing unit. The volume change is monitored by a Teflon plunger, which is sitting on top of the mercury column and connecting to a linear variable differential transducer (LVDT). Since the volume of mercury is affected by temperature, a thermistor is applied to monitor the temperature variation. The output of thermistor and the read of LVDT are collected by a computer, and the volume changes of the mercury are corrected by using the least-squares algorithm in considering of temperature fluctuations. During the measurement, after the reading of corrected LVDT was stabilized, the curing light was turned on for 1.0 min followed by the post-curing time of 1.0 h; thereafter, the curing light was turned on once more for another 30 s. If there was no significant change of the corrected LVDT reading during this time, the photopolymerization was considered complete and the data were recorded for further analysis. Three replicates were measured for each composite mixture (dental paste).
2.3.2
Photopolymerization kinetics
Real time near infrared spectroscopy (RT-NIR) was employed to investigate the photopolymerization kinetics of Bis-GMA/TEGDMA dental resins containing varied mass fractions of the silanized HNTs. The photopolymerization rate and the degree of vinyl double bond conversion were measured. A Bruker Tensor-27 FT-IR spectrometer equipped with a liquid nitrogen-cooled mercury–cadmium–telluride (MCT) detector was used to carry out this study. During the RT-NIR measurements, the spectrometer was continuously purged with dry nitrogen gas, and the uncured resin was placed in a U-shaped Teflon mold with both sides covered by glass slides (note that glass is transparent in the NIR region between wavenumbers of 4000 and 6500 cm −1 ). The length and width of the mold were 4 mm, and the thickness was 2 mm. The glass slides were tightly attached to the mold using small metal clamps. A standard visible light curing unit (Maxima 480), purchased from the L. D. Caulk Company (Milford, DE), was placed directly above the sample holder. The absorption band of vinyl double bond (6100–6250 cm −1 ) were monitored in situ by RT-NIR at ambient condition for 5 min, with 4 scans/spectrum and 8 wavenumber resolution, using series run. In RT-NIR series run, infrared spectra were collected periodically (the time interval of 1 s was used in this study) during the photopolymerization of the specimens. The curing light was manually turned on at 8 s and turned off at 38 s.
2.3.3
Mechanical properties
A standard three-point flexural test (ASTM D 793) with a span of 20 mm was used to fracture the specimens at crosshead speed of 0.5 mm/min using a computer-controlled universal mechanical testing machine (QTEST™/10, MTS Systems Co., USA). Mechanical properties of both Bis-GMA/TEGDMA dental resins (without conventional glass filler) and the corresponding composites (with the glass fillers) containing varied mass fractions of the silanized HNTs were acquired. The dimensions of Teflon mold for making the three-point flexural testing specimens were 2 mm × 2 mm × 25 mm. The specimens were photo-cured for 2 min on each side, and then carefully removed from the molds; all four sides of each specimen were carefully hand-polished with 2400 and 4000 grit silicon carbide paper and water coolant in a longitudinal direction. Five replicates were prepared for each sample.
2.3.4
Morphology
A Zeiss Supra 40 VP field-emission scanning electron microscope (SEM) was employed to examine morphologies of HNTs and representative fracture surfaces of Bis-GMA/TEGDMA dental resins/composites containing varied mass fractions of HNTs. A Hitachi H-7000 FA transmission electron microscope (TEM) was used to examine the structure of HNTs. Prior to SEM examination, the specimens were sputter-coated with gold to avoid charge accumulation. Prior to TEM examination, the silanized halloysite was dispersed in acetone with a mass fraction of ∼1%, and the suspension was then mechanically stirred for 30 min at 400 rpm. Thereafter, the carbon-coated TEM grids were dipped into the suspension and quickly removed; the samples were then used for TEM examination after the acetone on the TEM grids evaporated.
2.3.5
Statistical analysis
The acquired data of volumetric shrinkage, flexural strength ( S F ), elastic modulus ( E Y ), and work of fracture (WOF) were analyzed with one-way analysis of variance (ANOVA) using SPSS software, version 20 (SPSS, Chicago, IL). Significant differences were detected using the Tukey-HSD (honestly significant difference) test. References to significant differences were based on a probability of p < 0.05 unless otherwise stated.
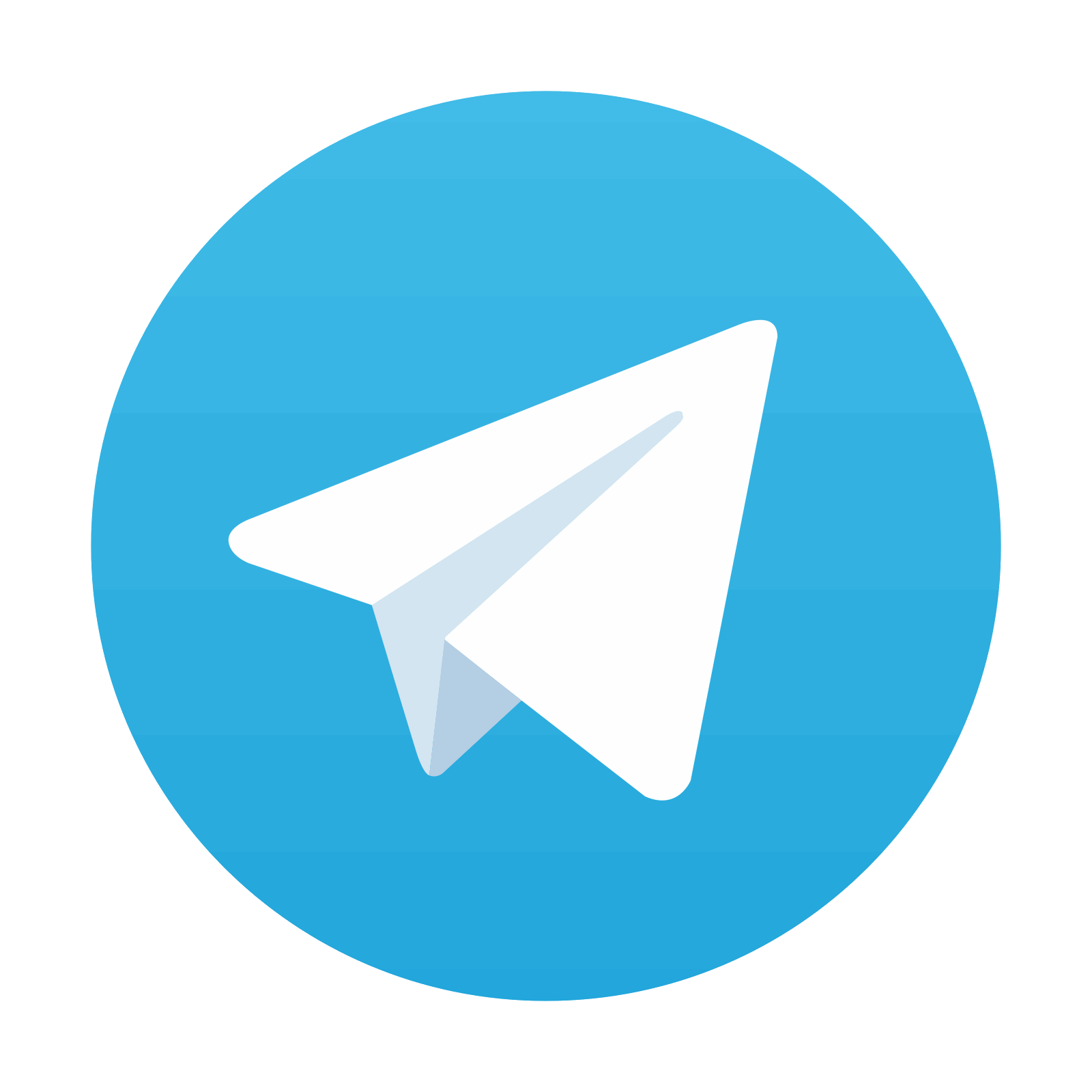
Stay updated, free dental videos. Join our Telegram channel

VIDEdental - Online dental courses
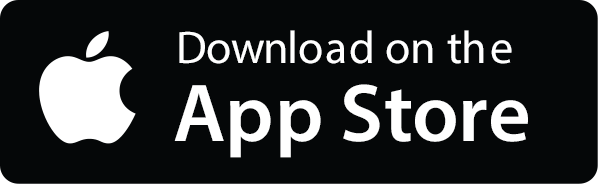
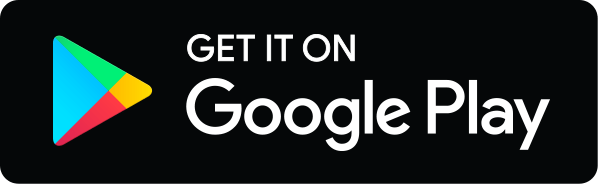